Welcome to the HPX documentation!¶
If you’re new to HPX you can get started with the Quick start guide. Don’t forget to read the Terminology section to learn about the most important concepts in HPX. The Examples give you a feel for how it is to write real HPX applications and the Manual contains detailed information about everything from building HPX to debugging it. There are links to blog posts and videos about HPX in Additional material.
If you can’t find what you’re looking for in the documentation, please:
- open an issue on GitHub;
- contact us on IRC, the HPX channel on the C++ Slack, or on our mailing list; or
- read or ask questions tagged with HPX on StackOverflow.
What is HPX?¶
HPX is a C++ Standard Library for Concurrency and Parallelism. It implements all of the corresponding facilities as defined by the C++ Standard. Additionally, in HPX we implement functionalities proposed as part of the ongoing C++ standardization process. We also extend the C++ Standard APIs to the distributed case. HPX is developed by the STE||AR group (see People).
The goal of HPX is to create a high quality, freely available, open source implementation of a new programming model for conventional systems, such as classic Linux based Beowulf clusters or multi-socket highly parallel SMP nodes. At the same time, we want to have a very modular and well designed runtime system architecture which would allow us to port our implementation onto new computer system architectures. We want to use real-world applications to drive the development of the runtime system, coining out required functionalities and converging onto a stable API which will provide a smooth migration path for developers.
The API exposed by HPX is not only modeled after the interfaces defined by the C++11/14/17/20 ISO standard. It also adheres to the programming guidelines used by the Boost collection of C++ libraries. We aim to improve the scalability of today’s applications and to expose new levels of parallelism which are necessary to take advantage of the exascale systems of the future.
What’s so special about HPX?¶
- HPX exposes a uniform, standards-oriented API for ease of programming parallel and distributed applications.
- It enables programmers to write fully asynchronous code using hundreds of millions of threads.
- HPX provides unified syntax and semantics for local and remote operations.
- HPX makes concurrency manageable with dataflow and future based synchronization.
- It implements a rich set of runtime services supporting a broad range of use cases.
- HPX exposes a uniform, flexible, and extendable performance counter framework which can enable runtime adaptivity
- It is designed to solve problems conventionally considered to be scaling-impaired.
- HPX has been designed and developed for systems of any scale, from hand-held devices to very large scale systems.
- It is the first fully functional implementation of the ParalleX execution model.
- HPX is published under a liberal open-source license and has an open, active, and thriving developer community.
Why HPX?¶
Current advances in high performance computing (HPC) continue to suffer from the issues plaguing parallel computation. These issues include, but are not limited to, ease of programming, inability to handle dynamically changing workloads, scalability, and efficient utilization of system resources. Emerging technological trends such as multi-core processors further highlight limitations of existing parallel computation models. To mitigate the aforementioned problems, it is necessary to rethink the approach to parallelization models. ParalleX contains mechanisms such as multi-threading, parcels, global name space support, percolation and local control objects (LCO). By design, ParalleX overcomes limitations of current models of parallelism by alleviating contention, latency, overhead and starvation. With ParalleX, it is further possible to increase performance by at least an order of magnitude on challenging parallel algorithms, e.g., dynamic directed graph algorithms and adaptive mesh refinement methods for astrophysics. An additional benefit of ParalleX is fine-grained control of power usage, enabling reductions in power consumption.
ParalleX—a new execution model for future architectures¶
ParalleX is a new parallel execution model that offers an alternative to the conventional computation models, such as message passing. ParalleX distinguishes itself by:
- Split-phase transaction model
- Message-driven
- Distributed shared memory (not cache coherent)
- Multi-threaded
- Futures synchronization
- Local Control Objects (LCOs)
- Synchronization for anonymous producer-consumer scenarios
- Percolation (pre-staging of task data)
The ParalleX model is intrinsically latency hiding, delivering an abundance of variable-grained parallelism within a hierarchical namespace environment. The goal of this innovative strategy is to enable future systems delivering very high efficiency, increased scalability and ease of programming. ParalleX can contribute to significant improvements in the design of all levels of computing systems and their usage from application algorithms and their programming languages to system architecture and hardware design together with their supporting compilers and operating system software.
What is HPX?¶
High Performance ParalleX (HPX) is the first runtime system implementation of the ParalleX execution model. The HPX runtime software package is a modular, feature-complete, and performance oriented representation of the ParalleX execution model targeted at conventional parallel computing architectures such as SMP nodes and commodity clusters. It is academically developed and freely available under an open source license. We provide HPX to the community for experimentation and application to achieve high efficiency and scalability for dynamic adaptive and irregular computational problems. HPX is a C++ library that supports a set of critical mechanisms for dynamic adaptive resource management and lightweight task scheduling within the context of a global address space. It is solidly based on many years of experience in writing highly parallel applications for HPC systems.
The two-decade success of the communicating sequential processes (CSP) execution model and its message passing interface (MPI) programming model has been seriously eroded by challenges of power, processor core complexity, multi-core sockets, and heterogeneous structures of GPUs. Both efficiency and scalability for some current (strong scaled) applications and future Exascale applications demand new techniques to expose new sources of algorithm parallelism and exploit unused resources through adaptive use of runtime information.
The ParalleX execution model replaces CSP to provide a new computing paradigm embodying the governing principles for organizing and conducting highly efficient scalable computations greatly exceeding the capabilities of today’s problems. HPX is the first practical, reliable, and performance-oriented runtime system incorporating the principal concepts of the ParalleX model publicly provided in open source release form.
HPX is designed by the STE||AR Group (Systems Technology, Emergent Parallelism, and Algorithm Research) at Louisiana State University (LSU)’s Center for Computation and Technology (CCT) to enable developers to exploit the full processing power of many-core systems with an unprecedented degree of parallelism. STE||AR is a research group focusing on system software solutions and scientific application development for hybrid and many-core hardware architectures.
What makes our systems slow?¶
Estimates say that we currently run our computers at way below 100% efficiency. The theoretical peak performance (usually measured in FLOPS—floating point operations per second) is much higher than any practical peak performance reached by any application. This is particularly true for highly parallel hardware. The more hardware parallelism we provide to an application, the better the application must scale in order to efficiently use all the resources of the machine. Roughly speaking, we distinguish two forms of scalability: strong scaling (see Amdahl’s Law) and weak scaling (see Gustafson’s Law). Strong scaling is defined as how the solution time varies with the number of processors for a fixed total problem size. It gives an estimate of how much faster can we solve a particular problem by throwing more resources at it. Weak scaling is defined as how the solution time varies with the number of processors for a fixed problem size per processor. In other words, it defines how much more data can we process by using more hardware resources.
In order to utilize as much hardware parallelism as possible an application must exhibit excellent strong and weak scaling characteristics, which requires a high percentage of work executed in parallel, i.e. using multiple threads of execution. Optimally, if you execute an application on a hardware resource with N processors it either runs N times faster or it can handle N times more data. Both cases imply 100% of the work is executed on all available processors in parallel. However, this is just a theoretical limit. Unfortunately, there are more things which limit scalability, mostly inherent to the hardware architectures and the programming models we use. We break these limitations into four fundamental factors which make our systems SLOW:
- Starvation occurs when there is insufficient concurrent work available to maintain high utilization of all resources.
- Latencies are imposed by the time-distance delay intrinsic to accessing remote resources and services.
- Overhead is work required for the management of parallel actions and resources on the critical execution path which is not necessary in a sequential variant.
- Waiting for contention resolution is the delay due to the lack of availability of oversubscribed shared resources.
Each of those four factors manifests itself in multiple and different ways; each of the hardware architectures and programming models expose specific forms. However the interesting part is that all of them are limiting the scalability of applications no matter what part of the hardware jungle we look at. Hand-helds, PCs, supercomputers, or the cloud, all suffer from the reign of the 4 horsemen: Starvation, Latency, Overhead, and Contention. This realization is very important as it allows us to derive the criteria for solutions to the scalability problem from first principles, it allows us to focus our analysis on very concrete patterns and measurable metrics. Moreover, any derived results will be applicable to a wide variety of targets.
Technology demands new response¶
Today’s computer systems are designed based on the initial ideas of John von Neumann, as published back in 1945, and later extended by the Harvard architecture. These ideas form the foundation, the execution model of computer systems we use currently. But apparently a new response is required in the light of the demands created by today’s technology.
So, what are the overarching objectives for designing systems allowing for applications to scale as they should? In our opinion, the main objectives are:
- Performance: as mentioned, scalability and efficiency are the main criteria people are interested in
- Fault tolerance: the low expected mean time between failures (MTBF) of future systems requires embracing faults, not trying to avoid them
- Power: minimizing energy consumption is a must as it is one of the major cost factors today, even more so in the future
- Generality: any system should be usable for a broad set of use cases
- Programmability: for me as a programmer this is a very important objective, ensuring long term platform stability and portability
What needs to be done to meet those objectives, to make applications scale better on tomorrow’s architectures? Well, the answer is almost obvious: we need to devise a new execution model—a set of governing principles for the holistic design of future systems—targeted at minimizing the effect of the outlined SLOW factors. Everything we create for future systems, every design decision we make, every criteria we apply, has to be validated against this single, uniform metric. This includes changes in the hardware architecture we prevalently use today, and it certainly involves new ways of writing software, starting from the operating system, runtime system, compilers, and at the application level. However the key point is that all those layers have to be co-designed, they are interdependent and cannot be seen as separate facets. The systems we have today have been evolving for over 50 years now. All layers function in a certain way relying on the other layers to do so as well. However, we do not have the time to wait for a coherent system to evolve for another 50 years. The new paradigms are needed now—therefore, co-design is the key.
Governing principles applied while developing HPX¶
As it turn out, we do not have to start from scratch. Not everything has to be invented and designed anew. Many of the ideas needed to combat the 4 horsemen have already been had, often more than 30 years ago. All it takes is to gather them into a coherent approach. We’ll highlight some of the derived principles we think to be crucial for defeating SLOW. Some of those are focused on high-performance computing, others are more general.
Focus on latency hiding instead of latency avoidance¶
It is impossible to design a system exposing zero latencies. In an effort to come as close as possible to this goal many optimizations are mainly targeted towards minimizing latencies. Examples for this can be seen everywhere, for instance low latency network technologies like InfiniBand, caching memory hierarchies in all modern processors, the constant optimization of existing MPI implementations to reduce related latencies, or the data transfer latencies intrinsic to the way we use GPGPUs today. It is important to note, that existing latencies are often tightly related to some resource having to wait for the operation to be completed. At the same time it would be perfectly fine to do some other, unrelated work in the meantime, allowing the system to hide the latencies by filling the idle-time with useful work. Modern systems already employ similar techniques (pipelined instruction execution in the processor cores, asynchronous input/output operations, and many more). What we propose is to go beyond anything we know today and to make latency hiding an intrinsic concept of the operation of the whole system stack.
Embrace fine-grained parallelism instead of heavyweight Threads¶
If we plan to hide latencies even for very short operations, such as fetching the contents of a memory cell from main memory (if it is not already cached), we need to have very lightweight threads with extremely short context switching times, optimally executable within one cycle. Granted, for mainstream architectures this is not possible today (even if we already have special machines supporting this mode of operation, such as the Cray XMT). For conventional systems however, the smaller the overhead of a context switch and the finer the granularity of the threading system, the better will be the overall system utilization and its efficiency. For today’s architectures we already see a flurry of libraries providing exactly this type of functionality: non-pre-emptive, task-queue based parallelization solutions, such as Intel Threading Building Blocks (TBB), Microsoft Parallel Patterns Library (PPL), Cilk++, and many others. The possibility to suspend a current task if some preconditions for its execution are not met (such as waiting for I/O or the result of a different task), seamlessly switching to any other task which can continue, and to reschedule the initial task after the required result has been calculated, which makes the implementation of latency hiding almost trivial.
Rediscover constraint-based synchronization to replace global Barriers¶
The code we write today is riddled with implicit (and explicit) global barriers. By global barrier we mean the synchronization of the control flow between several (very often all) threads (when using OpenMP) or processes (MPI). For instance, an implicit global barrier is inserted after each loop parallelized using OpenMP as the system synchronizes the threads used to execute the different iterations in parallel. In MPI each of the communication steps imposes an explicit barrier onto the execution flow as (often all) nodes have to be synchronized. Each of those barriers acts as an eye of the needle the overall execution is forced to be squeezed through. Even minimal fluctuations in the execution times of the parallel threads (jobs) causes them to wait. Additionally it is often only one of the threads executing doing the actual reduce operation, which further impedes parallelism. A closer analysis of a couple of key algorithms used in science applications reveals that these global barriers are not always necessary. In many cases it is sufficient to synchronize a small subset of the threads. Any operation should proceed whenever the preconditions for its execution are met, and only those. Usually there is no need to wait for iterations of a loop to finish before you could continue calculating other things, all you need is to have those iterations done which were producing the required results for a particular next operation. Good bye global barriers, hello constraint based synchronization! People have been trying to build this type of computing (and even computers) already back in the 1970’s. The theory behind what they did is based on ideas around static and dynamic dataflow. There are certain attempts today to get back to those ideas and to incorporate them with modern architectures. For instance, a lot of work is being done in the area of constructing dataflow oriented execution trees. Our results show that employing dataflow techniques in combination with the other ideas, as outlined herein, considerably improves scalability for many problems.
Adaptive Locality Control instead of Static Data Distribution¶
While this principle seems to be a given for single desktop or laptop computers (the operating system is your friend), it is everything but ubiquitous on modern supercomputers, which are usually built from a large number of separate nodes (i.e. Beowulf clusters), tightly interconnected by a high bandwidth, low latency network. Today’s prevalent programming model for those is MPI which does not directly help with proper data distribution, leaving it to the programmer to decompose the data to all of the nodes the application is running on. There are a couple of specialized languages and programming environments based on PGAS (Partitioned Global Address Space) designed to overcome this limitation, such as Chapel, X10, UPC, or Fortress. However all systems based on PGAS rely on static data distribution. This works fine as long as such a static data distribution does not result in homogeneous workload distributions or other resource utilization imbalances. In a distributed system these imbalances can be mitigated by migrating part of the application data to different localities (nodes). The only framework supporting (limited) migration today is Charm++. The first attempts towards solving related problem go back decades as well, a good example is the Linda coordination language. Nevertheless, none of the other mentioned systems support data migration today, which forces the users to either rely on static data distribution and live with the related performance hits or to implement everything themselves, which is very tedious and difficult. We believe that the only viable way to flexibly support dynamic and adaptive locality control is to provide a global, uniform address space to the applications, even on distributed systems.
Prefer moving work to the data over moving data to the work¶
For best performance it seems obvious to minimize the amount of bytes transferred from one part of the system to another. This is true on all levels. At the lowest level we try to take advantage of processor memory caches, thus minimizing memory latencies. Similarly, we try to amortize the data transfer time to and from GPGPUs as much as possible. At high levels we try to minimize data transfer between different nodes of a cluster or between different virtual machines on the cloud. Our experience (well, it’s almost common wisdom) show that the amount of bytes necessary to encode a certain operation is very often much smaller than the amount of bytes encoding the data the operation is performed upon. Nevertheless we still often transfer the data to a particular place where we execute the operation just to bring the data back to where it came from afterwards. As an example let me look at the way we usually write our applications for clusters using MPI. This programming model is all about data transfer between nodes. MPI is the prevalent programming model for clusters, it is fairly straightforward to understand and to use. Therefore, we often write the applications in a way accommodating this model, centered around data transfer. These applications usually work well for smaller problem sizes and for regular data structures. The larger the amount of data we have to churn and the more irregular the problem domain becomes, the worse are the overall machine utilization and the (strong) scaling characteristics. While it is not impossible to implement more dynamic, data driven, and asynchronous applications using MPI, it is overly difficult to so. At the same time, if we look at applications preferring to execute the code close the locality where the data was placed, i.e. utilizing active messages (for instance based on Charm++), we see better asynchrony, simpler application codes, and improved scaling.
Favor message driven computation over message passing¶
Today’s prevalently used programming model on parallel (multi-node) systems is MPI. It is based on message passing (as the name implies), which means that the receiver has to be aware of a message about to come in. Both codes, the sender and the receiver, have to synchronize in order to perform the communication step. Even the newer, asynchronous interfaces require explicitly coding the algorithms around the required communication scheme. As a result, any more than trivial MPI application spends a considerable amount of time waiting for incoming messages, thus causing starvation and latencies to impede full resource utilization. The more complex and more dynamic the data structures and algorithms become, the larger are the adverse effects. The community has discovered message-driven and (data-driven) methods of implementing algorithms a long time ago, and systems such as Charm++ already have integrated active messages demonstrating the validity of the concept. Message driven computation allows sending messages without requiring the receiver to actively wait for them. Any incoming message is handled asynchronously and triggers the encoded action by passing along arguments and—possibly—continuations. HPX combines this scheme with work queue-based scheduling as described above, which allows the system to overlap almost completely any communication with useful work, thereby minimizing latencies.
Quick start¶
This section is intended to get you to the point of running a basic HPX program as quickly as possible. To that end we skip many details but instead give you hints and links to more details along the way.
We assume that you are on a Unix system with access to reasonably recent
packages. You should have cmake
and make
available for the build system
(pkg-config
is also supported, see Using HPX with pkg-config).
Getting HPX¶
Download a tarball of the latest release from HPX Downloads and
unpack it or clone the repository directly using git
:
git clone https://github.com/STEllAR-GROUP/hpx.git
It is also recommended that you check out the latest stable tag:
git checkout 1.3.0
HPX dependencies¶
The minimum dependencies needed to use HPX are Boost and Portable Hardware Locality (HWLOC). If these
are not available through your system package manager, see
Installing Boost and Installing Hwloc for instructions on how
to build them yourself. In addition to Boost and Portable Hardware Locality (HWLOC), it is recommended
that you don’t use the system allocator, but instead use either tcmalloc
from google-perftools (default) or jemalloc for better performance. If you
would like to try HPX without a custom allocator at this point you can
configure HPX to use the system allocator in the next step.
A full list of required and optional dependencies, including recommended versions is available at Prerequisites.
Building HPX¶
Once you have the source code and the dependencies, set up a separate build directory and configure the project. Assuming all your dependencies are in paths known to CMake, the following gets you started:
# In the HPX source directory
mkdir build && cd build
cmake -DCMAKE_INSTALL_PREFIX=/install/path ..
make install
This will build the core HPX libraries and examples, and install them to your
chosen location. If you want to install HPX to system folders simply leave out
the CMAKE_INSTALL_PREFIX
option. This may take a while. To speed up the
process launch more jobs by passing the -jN
option to make
.
Tip
Do not set only -j
(i.e. -j
without an explicit number of jobs)
unless you have a lot of memory available on your machine.
Tip
If you want to change CMake variables for your build it is usually a good
idea to start with a clean build directory to avoid configuration problems.
It is especially important that you use a clean build directory when changing
between Release
and Debug
modes.
If your dependencies are in custom locations you may need to tell CMake where to find them by passing one or more of the following options to CMake:
-DBOOST_ROOT=/path/to/boost
-DHWLOC_ROOT=/path/to/hwloc
-DTCMALLOC_ROOT=/path/to/tcmalloc
-DJEMALLOC_ROOT=/path/to/jemalloc
If you want to try HPX without using a custom allocator pass
-DHPX_WITH_MALLOC=system
to CMake.
Important
If you are building HPX for a system with more than 64 processing units you
must change the CMake variables HPX_WITH_MORE_THAN_64_THREADS
(to
On
) and HPX_WITH_MAX_CPU_COUNT
(to a value at least as big as the
number of (virtual) cores on your system).
To build the tests run make tests
. To run the tests run either make test
or use ctest
for more control over which tests to run. You can run single
tests for example with ctest --output-on-failure -R
tests.unit.parallel.algorithms.for_loop
or a whole group of tests with ctest
--output-on-failure -R tests.unit
.
If you did not run make install
earlier do so now or build the
hello_world_1
example by running:
make hello_world_1
HPX executables end up in the bin
directory in your build directory. You
can now run hello_world_1
and should see the following output:
./bin/hello_world_1
Hello World!
You’ve just run an example which prints Hello World!
from the HPX runtime.
The source for the example is in examples/quickstart/hello_world_1.cpp
. The
hello_world_distributed
example (also available in the
examples/quickstart
directory) is a distributed hello world program which is
described in Remote execution with actions: Hello world. It provides a gentle introduction to
the distributed aspects of HPX.
Tip
Most build targets in HPX have two names: a simple name and
a hierarchical name corresponding to what type of example or
test the target is. If you are developing HPX it is often helpful to run
make help
to get a list of available targets. For example, make help |
grep hello_world
outputs the following:
... examples.quickstart.hello_world_2
... hello_world_2
... examples.quickstart.hello_world_1
... hello_world_1
... examples.quickstart.hello_world_distributed
... hello_world_distributed
It is also possible to build e.g. all quickstart examples using make
examples.quickstart
.
Hello, World!¶
The following CMakeLists.txt
is a minimal example of what you need in order to
build an executable using CMake and HPX:
cmake_minimum_required(VERSION 3.3.2)
project(my_hpx_project CXX)
find_package(HPX REQUIRED)
add_hpx_executable(my_hpx_program
SOURCES main.cpp
COMPONENT_DEPENDENCIES iostreams)
Note
You will most likely have more than one main.cpp
file in your project.
See the section on Using HPX with CMake-based projects for more details on how to use
add_hpx_executable
.
Note
COMPONENT_DEPENDENCIES iostreams
is optional for a minimal project but
lets us use the HPX equivalent of std::cout
, i.e. the HPX
The HPX I/O-streams component functionality in our application.
Create a new project directory and a CMakeLists.txt
with the contents above.
Also create a main.cpp
with the contents below.
// Including 'hpx/hpx_main.hpp' instead of the usual 'hpx/hpx_init.hpp' enables
// to use the plain C-main below as the direct main HPX entry point.
#include <hpx/hpx_main.hpp>
#include <hpx/include/iostreams.hpp>
int main()
{
// Say hello to the world!
hpx::cout << "Hello World!\n" << hpx::flush;
return 0;
}
Then, in your project directory run the following:
mkdir build && cd build
cmake -DCMAKE_PREFIX_PATH=/path/to/hpx/installation ..
make all
./my_hpx_program
The program looks almost like a regular C++ hello world with the exception of
the two includes and hpx::cout
. When you include hpx_main.hpp
some
things will be done behind the scenes to make sure that main
actually gets
launched on the HPX runtime. So while it looks almost the same you can now use
futures, async
, parallel algorithms and more which make use of the HPX
runtime with lightweight threads. hpx::cout
is a replacement for
std::cout
to make sure printing never blocks a lightweight thread. You can
read more about hpx::cout
in The HPX I/O-streams component. If you rebuild and run your
program now you should see the familiar Hello World!
:
./my_hpx_program
Hello World!
Note
You do not have to let HPX take over your main function like in the
example. You can instead keep your normal main function, and define a
separate hpx_main
function which acts as the entry point to the HPX
runtime. In that case you start the HPX runtime explicitly by calling
hpx::init
:
// Copyright (c) 2007-2012 Hartmut Kaiser
//
// Distributed under the Boost Software License, Version 1.0. (See accompanying
// file LICENSE_1_0.txt or copy at http://www.boost.org/LICENSE_1_0.txt)
///////////////////////////////////////////////////////////////////////////////
// The purpose of this example is to initialize the HPX runtime explicitly and
// execute a HPX-thread printing "Hello World!" once. That's all.
//[hello_world_2_getting_started
#include <hpx/hpx_init.hpp>
#include <hpx/include/iostreams.hpp>
int hpx_main(int, char**)
{
// Say hello to the world!
hpx::cout << "Hello World!\n" << hpx::flush;
return hpx::finalize();
}
int main(int argc, char* argv[])
{
return hpx::init(argc, argv);
}
//]
You can also use hpx::start
and hpx::stop
for a
non-blocking alternative, or use hpx::resume
and
hpx::suspend
if you need to combine HPX with other runtimes.
See Starting the HPX runtime for more details on how to initialize and run the HPX runtime.
Caution
When including hpx_main.hpp
the user-defined main
gets renamed and
the real main
function is defined by HPX. This means that the
user-defined main
must include a return statement, unlike the real
main
. If you do not include the return statement you may end up with
confusing compile time errors mentioning user_main
or even runtime
errors.
Writing task-based applications¶
So far we haven’t done anything that can’t be done using the C++ standard library. In this section we will give a short overview of what you can do with HPX on a single node. The essence is to avoid global synchronization and break up your application into small, composable tasks whose dependencies control the flow of your application. Remember, however, that HPX allows you to write distributed applications similarly to how you would write applications for a single node (see Why HPX? and Writing distributed HPX applications).
If you are already familiar with async
and future
s from the C++ standard
library, the same functionality is available in HPX.
The following terminology is essential when talking about task-based C++ programs:
- lightweight thread: Essential for good performance with task-based programs. Lightweight refers to smaller stacks and faster context switching compared to OS-threads. Smaller overheads allow the program to be broken up into smaller tasks, which in turns helps the runtime fully utilize all processing units.
async
: The most basic way of launching tasks asynchronously. Returns afuture<T>
.future<T>
: Represents a value of typeT
that will be ready in the future. The value can be retrieved withget
(blocking) and one can check if the value is ready withis_ready
(non-blocking).shared_future<T>
: Same asfuture<T>
but can be copied (similar tostd::unique_ptr
vsstd::shared_ptr
).- continuation: A function that is to be run after a previous task has run
(represented by a future).
then
is a method offuture<T>
that takes a function to run next. Used to build up dataflow DAGs (directed acyclic graphs).shared_future
s help you split up nodes in the DAG and functions likewhen_all
help you join nodes in the DAG.
The following example is a collection of the most commonly used functionality in HPX:
#include <hpx/hpx_main.hpp>
#include <hpx/include/iostreams.hpp>
#include <hpx/include/lcos.hpp>
#include <hpx/include/parallel_generate.hpp>
#include <hpx/include/parallel_sort.hpp>
#include <random>
#include <vector>
void final_task(hpx::future<hpx::util::tuple<hpx::future<double>, hpx::future<void>>>)
{
hpx::cout << "in final_task" << hpx::endl;
}
// Avoid ABI incompatibilities between C++11/C++17 as std::rand has exception
// specification in libstdc++.
int rand_wrapper()
{
return std::rand();
}
int main(int, char**)
{
// A function can be launched asynchronously. The program will not block
// here until the result is available.
hpx::future<int> f = hpx::async([]() { return 42; });
hpx::cout << "Just launched a task!" << hpx::endl;
// Use get to retrieve the value from the future. This will block this task
// until the future is ready, but the HPX runtime will schedule other tasks
// if there are tasks available.
hpx::cout << "f contains " << f.get() << hpx::endl;
// Let's launch another task.
hpx::future<double> g = hpx::async([]() { return 3.14; });
// Tasks can be chained using the then method. The continuation takes the
// future as an argument.
hpx::future<double> result = g.then([](hpx::future<double>&& gg)
{
// This function will be called once g is ready. gg is g moved
// into the continuation.
return gg.get() * 42.0 * 42.0;
});
// You can check if a future is ready with the is_ready method.
hpx::cout << "Result is ready? " << result.is_ready() << hpx::endl;
// You can launch other work in the meantime. Let's sort a vector.
std::vector<int> v(1000000);
// We fill the vector synchronously and sequentially.
hpx::parallel::generate(hpx::parallel::execution::seq,
std::begin(v), std::end(v), &rand_wrapper);
// We can launch the sort in parallel and asynchronously.
hpx::future<void> done_sorting =
hpx::parallel::sort(
hpx::parallel::execution::par( // In parallel.
hpx::parallel::execution::task), // Asynchronously.
std::begin(v),
std::end(v));
// We launch the final task when the vector has been sorted and result is
// ready using when_all.
auto all = hpx::when_all(result, done_sorting).then(&final_task);
// We can wait for all to be ready.
all.wait();
// all must be ready at this point because we waited for it to be ready.
hpx::cout <<
(all.is_ready() ? "all is ready!" : "all is not ready...") << hpx::endl;
return hpx::finalize();
}
Try copying the contents to your main.cpp
file and look at the output. It can
be a good idea to go through the program step by step with a debugger. You can
also try changing the types or adding new arguments to functions to make sure
you can get the types to match. The type of the then
method can be especially
tricky to get right (the continuation needs to take the future as an argument).
Note
HPX programs accept command line arguments. The most important one is
--hpx:threads
=N
to set the number of OS-threads used by
HPX. HPX uses one thread per core by default. Play around with the
example above and see what difference the number of threads makes on the
sort
function. See Launching and configuring HPX applications for more details on
how and what options you can pass to HPX.
Tip
The example above used the construction hpx::when_all(...).then(...)
. For
convenience and performance it is a good idea to replace uses of
hpx::when_all(...).then(...)
with dataflow
. See
Dataflow: Interest calculator for more details on dataflow
.
Tip
If possible, prefer to use the provided parallel algorithms instead of writing your own implementation. This can save you time and the resulting program is often faster.
Next steps¶
If you haven’t done so already, reading the Terminology section will help you get familiar with the terms used in HPX.
The Examples section contains small, self-contained walkthroughs of example HPX programs. The Local to remote: 1D stencil example is a thorough, realistic example starting from a single node implementation and going stepwise to a distributed implementation.
The Manual contains detailed information on writing, building and running HPX applications.
Terminology¶
This section gives definitions for some of the terms used throughout the HPX documentation and source code.
- Locality
- A locality in HPX describes a synchronous domain of execution, or the domain of bounded upper response time. This normally is just a single node in a cluster or a NUMA domain in a SMP machine.
- Active Global Address Space
- AGAS
- HPX incorporates a global address space. Any executing thread can access any object within the domain of the parallel application with the caveat that it must have appropriate access privileges. The model does not assume that global addresses are cache coherent; all loads and stores will deal directly with the site of the target object. All global addresses within a Synchronous Domain are assumed to be cache coherent for those processor cores that incorporate transparent caches. The Active Global Address Space used by HPX differs from research PGAS models. Partitioned Global Address Space is passive in their means of address translation. Copy semantics, distributed compound operations, and affinity relationships are some of the global functionality supported by AGAS.
- Process
- The concept of the “process” in HPX is extended beyond that of either sequential execution or communicating sequential processes. While the notion of process suggests action (as do “function” or “subroutine”) it has a further responsibility of context, that is, the logical container of program state. It is this aspect of operation that process is employed in HPX. Furthermore, referring to “parallel processes” in HPX designates the presence of parallelism within the context of a given process, as well as the coarse grained parallelism achieved through concurrency of multiple processes of an executing user job. HPX processes provide a hierarchical name space within the framework of the active global address space and support multiple means of internal state access from external sources.
- Parcel
- The Parcel is a component in HPX that communicates data, invokes an action at a distance, and distributes flow-control through the migration of continuations. Parcels bridge the gap of asynchrony between synchronous domains while maintaining symmetry of semantics between local and global execution. Parcels enable message-driven computation and may be seen as a form of “active messages”. Other important forms of message-driven computation predating active messages include dataflow tokens, the J-machine’s support for remote method instantiation, and at the coarse grained variations of Unix remote procedure calls, among others. This enables work to be moved to the data as well as performing the more common action of bringing data to the work. A parcel can cause actions to occur remotely and asynchronously, among which are the creation of threads at different system nodes or synchronous domains.
- Local Control Object
- Lightweight Control Object
- LCO
- Lightweight Control Object
A local control object (sometimes called a lightweight control object) is a general term for the synchronization mechanisms used in HPX. Any object implementing a certain concept can be seen as an LCO. This concepts encapsulates the ability to be triggered by one or more events which when taking the object into a predefined state will cause a thread to be executed. This could either create a new thread or resume an existing thread.
The LCO is a family of synchronization functions potentially representing many classes of synchronization constructs, each with many possible variations and multiple instances. The LCO is sufficiently general that it can subsume the functionality of conventional synchronization primitives such as spinlocks, mutexes, semaphores, and global barriers. However due to the rich concept an LCO can represent powerful synchronization and control functionality not widely employed, such as dataflow and futures (among others), which open up enormous opportunities for rich diversity of distributed control and operation.
See Using LCOs for more details on how to use LCOs in HPX.
- Action
- An action is a function that can be invoked remotely. In HPX a plain function can be made into an action using a macro. See Applying actions for details on how to use actions in HPX.
- Component
- A component is a C++ object which can be accessed remotely. A component can also contain member functions which can be invoked remotely. These are referred to as component actions. See Writing components for details on how to use components in HPX.
Examples¶
The following sections analyze some examples to help you get familiar with the HPX style of programming. We start off with simple examples that utilize basic HPX elements and then begin to expose the reader to the more complex and powerful HPX concepts.
Asynchronous execution with hpx::async
: Fibonacci¶
The Fibonacci sequence is a sequence of numbers starting with 0 and 1 where every subsequent number is the sum of the previous two numbers. In this example, we will use HPX to calculate the value of the n-th element of the Fibonacci sequence. In order to compute this problem in parallel, we will use a facility known as a future.
As shown in the Fig. 1 below, a future encapsulates a delayed computation. It acts as a proxy for a result initially not known, most of the time because the computation of the result has not completed yet. The future synchronizes the access of this value by optionally suspending any HPX-threads requesting the result until the value is available. When a future is created, it spawns a new HPX-thread (either remotely with a parcel or locally by placing it into the thread queue) which, when run, will execute the function associated with the future. The arguments of the function are bound when the future is created.
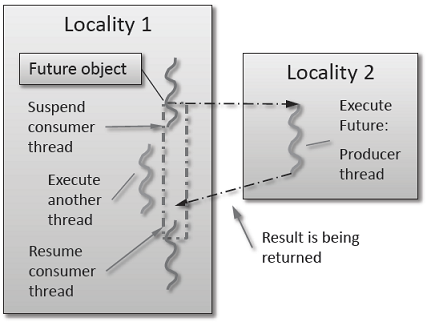
Fig. 1 Schematic of a future execution.
Once the function has finished executing, a write operation is performed on the future. The write operation marks the future as completed, and optionally stores data returned by the function. When the result of the delayed computation is needed, a read operation is performed on the future. If the future’s function hasn’t completed when a read operation is performed on it, the reader HPX-thread is suspended until the future is ready. The future facility allows HPX to schedule work early in a program so that when the function value is needed it will already be calculated and available. We use this property in our Fibonacci example below to enable its parallel execution.
Setup¶
The source code for this example can be found here:
fibonacci_local.cpp
.
To compile this program, go to your HPX build directory (see HPX build system for information on configuring and building HPX) and enter:
make examples.quickstart.fibonacci_local
To run the program type:
./bin/fibonacci_local
This should print (time should be approximate):
fibonacci(10) == 55
elapsed time: 0.002430 [s]
This run used the default settings, which calculate the tenth element of the
Fibonacci sequence. To declare which Fibonacci value you want to calculate, use
the --n-value
option. Additionally you can use the --hpx:threads
option to declare how many OS-threads you wish to use when running the program.
For instance, running:
./bin/fibonacci --n-value 20 --hpx:threads 4
Will yield:
fibonacci(20) == 6765
elapsed time: 0.062854 [s]
Walkthrough¶
Now that you have compiled and run the code, let’s look at how the code works.
Since this code is written in C++, we will begin with the main()
function.
Here you can see that in HPX, main()
is only used to initialize the
runtime system. It is important to note that application-specific command line
options are defined here. HPX uses Boost.Program Options for command line
processing. You can see that our programs --n-value
option is set by calling
the add_options()
method on an instance of
boost::program_options::options_description
. The default value of the
variable is set to 10. This is why when we ran the program for the first time
without using the --n-value
option the program returned the 10th value of
the Fibonacci sequence. The constructor argument of the description is the text
that appears when a user uses the --hpx:help
option to see what
command line options are available. HPX_APPLICATION_STRING
is a macro that
expands to a string constant containing the name of the HPX application
currently being compiled.
In HPX main()
is used to initialize the runtime system and pass the
command line arguments to the program. If you wish to add command line options
to your program you would add them here using the instance of the Boost class
options_description
, and invoking the public member function
.add_options()
(see Boost Documentation for more details). hpx::init
calls hpx_main()
after setting up HPX, which is where the logic of our
program is encoded.
int main(int argc, char* argv[])
{
// Configure application-specific options
boost::program_options::options_description
desc_commandline("Usage: " HPX_APPLICATION_STRING " [options]");
desc_commandline.add_options()
( "n-value",
boost::program_options::value<std::uint64_t>()->default_value(10),
"n value for the Fibonacci function")
;
// Initialize and run HPX
return hpx::init(desc_commandline, argc, argv);
}
The hpx::init
function in main()
starts the runtime system, and
invokes hpx_main()
as the first HPX-thread. Below we can see that the
basic program is simple. The command line option --n-value
is read in, a
timer (hpx::util::high_resolution_timer
) is set up to record the
time it takes to do the computation, the fibonacci
function is invoked
synchronously, and the answer is printed out.
int hpx_main(boost::program_options::variables_map& vm)
{
// extract command line argument, i.e. fib(N)
std::uint64_t n = vm["n-value"].as<std::uint64_t>();
{
// Keep track of the time required to execute.
hpx::util::high_resolution_timer t;
std::uint64_t r = fibonacci(n);
char const* fmt = "fibonacci({1}) == {2}\nelapsed time: {3} [s]\n";
hpx::util::format_to(std::cout, fmt, n, r, t.elapsed());
}
return hpx::finalize(); // Handles HPX shutdown
}
The fibonacci
function itself is synchronous as the work done inside is
asynchronous. To understand what is happening we have to look inside the
fibonacci
function:
std::uint64_t fibonacci(std::uint64_t n)
{
if (n < 2)
return n;
// Invoking the Fibonacci algorithm twice is inefficient.
// However, we intentionally demonstrate it this way to create some
// heavy workload.
hpx::future<std::uint64_t> n1 = hpx::async(fibonacci, n - 1);
hpx::future<std::uint64_t> n2 = hpx::async(fibonacci, n - 2);
return n1.get() + n2.get(); // wait for the Futures to return their values
}
This block of code is looks similar to regular C++ code. First, if (n < 2)
,
meaning n is 0 or 1, then we return 0 or 1 (recall the first element of the
Fibonacci sequence is 0 and the second is 1). If n is larger than 1 we spawn two
new tasks whose results are contained in n1
and n2
. This is done using
hpx::async
which takes as arguments a function (function pointer,
object or lambda) and the arguments to the function. Instead of returning a
std::uint64_t
like fibonacci
does, hpx::async
returns a future of a
std::uint64_t
, i.e. hpx::future<std::uint64_t>
. Each of these futures
represents an asynchronous, recursive call to fibonacci
. After we’ve created
the futures, we wait for both of them to finish computing, we add them together,
and return that value as our result. We get the values from the futures using
the get
method. The recursive call tree will continue until n is equal to 0
or 1, at which point the value can be returned because it is implicitly known.
When this termination condition is reached, the futures can then be added up,
producing the n-th value of the Fibonacci sequence.
Note that calling get
potentially blocks the calling HPX-thread, and lets
other HPX-threads run in the meantime. There are, however, more efficient ways
of doing this. examples/quickstart/fibonacci_futures.cpp
contains many more
variations of locally computing the Fibonacci numbers, where each method makes
different tradeoffs in where asynchrony and parallelism is applied. To get
started, however, the method above is sufficient and optimizations can be
applied once you are more familiar with HPX. The example
Dataflow: Interest calculator presents dataflow, which is a way to more
efficiently chain together multiple tasks.
Asynchronous execution with hpx::async
and actions: Fibonacci¶
This example extends the previous example by
introducing actions: functions that can be run remotely. In this
example, however, we will still only run the action locally. The mechanism to
execute actions stays the same: hpx::async
. Later
examples will demonstrate running actions on remote localities
(e.g. Remote execution with actions: Hello world).
Setup¶
The source code for this example can be found here:
fibonacci.cpp
.
To compile this program, go to your HPX build directory (see HPX build system for information on configuring and building HPX) and enter:
make examples.quickstart.fibonacci
To run the program type:
./bin/fibonacci
This should print (time should be approximate):
fibonacci(10) == 55
elapsed time: 0.00186288 [s]
This run used the default settings, which calculate the tenth element of the
Fibonacci sequence. To declare which Fibonacci value you want to calculate, use
the --n-value
option. Additionally you can use the --hpx:threads
option to declare how many OS-threads you wish to use when running the program.
For instance, running:
./bin/fibonacci --n-value 20 --hpx:threads 4
Will yield:
fibonacci(20) == 6765
elapsed time: 0.233827 [s]
Walkthrough¶
The code needed to initialize the HPX runtime is the same as in the previous example:
int main(int argc, char* argv[])
{
// Configure application-specific options
boost::program_options::options_description
desc_commandline("Usage: " HPX_APPLICATION_STRING " [options]");
desc_commandline.add_options()
( "n-value",
boost::program_options::value<std::uint64_t>()->default_value(10),
"n value for the Fibonacci function")
;
// Initialize and run HPX
return hpx::init(desc_commandline, argc, argv);
}
The hpx::init
function in main()
starts the runtime system, and
invokes hpx_main()
as the first HPX-thread. The command line option
--n-value
is read in, a timer
(hpx::util::high_resolution_timer
) is set up to record the time it
takes to do the computation, the fibonacci
action is invoked
synchronously, and the answer is printed out.
int hpx_main(boost::program_options::variables_map& vm)
{
// extract command line argument, i.e. fib(N)
std::uint64_t n = vm["n-value"].as<std::uint64_t>();
{
// Keep track of the time required to execute.
hpx::util::high_resolution_timer t;
// Wait for fib() to return the value
fibonacci_action fib;
std::uint64_t r = fib(hpx::find_here(), n);
char const* fmt = "fibonacci({1}) == {2}\nelapsed time: {3} [s]\n";
hpx::util::format_to(std::cout, fmt, n, r, t.elapsed());
}
return hpx::finalize(); // Handles HPX shutdown
}
Upon a closer look we see that we’ve created a std::uint64_t
to store the
result of invoking our fibonacci_action
fib
. This action will
launch synchronously (as the work done inside of the action will be
asynchronous itself) and return the result of the Fibonacci sequence. But wait,
what is an action? And what is this fibonacci_action
? For starters,
an action is a wrapper for a function. By wrapping functions, HPX can
send packets of work to different processing units. These vehicles allow users
to calculate work now, later, or on certain nodes. The first argument to our
action is the location where the action should be run. In this
case, we just want to run the action on the machine that we are
currently on, so we use hpx::find_here
that we wish to calculate. To
further understand this we turn to the code to find where fibonacci_action
was defined:
// forward declaration of the Fibonacci function
std::uint64_t fibonacci(std::uint64_t n);
// This is to generate the required boilerplate we need for the remote
// invocation to work.
HPX_PLAIN_ACTION(fibonacci, fibonacci_action);
A plain action is the most basic form of action. Plain
actions wrap simple global functions which are not associated with any
particular object (we will discuss other types of actions in
Components and actions: Accumulator). In this block of code the function fibonacci()
is declared. After the declaration, the function is wrapped in an action
in the declaration HPX_PLAIN_ACTION
. This function takes two
arguments: the name of the function that is to be wrapped and the name of the
action that you are creating.
This picture should now start making sense. The function fibonacci()
is
wrapped in an action fibonacci_action
, which was run synchronously
but created asynchronous work, then returns a std::uint64_t
representing the
result of the function fibonacci()
. Now, let’s look at the function
fibonacci()
:
std::uint64_t fibonacci(std::uint64_t n)
{
if (n < 2)
return n;
// We restrict ourselves to execute the Fibonacci function locally.
hpx::naming::id_type const locality_id = hpx::find_here();
// Invoking the Fibonacci algorithm twice is inefficient.
// However, we intentionally demonstrate it this way to create some
// heavy workload.
fibonacci_action fib;
hpx::future<std::uint64_t> n1 =
hpx::async(fib, locality_id, n - 1);
hpx::future<std::uint64_t> n2 =
hpx::async(fib, locality_id, n - 2);
return n1.get() + n2.get(); // wait for the Futures to return their values
}
This block of code is much more straightforward and should look familiar from
the previous example. First, if (n < 2)
,
meaning n is 0 or 1, then we return 0 or 1 (recall the first element of the
Fibonacci sequence is 0 and the second is 1). If n is larger than 1 we spawn two
tasks using hpx::async
. Each of these futures represents an
asynchronous, recursive call to fibonacci
. As previously we wait for both
futures to finish computing, get the results, add them together, and return that
value as our result. The recursive call tree will continue until n is equal to 0
or 1, at which point the value can be returned because it is implicitly known.
When this termination condition is reached, the futures can then be added up,
producing the n-th value of the Fibonacci sequence.
Remote execution with actions: Hello world¶
This program will print out a hello world message on every OS-thread on every locality. The output will look something like this:
hello world from OS-thread 1 on locality 0
hello world from OS-thread 1 on locality 1
hello world from OS-thread 0 on locality 0
hello world from OS-thread 0 on locality 1
Setup¶
The source code for this example can be found here:
hello_world_distributed.cpp
.
To compile this program, go to your HPX build directory (see HPX build system for information on configuring and building HPX) and enter:
make examples.quickstart.hello_world_distributed
To run the program type:
./bin/hello_world_distributed
This should print:
hello world from OS-thread 0 on locality 0
To use more OS-threads use the command line option --hpx:threads
and
type the number of threads that you wish to use. For example, typing:
./bin/hello_world_distributed --hpx:threads 2
will yield:
hello world from OS-thread 1 on locality 0
hello world from OS-thread 0 on locality 0
Notice how the ordering of the two print statements will change with subsequent runs. To run this program on multiple localities please see the section How to use HPX applications with PBS.
Walkthrough¶
Now that you have compiled and run the code, let’s look at how the code works,
beginning with main()
:
//` Here is the main entry point. By using the include 'hpx/hpx_main.hpp' HPX
//` will invoke the plain old C-main() as its first HPX thread.
int main()
{
// Get a list of all available localities.
std::vector<hpx::naming::id_type> localities =
hpx::find_all_localities();
// Reserve storage space for futures, one for each locality.
std::vector<hpx::lcos::future<void> > futures;
futures.reserve(localities.size());
for (hpx::naming::id_type const& node : localities)
{
// Asynchronously start a new task. The task is encapsulated in a
// future, which we can query to determine if the task has
// completed.
typedef hello_world_foreman_action action_type;
futures.push_back(hpx::async<action_type>(node));
}
// The non-callback version of hpx::lcos::wait_all takes a single parameter,
// a vector of futures to wait on. hpx::wait_all only returns when
// all of the futures have finished.
hpx::wait_all(futures);
return 0;
}
In this excerpt of the code we again see the use of futures. This time the
futures are stored in a vector so that they can easily be accessed.
hpx::wait_all
is a family of functions that wait on for an
std::vector<>
of futures to become ready. In this piece of code, we are
using the synchronous version of hpx::wait_all
, which takes one
argument (the std::vector<>
of futures to wait on). This function will not
return until all the futures in the vector have been executed.
In Asynchronous execution with hpx::async and actions: Fibonacci we used hpx::find_here
to specify the
target of our actions. Here, we instead use
hpx::find_all_localities
, which returns an std::vector<>
containing the identifiers of all the machines in the system, including the one
that we are on.
As in Asynchronous execution with hpx::async and actions: Fibonacci our futures are set using
hpx::async<>
. The hello_world_foreman_action
is declared
here:
// Define the boilerplate code necessary for the function 'hello_world_foreman'
// to be invoked as an HPX action.
HPX_PLAIN_ACTION(hello_world_foreman, hello_world_foreman_action);
Another way of thinking about this wrapping technique is as follows: functions (the work to be done) are wrapped in actions, and actions can be executed locally or remotely (e.g. on another machine participating in the computation).
Now it is time to look at the hello_world_foreman()
function which was
wrapped in the action above:
void hello_world_foreman()
{
// Get the number of worker OS-threads in use by this locality.
std::size_t const os_threads = hpx::get_os_thread_count();
// Find the global name of the current locality.
hpx::naming::id_type const here = hpx::find_here();
// Populate a set with the OS-thread numbers of all OS-threads on this
// locality. When the hello world message has been printed on a particular
// OS-thread, we will remove it from the set.
std::set<std::size_t> attendance;
for (std::size_t os_thread = 0; os_thread < os_threads; ++os_thread)
attendance.insert(os_thread);
// As long as there are still elements in the set, we must keep scheduling
// HPX-threads. Because HPX features work-stealing task schedulers, we have
// no way of enforcing which worker OS-thread will actually execute
// each HPX-thread.
while (!attendance.empty())
{
// Each iteration, we create a task for each element in the set of
// OS-threads that have not said "Hello world". Each of these tasks
// is encapsulated in a future.
std::vector<hpx::lcos::future<std::size_t> > futures;
futures.reserve(attendance.size());
for (std::size_t worker : attendance)
{
// Asynchronously start a new task. The task is encapsulated in a
// future, which we can query to determine if the task has
// completed.
typedef hello_world_worker_action action_type;
futures.push_back(hpx::async<action_type>(here, worker));
}
// Wait for all of the futures to finish. The callback version of the
// hpx::lcos::wait_each function takes two arguments: a vector of futures,
// and a binary callback. The callback takes two arguments; the first
// is the index of the future in the vector, and the second is the
// return value of the future. hpx::lcos::wait_each doesn't return until
// all the futures in the vector have returned.
hpx::lcos::local::spinlock mtx;
hpx::lcos::wait_each(
hpx::util::unwrapping([&](std::size_t t) {
if (std::size_t(-1) != t)
{
std::lock_guard<hpx::lcos::local::spinlock> lk(mtx);
attendance.erase(t);
}
}),
futures);
}
}
Now, before we discuss hello_world_foreman()
, let’s talk about the
hpx::wait_each
function. hpx::lcos::wait_each
for each
one. The version of hpx::lcos::wait_each
invokes a callback function
provided by the user, supplying the callback function with the result of the
future.
In hello_world_foreman()
, an std::set<>
called attendance
keeps
track of which OS-threads have printed out the hello world message. When the
OS-thread prints out the statement, the future is marked as ready, and
hpx::lcos::wait_each
in hello_world_foreman()
. If it is not
executing on the correct OS-thread, it returns a value of -1, which causes
hello_world_foreman()
to leave the OS-thread id in attendance
.
std::size_t hello_world_worker(std::size_t desired)
{
// Returns the OS-thread number of the worker that is running this
// HPX-thread.
std::size_t current = hpx::get_worker_thread_num();
if (current == desired)
{
// The HPX-thread has been run on the desired OS-thread.
char const* msg = "hello world from OS-thread {1} on locality {2}\n";
hpx::util::format_to(hpx::cout, msg, desired, hpx::get_locality_id())
<< hpx::flush;
return desired;
}
// This HPX-thread has been run by the wrong OS-thread, make the foreman
// try again by rescheduling it.
return std::size_t(-1);
}
// Define the boilerplate code necessary for the function 'hello_world_worker'
// to be invoked as an HPX action (by a HPX future). This macro defines the
// type 'hello_world_worker_action'.
HPX_PLAIN_ACTION(hello_world_worker, hello_world_worker_action);
Because HPX features work stealing task schedulers, there is no way to guarantee that an action will be scheduled on a particular OS-thread. This is why we must use a guess-and-check approach.
Components and actions: Accumulator¶
The accumulator example demonstrates the use of components. Components are C++ classes that expose methods as a type of HPX action. These actions are called component actions.
Components are globally named, meaning that a component action can be called remotely (e.g. from another machine). There are two accumulator examples in HPX; accumulator.
In the Asynchronous execution with hpx::async and actions: Fibonacci and the Remote execution with actions: Hello world, we introduced plain actions, which wrapped global functions. The target of a plain action is an identifier which refers to a particular machine involved in the computation. For plain actions, the target is the machine where the action will be executed.
Component actions, however, do not target machines. Instead, they target component instances. The instance may live on the machine that we’ve invoked the component action from, or it may live on another machine.
The component in this example exposes three different functions:
reset()
- Resets the accumulator value to 0.add(arg)
- Addsarg
to the accumulators value.query()
- Queries the value of the accumulator.
This example creates an instance of the accumulator, and then allows the user to enter commands at a prompt, which subsequently invoke actions on the accumulator instance.
Setup¶
The source code for this example can be found here:
accumulator_client.cpp
.
To compile this program, go to your HPX build directory (see HPX build system for information on configuring and building HPX) and enter:
make examples.accumulators.accumulator
To run the program type:
./bin/accumulator_client
Once the program starts running, it will print the following prompt and then wait for input. An example session is given below:
commands: reset, add [amount], query, help, quit
> add 5
> add 10
> query
15
> add 2
> query
17
> reset
> add 1
> query
1
> quit
Walkthrough¶
Now, let’s take a look at the source code of the accumulator example. This
example consists of two parts: an HPX component library (a library that
exposes an HPX component) and a client application which uses the library.
This walkthrough will cover the HPX component library. The code for the client
application can be found here: accumulator_client.cpp
.
An HPX component is represented by two C++ classes:
- A server class - The implementation of the components functionality.
- A client class - A high-level interface that acts as a proxy for an instance of the component.
Typically, these two classes all have the same name, but the server class
usually lives in different sub-namespaces (server
). For example, the full
names of the two classes in accumulator are:
examples::server::accumulator
(server class)examples::accumulator
(client class)
The server class¶
The following code is from: accumulator.hpp
.
All HPX component server classes must inherit publicly from the HPX
component base class: hpx::components::component_base
The accumulator component inherits from
hpx::components::locking_hook
. This allows the runtime system to
ensure that all action invocations are serialized. That means that the system
ensures that no two actions are invoked at the same time on a given component
instance. This makes the component thread safe and no additional locking has to
be implemented by the user. Moreover, accumulator component is a component,
because it also inherits from hpx::components::component_base
(the
template argument passed to locking_hook is used as its base class). The
following snippet shows the corresponding code:
class accumulator
: public hpx::components::locking_hook<
hpx::components::component_base<accumulator> >
Our accumulator class will need a data member to store its value in, so let’s declare a data member:
argument_type value_;
The constructor for this class simply initializes value_
to 0:
accumulator() : value_(0) {}
Next, let’s look at the three methods of this component that we will be exposing as component actions:
/// Reset the components value to 0.
void reset()
{
// set value_ to 0.
value_ = 0;
}
/// Add the given number to the accumulator.
void add(argument_type arg)
{
// add value_ to arg, and store the result in value_.
value_ += arg;
}
/// Return the current value to the caller.
argument_type query() const
{
// Get the value of value_.
return value_;
}
Here are the action types. These types wrap the methods we’re exposing. The wrapping technique is very similar to the one used in the Asynchronous execution with hpx::async and actions: Fibonacci and the Remote execution with actions: Hello world:
HPX_DEFINE_COMPONENT_ACTION(accumulator, reset);
HPX_DEFINE_COMPONENT_ACTION(accumulator, add);
HPX_DEFINE_COMPONENT_ACTION(accumulator, query);
The last piece of code in the server class header is the declaration of the action type registration code:
HPX_REGISTER_ACTION_DECLARATION(
examples::server::accumulator::reset_action,
accumulator_reset_action);
HPX_REGISTER_ACTION_DECLARATION(
examples::server::accumulator::add_action,
accumulator_add_action);
HPX_REGISTER_ACTION_DECLARATION(
examples::server::accumulator::query_action,
accumulator_query_action);
Note
The code above must be placed in the global namespace.
The rest of the registration code is in
accumulator.cpp
///////////////////////////////////////////////////////////////////////////////
// Add factory registration functionality.
HPX_REGISTER_COMPONENT_MODULE();
///////////////////////////////////////////////////////////////////////////////
typedef hpx::components::component<
examples::server::accumulator
> accumulator_type;
HPX_REGISTER_COMPONENT(accumulator_type, accumulator);
///////////////////////////////////////////////////////////////////////////////
// Serialization support for accumulator actions.
HPX_REGISTER_ACTION(
accumulator_type::wrapped_type::reset_action,
accumulator_reset_action);
HPX_REGISTER_ACTION(
accumulator_type::wrapped_type::add_action,
accumulator_add_action);
HPX_REGISTER_ACTION(
accumulator_type::wrapped_type::query_action,
accumulator_query_action);
Note
The code above must be placed in the global namespace.
The client class¶
The following code is from accumulator.hpp
.
The client class is the primary interface to a component instance. Client classes are used to create components:
// Create a component on this locality.
examples::accumulator c = hpx::new_<examples::accumulator>(hpx::find_here());
and to invoke component actions:
c.add(hpx::launch::apply, 4);
Clients, like servers, need to inherit from a base class, this time,
hpx::components::client_base
:
class accumulator
: public hpx::components::client_base<
accumulator, server::accumulator
>
For readability, we typedef the base class like so:
typedef hpx::components::client_base<
accumulator, server::accumulator
> base_type;
Here are examples of how to expose actions through a client class:
There are a few different ways of invoking actions:
- Non-blocking: For actions which don’t have return types, or when we do not
care about the result of an action, we can invoke the action using
fire-and-forget semantics. This means that once we have asked HPX to compute
the action, we forget about it completely and continue with our computation.
We use
hpx::apply
to invoke an action in a non-blocking fashion.
void reset(hpx::launch::apply_policy)
{
HPX_ASSERT(this->get_id());
typedef server::accumulator::reset_action action_type;
hpx::apply<action_type>(this->get_id());
}
- Asynchronous: Futures, as demonstrated in Asynchronous execution with hpx::async: Fibonacci, Asynchronous execution with hpx::async and actions: Fibonacci, and the Remote execution with actions: Hello world, enable asynchronous action invocation. Here’s an example from the accumulator client class:
hpx::future<argument_type> query(hpx::launch::async_policy)
{
HPX_ASSERT(this->get_id());
typedef server::accumulator::query_action action_type;
return hpx::async<action_type>(hpx::launch::async, this->get_id());
}
- Synchronous: To invoke an action in a fully synchronous manner, we can
simply call
hpx::async
().get()
(e.g., create a future and immediately wait on it to be ready). Here’s an example from the accumulator client class:
void add(argument_type arg)
{
HPX_ASSERT(this->get_id());
typedef server::accumulator::add_action action_type;
action_type()(this->get_id(), arg);
}
Note that this->get_id()
references a data member of the
hpx::components::client_base
base class which identifies the server
accumulator instance.
hpx::naming::id_type
is a type which represents a global identifier
in HPX. This type specifies the target of an action. This is the type that is
returned by hpx::find_here
in which case it represents the
locality the code is running on.
Dataflow: Interest calculator¶
HPX provides its users with several different tools to simply express parallel concepts. One of these tools is a local control object (LCO) called dataflow. An LCO is a type of component that can spawn a new thread when triggered. They are also distinguished from other components by a standard interface which allow users to understand and use them easily. Dataflows, being a LCO, is triggered when the values it depends on become available. For instance, if you have a calculation X that depends on the result of three other calculations, you could set up a dataflow that would begin the calculation X as soon as the other three calculations have returned their values. Dataflows are set up to depend on other dataflows. It is this property that makes dataflow a powerful parallelization tool. If you understand the dependencies of your calculation, you can devise a simple algorithm which sets up a dependency tree to be executed. In this example, we calculate compound interest. To calculate compound interest, one must calculate the interest made in each compound period, and then add that interest back to the principal before calculating the interest made in the next period. A practical person would of course use the formula for compound interest:
where \(F\) is the future value, \(P\) is the principal value, \(i\) is the interest rate, and \(n\) is the number of compound periods.
Nevertheless, we have chosen for the sake of example to manually calculate the future value by iterating:
and
Setup¶
The source code for this example can be found here:
interest_calculator.cpp
.
To compile this program, go to your HPX build directory (see HPX build system for information on configuring and building HPX) and enter:
make examples.quickstart.interest_calculator
To run the program type:
./bin/interest_calculator --principal 100 --rate 5 --cp 6 --time 36
This should print:
Final amount: 134.01
Amount made: 34.0096
Walkthrough¶
Let us begin with main, here we can see that we again are using
Boost.Program Options to set our command line variables (see
Asynchronous execution with hpx::async and actions: Fibonacci for more details). These options set the principal,
rate, compound period, and time. It is important to note that the units of time
for cp
and time
must be the same.
int main(int argc, char ** argv)
{
options_description cmdline("Usage: " HPX_APPLICATION_STRING " [options]");
cmdline.add_options()
("principal", value<double>()->default_value(1000), "The principal [$]")
("rate", value<double>()->default_value(7), "The interest rate [%]")
("cp", value<int>()->default_value(12), "The compound period [months]")
("time", value<int>()->default_value(12*30),
"The time money is invested [months]")
;
return hpx::init(cmdline, argc, argv);
}
Next we look at hpx_main.
int hpx_main(variables_map & vm)
{
{
using hpx::shared_future;
using hpx::make_ready_future;
using hpx::dataflow;
using hpx::util::unwrapping;
hpx::naming::id_type here = hpx::find_here();
double init_principal=vm["principal"].as<double>(); //Initial principal
double init_rate=vm["rate"].as<double>(); //Interest rate
int cp=vm["cp"].as<int>(); //Length of a compound period
int t=vm["time"].as<int>(); //Length of time money is invested
init_rate/=100; //Rate is a % and must be converted
t/=cp; //Determine how many times to iterate interest calculation:
//How many full compound periods can fit in the time invested
// In non-dataflow terms the implemented algorithm would look like:
//
// int t = 5; // number of time periods to use
// double principal = init_principal;
// double rate = init_rate;
//
// for (int i = 0; i < t; ++i)
// {
// double interest = calc(principal, rate);
// principal = add(principal, interest);
// }
//
// Please note the similarity with the code below!
shared_future<double> principal = make_ready_future(init_principal);
shared_future<double> rate = make_ready_future(init_rate);
for (int i = 0; i < t; ++i)
{
shared_future<double> interest = dataflow(unwrapping(calc), principal, rate);
principal = dataflow(unwrapping(add), principal, interest);
}
// wait for the dataflow execution graph to be finished calculating our
// overall interest
double result = principal.get();
std::cout << "Final amount: " << result << std::endl;
std::cout << "Amount made: " << result-init_principal << std::endl;
}
return hpx::finalize();
}
Here we find our command line variables read in, the rate is converted from a
percent to a decimal, the number of calculation iterations is determined, and
then our shared_futures are set up. Notice that we first place our principal and
rate into shares futures by passing the variables init_principal
and
init_rate
using hpx::make_ready_future
.
In this way hpx::shared_future
<double>
principal and rate
will be initialized to init_principal
and init_rate
when
hpx::make_ready_future
<double>
returns a future containing
those initial values. These shared futures then enter the for loop and are
passed to interest
. Next principal
and interest
are passed to the
reassignment of principal
using a hpx::dataflow
. A dataflow
will first wait for its arguments to be ready before launching any callbacks, so
add
in this case will not begin until both principal
and interest
are ready. This loop continues for each compound period that must be calculated.
To see how interest
and principal
are calculated in the loop let us look
at calc_action
and add_action
:
// Calculate interest for one period
double calc(double principal, double rate)
{
return principal * rate;
}
///////////////////////////////////////////////////////////////////////////////
// Add the amount made to the principal
double add(double principal, double interest)
{
return principal + interest;
}
After the shared future dependencies have been defined in hpx_main, we see the following statement:
double result = principal.get();
This statement calls hpx::future::get
on the shared future
principal which had its value calculated by our for loop. The program will wait
here until the entire dataflow tree has been calculated and the value assigned
to result. The program then prints out the final value of the investment and the
amount of interest made by subtracting the final value of the investment from
the initial value of the investment.
Local to remote: 1D stencil¶
When developers write code they typically begin with a simple serial code and build upon it until all of the required functionality is present. The following set of examples were developed to demonstrate this iterative process of evolving a simple serial program to an efficient, fully distributed HPX application. For this demonstration, we implemented a 1D heat distribution problem. This calculation simulates the diffusion of heat across a ring from an initialized state to some user defined point in the future. It does this by breaking each portion of the ring into discrete segments and using the current segment’s temperature and the temperature of the surrounding segments to calculate the temperature of the current segment in the next timestep as shown by Fig. 2 below.
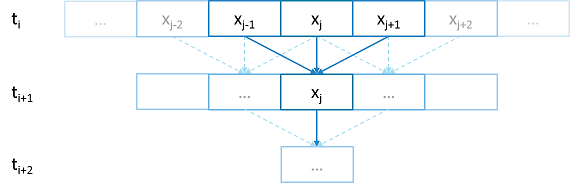
Fig. 2 Heat diffusion example program flow.
We parallelize this code over the following eight examples:
The first example is straight serial code. In this code we instantiate a vector
U
which contains two vectors of doubles as seen in the structure
stepper
.
struct stepper
{
// Our partition type
typedef double partition;
// Our data for one time step
typedef std::vector<partition> space;
// Our operator
static double heat(double left, double middle, double right)
{
return middle + (k*dt/(dx*dx)) * (left - 2*middle + right);
}
// do all the work on 'nx' data points for 'nt' time steps
space do_work(std::size_t nx, std::size_t nt)
{
// U[t][i] is the state of position i at time t.
std::vector<space> U(2);
for (space& s : U)
s.resize(nx);
// Initial conditions: f(0, i) = i
for (std::size_t i = 0; i != nx; ++i)
U[0][i] = double(i);
// Actual time step loop
for (std::size_t t = 0; t != nt; ++t)
{
space const& current = U[t % 2];
space& next = U[(t + 1) % 2];
next[0] = heat(current[nx-1], current[0], current[1]);
for (std::size_t i = 1; i != nx-1; ++i)
next[i] = heat(current[i-1], current[i], current[i+1]);
next[nx-1] = heat(current[nx-2], current[nx-1], current[0]);
}
// Return the solution at time-step 'nt'.
return U[nt % 2];
}
};
Each element in the vector of doubles represents a single grid point. To
calculate the change in heat distribution, the temperature of each grid point,
along with its neighbors, are passed to the function heat
. In order to
improve readability, references named current
and next
are created
which, depending on the time step, point to the first and second vector of
doubles. The first vector of doubles is initialized with a simple heat ramp.
After calling the heat function with the data in the current
vector, the
results are placed into the next
vector.
In example 2 we employ a technique called futurization. Futurization is a method
by which we can easily transform a code which is serially executed into a code
which creates asynchronous threads. In the simplest case this involves replacing
a variable with a future to a variable, a function with a future to a function,
and adding a .get()
at the point where a value is actually needed. The code
below shows how this technique was applied to the struct stepper
.
struct stepper
{
// Our partition type
typedef hpx::shared_future<double> partition;
// Our data for one time step
typedef std::vector<partition> space;
// Our operator
static double heat(double left, double middle, double right)
{
return middle + (k*dt/(dx*dx)) * (left - 2*middle + right);
}
// do all the work on 'nx' data points for 'nt' time steps
hpx::future<space> do_work(std::size_t nx, std::size_t nt)
{
using hpx::dataflow;
using hpx::util::unwrapping;
// U[t][i] is the state of position i at time t.
std::vector<space> U(2);
for (space& s : U)
s.resize(nx);
// Initial conditions: f(0, i) = i
for (std::size_t i = 0; i != nx; ++i)
U[0][i] = hpx::make_ready_future(double(i));
auto Op = unwrapping(&stepper::heat);
// Actual time step loop
for (std::size_t t = 0; t != nt; ++t)
{
space const& current = U[t % 2];
space& next = U[(t + 1) % 2];
// WHEN U[t][i-1], U[t][i], and U[t][i+1] have been computed, THEN we
// can compute U[t+1][i]
for (std::size_t i = 0; i != nx; ++i)
{
next[i] = dataflow(
hpx::launch::async, Op,
current[idx(i, -1, nx)], current[i], current[idx(i, +1, nx)]
);
}
}
// Now the asynchronous computation is running; the above for-loop does not
// wait on anything. There is no implicit waiting at the end of each timestep;
// the computation of each U[t][i] will begin when as soon as its dependencies
// are ready and hardware is available.
// Return the solution at time-step 'nt'.
return hpx::when_all(U[nt % 2]);
}
};
In example 2, we re-define our partition type as a shared_future
and, in
main
, create the object result
which is a future to a vector of
partitions. We use result
to represent the last vector in a string of
vectors created for each timestep. In order to move to the next timestep, the
values of a partition and its neighbors must be passed to heat
once the
futures that contain them are ready. In HPX, we have an LCO (Local Control
Object) named Dataflow which assists the programmer in expressing this
dependency. Dataflow allows us to pass the results of a set of futures to a
specified function when the futures are ready. Dataflow takes three types of
arguments, one which instructs the dataflow on how to perform the function call
(async or sync), the function to call (in this case Op
), and futures to the
arguments that will be passed to the function. When called, dataflow immediately
returns a future to the result of the specified function. This allows users to
string dataflows together and construct an execution tree.
After the values of the futures in dataflow are ready, the values must be pulled
out of the future container to be passed to the function heat
. In order to
do this, we use the HPX facility unwrapped
, which underneath calls
.get()
on each of the futures so that the function heat
will be passed
doubles and not futures to doubles.
By setting up the algorithm this way, the program will be able to execute as quickly as the dependencies of each future are met. Unfortunately, this example runs terribly slow. This increase in execution time is caused by the overheads needed to create a future for each data point. Because the work done within each call to heat is very small, the overhead of creating and scheduling each of the three futures is greater than that of the actual useful work! In order to amortize the overheads of our synchronization techniques, we need to be able to control the amount of work that will be done with each future. We call this amount of work per overhead grain size.
In example 3, we return to our serial code to figure out how to control the
grain size of our program. The strategy that we employ is to create “partitions”
of data points. The user can define how many partitions are created and how many
data points are contained in each partition. This is accomplished by creating
the struct partition
which contains a member object data_
, a vector of
doubles which holds the data points assigned to a particular instance of
partition
.
In example 4, we take advantage of the partition setup by redefining space
to be a vector of shared_futures with each future representing a partition. In
this manner, each future represents several data points. Because the user can
define how many data points are contained in each partition (and therefore how
many data points that are represented by one future) a user can now control the
grainsize of the simulation. The rest of the code was then futurized in the same
manner that was done in example 2. It should be noted how strikingly similar
example 4 is to example 2.
Example 4 finally shows good results. This code scales equivalently to the OpenMP version. While these results are promising, there are more opportunities to improve the application’s scalability. Currently this code only runs on one locality, but to get the full benefit of HPX we need to be able to distribute the work to other machines in a cluster. We begin to add this functionality in example 5.
In order to run on a distributed system, a large amount of boilerplate code must
be added. Fortunately, HPX provides us with the concept of a component
which saves us from having to write quite as much code. A component is an object
which can be remotely accessed using its global address. Components are made of
two parts: a server and a client class. While the client class is not required,
abstracting the server behind a client allows us to ensure type safety instead
of having to pass around pointers to global objects. Example 5 renames example
4’s struct partition
to partition_data
and adds serialization support.
Next we add the server side representation of the data in the structure
partition_server
. Partition_server
inherits from
hpx::components::component_base
which contains a server side component
boilerplate. The boilerplate code allows a component’s public members to be
accessible anywhere on the machine via its Global Identifier (GID). To
encapsulate the component, we create a client side helper class. This object
allows us to create new instances of our component, and access its members
without having to know its GID. In addition, we are using the client class to
assist us with managing our asynchrony. For example, our client class
partition
’s member function get_data()
returns a future to
partition_data get_data()
. This struct inherits its boilerplate code from
hpx::components::client_base
.
In the structure stepper
, we have also had to make some changes to
accommodate a distributed environment. In order to get the data from a
neighboring partition, which could be remote, we must retrieve the data from the
neighboring partitions. These retrievals are asynchronous and the function
heat_part_data
, which amongst other things calls heat
, should not be
called unless the data from the neighboring partitions have arrived. Therefore
it should come as no surprise that we synchronize this operation with another
instance of dataflow (found in heat_part
). This dataflow is passed futures
to the data in the current and surrounding partitions by calling get_data()
on each respective partition. When these futures are ready dataflow passes then
to the unwrapped
function, which extracts the shared_array of doubles and
passes them to the lambda. The lambda calls heat_part_data
on the
locality which the middle partition is on.
Although this example could run in distributed, it only runs on one
locality as it always uses hpx::find_here()
as the target for the
functions to run on.
In example 6, we begin to distribute the partition data on different nodes. This
is accomplished in stepper::do_work()
by passing the GID of the
locality where we wish to create the partition to the the partition
constructor.
for (std::size_t i = 0; i != np; ++i)
U[0][i] = partition(localities[locidx(i, np, nl)], nx, double(i));
We distribute the partitions evenly based on the number of localities used,
which is described in the function locidx
. Because some of the data needed
to update the partition in heat_part
could now be on a new locality,
we must devise a way of moving data to the locality of the middle
partition. We accomplished this by adding a switch in the function
get_data()
which returns the end element of the buffer data_
if it is
from the left partition or the first element of the buffer if the data is from
the right partition. In this way only the necessary elements, not the whole
buffer, are exchanged between nodes. The reader should be reminded that this
exchange of end elements occurs in the function get_data()
and therefore is
executed asynchronously.
Now that we have the code running in distributed, it is time to make some
optimizations. The function heat_part
spends most of its time on two tasks:
retrieving remote data and working on the data in the middle partition. Because
we know that the data for the middle partition is local, we can overlap the work
on the middle partition with that of the possibly remote call of get_data()
.
This algorithmic change which was implemented in example 7 can be seen below:
// The partitioned operator, it invokes the heat operator above on all elements
// of a partition.
static partition heat_part(partition const& left,
partition const& middle, partition const& right)
{
using hpx::dataflow;
using hpx::util::unwrapping;
hpx::shared_future<partition_data> middle_data =
middle.get_data(partition_server::middle_partition);
hpx::future<partition_data> next_middle = middle_data.then(
unwrapping(
[middle](partition_data const& m) -> partition_data
{
HPX_UNUSED(middle);
// All local operations are performed once the middle data of
// the previous time step becomes available.
std::size_t size = m.size();
partition_data next(size);
for (std::size_t i = 1; i != size-1; ++i)
next[i] = heat(m[i-1], m[i], m[i+1]);
return next;
}
)
);
return dataflow(
hpx::launch::async,
unwrapping(
[left, middle, right](partition_data next, partition_data const& l,
partition_data const& m, partition_data const& r) -> partition
{
HPX_UNUSED(left);
HPX_UNUSED(right);
// Calculate the missing boundary elements once the
// corresponding data has become available.
std::size_t size = m.size();
next[0] = heat(l[size-1], m[0], m[1]);
next[size-1] = heat(m[size-2], m[size-1], r[0]);
// The new partition_data will be allocated on the same locality
// as 'middle'.
return partition(middle.get_id(), next);
}
),
std::move(next_middle),
left.get_data(partition_server::left_partition),
middle_data,
right.get_data(partition_server::right_partition)
);
}
Example 8 completes the futurization process and utilizes the full potential of
HPX by distributing the program flow to multiple localities, usually defined as
nodes in a cluster. It accomplishes this task by running an instance of HPX main
on each locality. In order to coordinate the execution of the program
the struct stepper
is wrapped into a component. In this way, each
locality contains an instance of stepper which executes its own instance
of the function do_work()
. This scheme does create an interesting
synchronization problem that must be solved. When the program flow was being
coordinated on the head node the, GID of each component was known. However, when
we distribute the program flow, each partition has no notion of the GID of its
neighbor if the next partition is on another locality. In order to make
the GIDs of neighboring partitions visible to each other, we created two buffers
to store the GIDs of the remote neighboring partitions on the left and right
respectively. These buffers are filled by sending the GID of a newly created
edge partitions to the right and left buffers of the neighboring localities.
In order to finish the simulation the solution vectors named result
are then
gathered together on locality 0 and added into a vector of spaces
overall_result
using the HPX functions gather_id
and gather_here
.
Example 8 completes this example series which takes the serial code of example 1 and incrementally morphs it into a fully distributed parallel code. This evolution was guided by the simple principles of futurization, the knowledge of grainsize, and utilization of components. Applying these techniques easily facilitates the scalable parallelization of most applications.
Manual¶
The manual is your comprehensive guide to HPX. It contains detailed information on how to build and use HPX in different scenarios.
Getting HPX¶
There are HPX packages available for a few Linux distributions. The easiest way to get started with HPX is to use those packages. We keep an up-to-date list with instructions on the HPX Downloads page. If you use one of the available packages you can skip the next section, HPX build system, but we still recommend that you look through it as it contains useful information on how you can customize HPX at compile-time.
If there isn’t a package available for your platform you should either clone our repository:
or download a package with the source files from HPX Downloads.
HPX build system¶
The build system for HPX is based on CMake. CMake is a cross-platform build-generator tool. CMake does not build the project, it generates the files needed by your build tool (GNU make, Visual Studio, etc.) for building HPX.
This section gives an introduction on how to use our build system to build HPX and how to use HPX in your own projects.
CMake basics¶
CMake is a cross-platform build-generator tool. cmake does not build the project, it generates the files needed by your build tool (gnu make, visual studio, etc.) for building HPX.
in general, the hpx CMake scripts try to adhere to the general cmake policies on how to write CMake based projects.
Basic CMake usage¶
This section explains basic aspects of CMake, mostly for explaining those options which you may need on your day-to-day usage.
CMake comes with extensive documentation in the form of html files and on the
cmake executable itself. Execute cmake --help
for further help options.
CMake requires to know for which build tool it shall generate files (GNU make,
Visual Studio, Xcode, etc.). If not specified on the command line, it tries to
guess it based on you environment. Once identified the build tool, CMake uses
the corresponding Generator for creating files for your build tool. You can
explicitly specify the generator with the command line option -G "Name of the
generator"
. For knowing the available generators on your platform, execute:
cmake --help
This will list the generator names at the end of the help text. Generator names are case-sensitive. Example:
cmake -G "Visual Studio 9 2008" path/to/hpx
For a given development platform there can be more than one adequate generator.
If you use Visual Studio "NMake Makefiles"
is a generator you can use for
building with NMake. By default, CMake chooses the more specific generator
supported by your development environment. If you want an alternative generator,
you must tell this to CMake with the -G
option.
Quick start¶
We use here the command-line, non-interactive CMake interface.
Download and install CMake here: CMake Downloads. Version 3.3.2 is the minimally required version for HPX.
Open a shell. Your development tools must be reachable from this shell through the
PATH
environment variable.Create a directory for containing the build. It is not supported to build HPX on the source directory. cd to this directory:
mkdir mybuilddir cd mybuilddir
Execute this command on the shell replacing
path/to/hpx/
with the path to the root of your HPX source tree:cmake path/to/hpx
CMake will detect your development environment, perform a series of tests and will generate the files required for building HPX. CMake will use default values for all build parameters. See the CMake variables used to configure HPX section for fine-tuning your build.
This can fail if CMake can’t detect your toolset, or if it thinks that the
environment is not sane enough. In this case make sure that the toolset that you
intend to use is the only one reachable from the shell and that the shell itself
is the correct one for you development environment. CMake will refuse to build
MinGW makefiles if you have a POSIX shell reachable through the PATH
environment variable, for instance. You can force CMake to use various compilers
and tools. Please visit CMake Useful Variables
for a detailed overview of specific CMake variables.
Options and variables¶
Variables customize how the build will be generated. Options are boolean
variables, with possible values ON
/OFF
. Options and variables are
defined on the CMake command line like this:
cmake -DVARIABLE=value path/to/hpx
You can set a variable after the initial CMake invocation for changing its value. You can also undefine a variable:
cmake -UVARIABLE path/to/hpx
Variables are stored on the CMake cache. This is a file named CMakeCache.txt
on the root of the build directory. Do not hand-edit it.
Variables are listed here appending its type after a colon. It is correct to write the variable and the type on the CMake command line:
cmake -DVARIABLE:TYPE=value path/to/llvm/source
CMake supports the following variable types: BOOL
(options), STRING
(arbitrary string), PATH
(directory name), FILEPATH
(file name).
Prerequisites¶
Supported platforms¶
At this time, HPX supports the following platforms. Other platforms may work, but we do not test HPX with other platforms, so please be warned.
Name | Recommended Version | Minimum Version | Architectures |
---|---|---|---|
Linux | 3.2 | 2.6 | x86-32, x86-64, k1om |
BlueGeneQ | V1R2M0 | V1R2M0 | PowerPC A2 |
Windows | 7, Server 2008 R2 | Any Windows system | x86-32, x86-64 |
Mac OSX | Any OSX system | x86-64 |
Software and libraries¶
In the simplest case, HPX depends on Boost and Portable Hardware Locality (HWLOC). So, before you read further, please make sure you have a recent version of Boost installed on your target machine. HPX currently requires at least Boost V1.61.0 to work properly. It may build and run with older versions, but we do not test HPX with those versions, so please be warned.
Installing the Boost libraries is described in detail in Boost’s own Getting Started document. It is often possible to download the Boost libraries using the package manager of your distribution. Please refer to the corresponding documentation for your system for more information.
The installation of Boost is described in detail in Boost’s own Getting Started document. However, if you’ve never used the Boost libraries (or even if you have), here’s a quick primer: Installing Boost.
In addition, we require a recent version of hwloc in order to support thread pinning and NUMA awareness. See Installing Hwloc for instructions on building Portable Hardware Locality (HWLOC).
HPX is written in 99.99% Standard C++ (the remaining 0.01% is platform specific assembly code). As such HPX is compilable with almost any standards compliant C++ compiler. A compiler supporting the C++11 Standard is highly recommended. The code base takes advantage of C++11 language features when available (move semantics, rvalue references, magic statics, etc.). This may speed up the execution of your code significantly. We currently support the following C++ compilers: GCC, MSVC, ICPC and clang. For the status of your favorite compiler with HPX visit HPX Buildbot Website.
Name | Recommended version | Minimum version | Notes |
Compilers | |||
GNU Compiler Collection (g++) | 4.9 or newer | 4.9 | |
Intel Composer XE Suites | 2014 or newer | 2014 | |
clang: a C language family frontend for LLVM | 3.8 or newer | 3.8 | |
Build System | |||
CMake | 3.9.0 | 3.3.2 | Cuda support 3.9 |
Required Libraries | |||
Boost C++ Libraries | 1.67.0 or newer | 1.61.0 | |
Portable Hardware Locality (HWLOC) | 1.11 | 1.2 (Xeon Phi: 1.6) |
Note
When compiling with the Intel Compiler on Linux systems, we only support C++
Standard Libraries provided by gcc 4.8 and upwards. If the g++
in your
path is older than 4.8, please specify the path of a newer g++
by setting
CMAKE_CXX_FLAGS='-gxx-name=/path/to/g++'
via CMake.
Note
When building Boost using gcc please note that it is always a good idea to
specify a cxxflags=-std=c++11
command line argument to b2
(bjam
).
Note however, that this is absolutely necessary when using gcc V5.2 and
above.
Name | Recommended version | Minimum version | Notes |
Compilers | |||
Visual C++ (x64) | 2015 | 2015 | |
Build System | |||
CMake | 3.9.0 | 3.3.2 | |
Required Libraries | |||
Boost | 1.67.0 or newer | 1.61.0 | |
Portable Hardware Locality (HWLOC) | 1.11 | 1.5 |
Note
You need to build the following Boost libraries for HPX: Boost.Filesystem, Boost.ProgramOptions, Boost.Regex, and Boost.System. The following are not needed by default, but are required in certain configurations: Boost.Chrono, Boost.DateTime, Boost.Log, Boost.LogSetup, and Boost.Thread.
Depending on the options you chose while building and installing HPX, you will find that HPX may depend on several other libraries such as those listed below.
Note
In order to use a high speed parcelport, we currently recommend configuring
HPX to use MPI so that MPI can be used for communication between different
localities. Please set the CMake variable MPI_CXX_COMPILER
to your MPI
C++ compiler wrapper if not detected automatically.
Name | Recommended version | Minimum version | Notes |
google-perftools | 1.7.1 | 1.7.1 | Used as a replacement for the system allocator, and for allocation diagnostics. |
libunwind | 0.99 | 0.97 | Dependency of google-perftools on x86-64, used for stack unwinding. |
Open MPI | 1.10.1 | 1.8.0 | Can be used as a highspeed communication library backend for the parcelport. |
Note
When using OpenMPI please note that Ubuntu (notably 18.04 LTS) and older
Debian ship an OpenMPI 2.x built with --enable-heterogeneous
which may
cause communication failures at runtime and should not be used.
Name | Recommended version | Minimum version | Notes |
Performance Application Programming Interface (PAPI) | Used for accessing hardware performance data. | ||
jemalloc | 2.1.2 | 2.1.0 | Used as a replacement for the system allocator. |
Hierarchical Data Format V5 (HDF5) | 1.8.7 | 1.6.7 | Used for data I/O in some example applications. See important note below. |
Name | Recommended version | Minimum version | Notes |
Hierarchical Data Format V5 (HDF5) | 1.8.7 | 1.6.7 | Used for data I/O in some example applications. See important note below. |
Important
The C++ HDF5 libraries must be compiled with enabled thread safety support. This has to be explicitly specified while configuring the HDF5 libraries as it is not the default. Additionally, you must set the following environment variables before configuring the HDF5 libraries (this part only needs to be done on Linux):
export CFLAGS='-DHDatexit=""'
export CPPFLAGS='-DHDatexit=""'
Documentation¶
To build the HPX documentation you need recent versions of the following packages:
python
(2 or 3)sphinx
(Python package)sphinx_rtd_theme
(Python package)breathe
(Python package)doxygen
If the Python dependencies are not available through your system package
manager you can install them using the Python package manager pip
:
pip install --user sphinx sphinx_rtd_theme breathe
You may need to set the following CMake variables to make sure CMake can find the required dependencies.
Installing Boost¶
Important
When building Boost using gcc please note that it is always a good idea to
specify a cxxflags=-std=c++11
command line argument to b2
(bjam
).
Note however, that this is absolutely necessary when using gcc V5.2 and
above.
Important
On Windows, depending on the installed versions of Visual Studio, you might
also want to pass the correct toolset to the b2
command depending on
which version of the IDE you want to use. In addition, passing
address-model=64
is highly recommended. It might be also necessary to add
command line argument --build-type=complete
to the b2
command on the
Windows platform.
The easiest way to create a working Boost installation is to compile Boost from
sources yourself. This is particularly important as many high performance
resources, even if they have Boost installed, usually only provide you with an
older version of Boost. We suggest you download the most recent release of the
Boost libraries from here: Boost Downloads. Unpack the downloaded archive
into a directory of your choosing. We will refer to this directory a $BOOST
.
Building and installing the Boost binaries is simple, regardless what platform you are on the basic instructions are as follows (with possible additional platform-dependent command line arguments):
cd $BOOST
bootstrap --prefix=<where to install boost>
./b2 -j<N>
./b2 install
where: <where to install boost>
is the directory the built binaries will be
installed to, and <N>
is the number of cores to use to build the Boost
binaries.
After the above sequence of commands has been executed (this may take a while!)
you will need to specify the directory where Boost was installed as
BOOST_ROOT
(<where to install boost>
) while executing cmake for HPX as
explained in detail in the sections How to install HPX on Unix variants and
How to install HPX on Windows.
Installing Hwloc¶
Note
These instructions are for everything except Windows. On Windows there is no
need to build hwloc. Instead download the latest release, extract the files,
and set HWLOC_ROOT
during cmake configuration to the directory in which
you extracted the files.
We suggest you download the most recent release of hwloc from here:
Hwloc Downloads. Unpack the downloaded archive into a directory of your
choosing. We will refer to this directory as $HWLOC
.
To build hwloc run:
cd $HWLOC
./configure --prefix=<where to install hwloc>
make -j<N> install
where: <where to install hwloc>
is the directory the built binaries will be
installed to, and <N>
is the number of cores to use to build hwloc.
After the above sequence of commands has been executed you will need to specify
the directory where Hwloc was installed as HWLOC_ROOT
(<where to install
hwloc>
) while executing cmake for HPX as explained in detail in the sections
How to install HPX on Unix variants and How to install HPX on Windows.
Please see Hwloc Documentation for more information about Hwloc.
Building HPX¶
Basic information¶
Once CMake has been run, the build process can be started. The HPX build process is highly configurable through CMake and various CMake variables influence the build process. The build process consists of the following parts:
- The HPX core libraries (target core): This forms the basic set of HPX
libraries. The generated targets are:
hpx
: The core HPX library (always enabled).hpx_init
: The HPX initialization library that applications need to link against to define the HPX entry points (disabled for static builds).hpx_wrap
: The HPX static library used to determine the runtime behavior of HPX code and respective entry points forhpx_main.h
iostreams_component
: The component used for (distributed) IO (always enabled).component_storage_component
: The component needed for migration to persistent storage.unordered_component
: The component needed for a distributed (partitioned) hash table.partioned_vector_component
: The component needed for a distributed (partitioned) vector.memory_component
: A dynamically loaded plugin that exposed memory based performance counters (only available on Linux).io_counter_component
: A dynamically loaded plugin plugin that exposes I/O performance counters (only available on Linux).papi_component
: A dynamically loaded plugin that exposes PAPI performance counters (enabled withHPX_WITH_PAPI:BOOL
, default isOff
).
- HPX Examples (target
examples
): This target is enabled by default and builds all HPX examples (disable by settingHPX_WITH_EXAMPLES:BOOL
=Off
). HPX examples are part of theall
target and are included in the installation if enabled. - HPX Tests (target
tests
): This target builds the HPX test suite and is enabled by default (disable by settingHPX_WITH_TESTS:BOOL
=Off
). They are not built by theall
target and have to be built separately. - HPX Documentation (target
docs
): This target builds the documentation, this is not enabled by default (enable by settingHPX_WITH_DOCUMENTATION:BOOL
=On
. For more information see Documentation.
For a complete list of available CMake variables that influence the build of HPX see CMake variables used to configure HPX.
The variables can be used to refine the recipes that can be found Platform specific build recipes which show some basic steps on how to build HPX for a specific platform.
In order to use HPX, only the core libraries are required (the ones marked as
optional above are truly optional). When building against HPX, the CMake
variable HPX_LIBRARIES
will contain hpx
and hpx_init
(for pkgconfig,
those are added to the Libs
sections). In order to use the optional
libraries, you need to specify them as link dependencies in your build (See
Creating HPX projects).
As HPX is a modern C++ Library we require a certain minimal set of features from the C++11 standard. In addition, we make use of certain C++14 features if the used compiler supports them. This means that the HPX build system will try to determine the highest support C++ standard flavor and check for availability of those features. That is, the default will be the highest C++ standard version available. If you want to force HPX to use a specific C++ standard version you can use the following CMake variables:
HPX_WITH_CXX0X
: Enables Pre-C++11 support (This is the minimal required mode on older gcc versions).HPX_WITH_CXX11
: Enables C++11 supportHPX_WITH_CXX14
: Enables C++14 supportHPX_WITH_CXX17
: Enables C++17 supportHPX_WITH_CXX2A
: Enables (experimental) C++20 support
Build types¶
CMake can be configured to generate project files suitable for builds that
have enabled debugging support or for an optimized build (without debugging
support). The CMake variable used to set the build type is
CMAKE_BUILD_TYPE
(for more information see the CMake Documentation).
Available build types are:
- Debug: Full debug symbols available and additional assertions to help
debugging. To enable the debug build type for the HPX API, the C++ Macro
HPX_DEBUG
is defined. - RelWithDebInfo: Release build with debugging symbols. This is most useful for profiling applications
- Release: Release build. This disables assertions and enables default compiler optimizations.
- RelMinSize: Release build with optimizations for small binary sizes.
Important
We currently don’t guarantee ABI compatibility between Debug and Release
builds. Please make sure that applications built against HPX use the same
build type as you used to build HPX. For CMake builds, this means that
the CMAKE_BUILD_TYPE
variables have to match and for projects not using
CMake, the HPX_DEBUG
macro has to be set in debug mode.
Platform specific notes¶
Some platforms require to have special link and/or compiler flags specified to build HPX. This is handled via CMake’s support for different toolchains (see cmake-toolchains(7) for more information). This is also used for cross compilation.
HPX ships with a set of toolchains that can be used for compilation of HPX itself and applications depending on HPX. Please see CMake toolchains shipped with HPX for more information.
In order to enable full static linking with the libraries, the CMake variable
HPX_WITH_STATIC_LINKING:BOOL
has to be set to On
.
Debugging applications using core files¶
For HPX to generate useful core files, HPX has to be compiled without signal
and exception handlers
HPX_WITH_DISABLED_SIGNAL_EXCEPTION_HANDLERS:BOOL
. If this option is
not specified, the signal handlers change the application state. For example,
after a segmentation fault the stack trace will show the signal handler.
Similarly, unhandled exceptions are also caught by the these handlers and the
stack trace will not point to the location where the unhandled exception was
thrown.
In general, core files are a helpful tool to inspect the state of the application at the moment of the crash (post-mortem debugging), without the need of attaching a debugger beforehand. This approach to debugging is especially useful if the error cannot be reliably reproduced, as only a single crashed application run is required to gain potentially helpful information like a stacktrace.
To debug with core files, the operating system first has to be told to actually write them. On most unix systems this can be done by calling:
ulimit -c unlimited
in the shell. Now the debugger can be started up with:
gdb <application> <core file name>
The debugger should now display the last state of the application. The default
file name for core files is core
.
Platform specific build recipes¶
Note
The following build recipes are mostly user-contributed and may be outdated. We always welcome updated and new build recipes.
How to install HPX on Unix variants¶
Create a build directory. HPX requires an out-of-tree build. This means you will be unable to run CMake in the HPX source tree.
cd hpx mkdir my_hpx_build cd my_hpx_build
Invoke CMake from your build directory, pointing the CMake driver to the root of your HPX source tree.
cmake -DBOOST_ROOT=/root/of/boost/installation \ -DHWLOC_ROOT=/root/of/hwloc/installation [other CMake variable definitions] \ /path/to/source/tree
for instance:
cmake -DBOOST_ROOT=~/packages/boost -DHWLOC_ROOT=/packages/hwloc -DCMAKE_INSTALL_PREFIX=~/packages/hpx ~/downloads/hpx_0.9.10
Invoke GNU make. If you are on a machine with multiple cores, add the -jN flag to your make invocation, where N is the number of parallel processes HPX gets compiled with.
gmake -j4
Caution
Compiling and linking HPX needs a considerable amount of memory. It is advisable that at least 2 GB of memory per parallel process is available.
Note
Many Linux distributions use
make
as an alias forgmake
.To complete the build and install HPX:
gmake install
Important
These commands will build and install the essential core components of HPX only. In order to build and run the tests, please invoke:
gmake tests && gmake test
and in order to build (and install) all examples invoke:
cmake -DHPX_WITH_EXAMPLES=On . gmake examples gmake install
For more detailed information about using CMake please refer its documentation
and also the section Building HPX. Please pay special attention to the
section about HPX_WITH_MALLOC:STRING
as this is crucial for getting
decent performance.
How to install HPX on OS X (Mac)¶
This section describes how to build HPX for OS X (Mac).
To build Boost with Clang and make it link to libc++ as standard library, you’ll
need to set up either of the following in your ~/user-config.jam
file:
# user-config.jam (put this file into your home directory)
# ...
using clang
:
: "/usr/bin/clang++"
: <cxxflags>"-std=c++11 -fcolor-diagnostics"
<linkflags>"-stdlib=libc++ -L/path/to/libcxx/lib"
;
(Again, remember to replace /path/to
with whatever you used earlier.)
You can then use as build command either:
b2 --build-dir=/tmp/build-boost --layout=versioned toolset=clang install -j4
or:
b2 --build-dir=/tmp/build-boost --layout=versioned toolset=clang install -j4
We verified this using Boost V1.53. If you use a different version, just
remember to replace /usr/local/include/boost-1_53
with whatever include prefix
you had in your installation.
cd /path/to
git clone https://github.com/STEllAR-GROUP/hpx.git
mkdir build-hpx && cd build-hpx
To build with Clang 3.2, execute:
cmake ../hpx \
-DCMAKE_CXX_COMPILER=clang++ \
-DBOOST_INCLUDE_DIR=/usr/local/include/boost-1_53 \
-DBOOST_LIBRARY_DIR=/usr/local/lib \
-DBOOST_SUFFIX=-clang-darwin32-mt-1_53 \
make
To build with Clang 3.3 (trunk), execute:
cmake ../hpx \
-DCMAKE_CXX_COMPILER=clang++ \
-DBOOST_INCLUDE_DIR=/usr/local/include/boost-1_53 \
-DBOOST_LIBRARY_DIR=/usr/local/lib \
-DBOOST_SUFFIX=-clang-darwin33-mt-1_53 \
make
For more detailed information about using CMake please refer its documentation and to the section Building HPX for.
Alternatively, you can install a recent version of gcc as well as all required libraries via MacPorts:
Install MacPorts
Install CMake, gcc 4.8, and hwloc:
sudo port install gcc48 sudo port install hwloc
You may also want:
sudo port install cmake sudo port install git-core
Make this version of gcc your default compiler:
sudo port install gcc_select sudo port select gcc mp-gcc48
Build Boost manually (the Boost package of MacPorts is built with Clang, and unfortunately doesn’t work with a GCC-build version of HPX):
wget https://dl.bintray.com/boostorg/release/1.69.0/source/boost_1_69_0.tar.bz2 tar xjf boost_1_69_0.tar.bz2 pushd boost_1_69_0 export BOOST_ROOT=$HOME/boost_1_69_0 ./bootstrap.sh --prefix=$BOOST_DIR ./b2 -j8 ./b2 -j8 install export DYLD_LIBRARY_PATH=$DYLD_LIBRARY_PATH:$BOOST_ROOT/lib popd
Build HPX:
git clone https://github.com/STEllAR-GROUP/hpx.git mkdir hpx-build pushd hpx-build export HPX_ROOT=$HOME/hpx cmake -DCMAKE_C_COMPILER=gcc \ -DCMAKE_CXX_COMPILER=g++ \ -DCMAKE_FORTRAN_COMPILER=gfortran \ -DCMAKE_C_FLAGS="-Wno-unused-local-typedefs" \ -DCMAKE_CXX_FLAGS="-Wno-unused-local-typedefs" \ -DBOOST_ROOT=$BOOST_ROOT \ -DHWLOC_ROOT=/opt/local \ -DCMAKE_INSTALL_PREFIX=$HOME/hpx \ $(pwd)/../hpx make -j8 make -j8 install export DYLD_LIBRARY_PATH=$DYLD_LIBRARY_PATH:$HPX_ROOT/lib/hpx popd
Note that you need to set
BOOST_ROOT
,HPX_ROOT
andDYLD_LIBRARY_PATH
(for bothBOOST_ROOT
andHPX_ROOT
every time you configure, build, or run an HPX application.If you want to use HPX with MPI, you need to enable the MPI parcelport, and also specify the location of the MPI wrapper scripts. This can be done e.g. with the following command:
cmake -DHPX_WITH_PARCELPORT_MPI=ON \ -DCMAKE_C_COMPILER=gcc \ -DCMAKE_CXX_COMPILER=g++ \ -DCMAKE_FORTRAN_COMPILER=gfortran \ -DMPI_C_COMPILER=openmpicc \ -DMPI_CXX_COMPILER=openmpic++ \ -DMPI_FORTRAN_COMPILER=openmpif90 \ -DCMAKE_C_FLAGS="-Wno-unused-local-typedefs" \ -DCMAKE_CXX_FLAGS="-Wno-unused-local-typedefs" \ -DBOOST_ROOT=$BOOST_DIR \ -DHWLOC_ROOT=/opt/local \ -DCMAKE_INSTALL_PREFIX=$HOME/hpx $(pwd)/../hpx
How to install HPX on Windows¶
- Download the Boost c++ libraries from Boost Downloads
- Install the boost library as explained in the section Installing Boost
- Install the hwloc library as explained in the section Installing Hwloc
- Download the latest version of CMake binaries, which are located under the platform section of the downloads page at CMake Downloads.
- Download the latest version of HPX from the STE||AR website: HPX Downloads.
- Create a build folder. HPX requires an out-of-tree-build. This means that you will be unable to run CMake in the HPX source folder.
- Open up the CMake GUI. In the input box labelled “Where is the source code:”, enter the full path to the source folder. The source directory is one where the sources were checked out. CMakeLists.txt files in the source directory as well as the subdirectories describe the build to CMake. In addition to this, there are CMake scripts (usually ending in .cmake) stored in a special CMake directory. CMake does not alter any file in the source directory and doesn’t add new ones either. In the input box labelled “Where to build the binaries:”, enter the full path to the build folder you created before. The build directory is one where all compiler outputs are stored, which includes object files and final executables.
- Add CMake variable definitions (if any) by clicking the “Add Entry” button.
There are two required variables you need to define:
BOOST_ROOT
andHWLOC_ROOT
These (PATH
) variables need to be set to point to the root folder of your Boost and Portable Hardware Locality (HWLOC) installations. It is recommended to set the variableCMAKE_INSTALL_PREFIX
as well. This determines where the HPX libraries will be built and installed. If this (PATH
) variable is set, it has to refer to the directory where the built HPX files should be installed to. - Press the “Configure” button. A window will pop up asking you which compilers to use. Select the Visual Studio 10 (64Bit) compiler (it usually is the default if available). The Visual Studio 2012 (64Bit) and Visual Studio 2013 (64Bit) compilers are supported as well. Note that while it is possible to build HPX for x86, we don’t recommend doing so as 32 bit runs are severely restricted by a 32 bit Windows system limitation affecting the number of HPX threads you can create.
- Press “Configure” again. Repeat this step until the “Generate” button becomes clickable (and until no variable definitions are marked red anymore).
- Press “Generate”.
- Open up the build folder, and double-click hpx.sln.
- Build the INSTALL target.
For more detailed information about using CMake please refer its documentation and also the section Building HPX.
Download the CMake V3.4.3 installer (or latest version) from here
Download the Portable Hardware Locality (HWLOC) V1.11.0 (or latest version) from here and unpack it.
Download the latest Boost libraries from here and unpack them.
Build the boost DLLs and LIBs by using these commands from Command Line (or PowerShell). Open CMD/PowerShell inside the Boost dir and type in:
bootstrap.bat
This batch file will set up everything needed to create a successful build. Now execute:
b2.exe link=shared variant=release,debug architecture=x86 address-model=64 threading=multi --build-type=complete install
This command will start a (very long) build of all available Boost libraries. Please, be patient.
Open CMake-GUI.exe and set up your source directory (input field ‘Where is the source code’) to the base directory of the source code you downloaded from HPX’s GitHub pages. Here’s an example of my CMake path settings which point to my
Documents/GitHub/hpx
folder:Fig. 3 Example CMake path settings.
Inside the ‘Where is the source-code’ enter the base directory of your HPX source directory (do not enter the “src” sub-directory!) Inside ‘Where to build the binaries’ you should put in the path where all the building process will happen. This is important because the building machinery will do an “out-of-tree” build. CMake is not touching or changing in any way the original source files. Instead, it will generate Visual Studio Solution Files which will build HPX packages out of the HPX source tree.
Set three new environment variables (in CMake, not in Windows environment, by the way):
BOOST_ROOT
,HWLOC_ROOT
,CMAKE_INSTALL_PREFIX
. The meaning of these variables is as follows:BOOST_ROOT
the root directory of the unpacked Boost headers/cpp files.HWLOC_ROOT
the root directory of the unpacked Portable Hardware Locality files.CMAKE_INSTALL_PREFIX
the “root directory” where the future builds of HPX should be installed to.Note
HPX is a BIG software collection and I really don’t recommend using the default
C:\Program Files\hpx
. I prefer simpler paths without white space, likeC:\bin\hpx
orD:\bin\hpx
etc.
To insert new env-vars click on “Add Entry” and then insert the name inside “Name”, select
PATH
as Type and put the path-name in “Path” text field. Repeat this for the first three variables.This is how variable insertion looks like:
Fig. 4 Example CMake adding entry.
Alternatively you could provide
BOOST_LIBRARYDIR
instead ofBOOST_ROOT
with a difference thatBOOST_LIBRARYDIR
should point to the subdirectory inside Boost root where all the compiled DLLs/LIBs are. I myself have usedBOOST_LIBRARYDIR
which pointed to thebin.v2
subdirectory under the Boost rootdir. Important is to keep the meanings of these two variables separated from each other:BOOST_DIR
points to the ROOT folder of the boost library.BOOST_LIBRARYDIR
points to the subdir inside Boost root folder where the compiled binaries are.Click the ‘Configure’ button of CMake-GUI. You will be immediately presented a small window where you can select the C++ compiler to be used within Visual Studio. In my case I have used the latest v14 (a.k.a C++ 2015) but older versions should be sufficient too. Make sure to select the 64Bit compiler
After the generate process has finished successfully click the ‘Generate’ button. Now, CMake will put new VS Solution files into the BUILD folder you selected at the beginning.
Open Visual Studio and load the
HPX.sln
from your build folder.Go to
CMakePredefinedTargets
and build theINSTALL
project:Fig. 5 Visual Studio INSTALL target.
It will take some time to compile everything and in the end you should see an output similar to this one:
Fig. 6 Visual Studio build output.
How to Install HPX on BlueGene/Q¶
So far we only support BGClang for compiling HPX on the BlueGene/Q.
Check if BGClang is available on your installation. If not obtain and install a copy from the BGClang trac page.
Build (and install) a recent version of Hwloc Downloads. With the following commands:
./configure \ --host=powerpc64-bgq-linux \ --prefix=$HOME/install/hwloc \ --disable-shared \ --enable-static \ CPPFLAGS='-I/bgsys/drivers/ppcfloor -I/bgsys/drivers/ppcfloor/spi/include/kernel/cnk/' make make install
Build (and install) a recent version of Boost, using BGClang. To build Boost with BGClang, you’ll need to set up the following in your Boost
~/user-config.jam
file:# user-config.jam (put this file into your home directory) using clang : : bgclang++11 : ;
You can then use this as your build command:
./bootstrap.sh ./b2 --build-dir=/tmp/build-boost --layout=versioned toolset=clang -j12
Clone the master HPX git repository (or a stable tag):
git clone git://github.com/STEllAR-GROUP/hpx.git
Generate the HPX buildfiles using cmake:
cmake -DHPX_PLATFORM=BlueGeneQ \ -DCMAKE_TOOLCHAIN_FILE=/path/to/hpx/cmake/toolchains/BGQ.cmake \ -DCMAKE_CXX_COMPILER=bgclang++11 \ -DMPI_CXX_COMPILER=mpiclang++11 \ -DHWLOC_ROOT=/path/to/hwloc/installation \ -DBOOST_ROOT=/path/to/boost \ -DHPX_WITH_MALLOC=system \ /path/to/hpx
To complete the build and install HPX:
make -j24 make install
This will build and install the essential core components of HPX only. Use:
make -j24 examples make -j24 install
to build and install the examples.
How to Install HPX on the Xeon Phi¶
Download Boost Downloads for Linux and unpack the retrieved tarball.
Adapt your
~/user-config.jam
to contain the following lines:## Toolset to be used for compiling for the host using intel : host : : <cxxflags>"-std=c++0x" ; ## Toolset to be used for compiling for the Xeon Phi using intel : mic : : <cxxflags>"-std=c++0x -mmic" <linkflags>"-std=c++0x -mmic" ;
Change to the directory you unpacked boost in (from now on referred to as
$BOOST_ROOT
) and execute the following commands:./bootstrap.sh ./b2 toolset=intel-mic -j<N>
You should now have all the required boost libraries.
Download Hwloc Downloads, unpack the retrieved tarball and change to the newly created directory.
Run the configure-make-install procedure as follows:
CC=icc CFLAGS=-mmic CXX=icpc CXXFLAGS=-mmic LDFLAGS=-mmic ./configure --host=x86_64-k1om-linux --prefix=$HWLOC_ROOT make make install
Important
The minimally required version of the Portable Hardware Locality (HWLOC) library on the Intel Xeon Phi is V1.6.
You now have a working hwloc installation in $HWLOC_ROOT
.
After all the prerequisites have been successfully installed, we can now start building and installing HPX. The build procedure is almost the same as for How to install HPX on Unix variants with the sole difference that you have to enable the Xeon Phi in the CMake Build system. This is achieved by invoking CMake in the following way:
cmake \
-DCMAKE_TOOLCHAIN_FILE=/path/to/hpx/cmake/toolchains/XeonPhi.cmake \
-DBOOST_ROOT=$BOOST_ROOT \
-DHWLOC_ROOT=$HWLOC_ROOT \
/path/to/hpx
For more detailed information about using CMake please refer to its
documentation and to the section Building HPX. Please pay special
attention to the section about HPX_WITH_MALLOC:STRING
as this is
crucial for getting decent performance on the Xeon Phi.
How to install HPX on Fedora distributions¶
Important
There are official HPX packages for Fedora. Unless you want to customize your build you may want to start off with the official packages. Instructions can be found on the HPX Downloads page.
Note
This section of the manual is based off of our collaborators Patrick Diehl’s blog post Installing HPX on Fedora 22.
Install all packages for minimal installation:
sudo dnf install gcc-c++ cmake boost-build boost boost-devel hwloc-devel \ hwloc gcc-gfortran papi-devel gperftools-devel docbook-dtds \ docbook-style-xsl libsodium-devel doxygen boost-doc hdf5-devel \ fop boost-devel boost-openmpi-devel boost-mpich-devel
Get the development branch of HPX:
git clone https://github.com/STEllAR-GROUP/hpx.git
Configure it with CMake:
cd hpx mkdir build cd build cmake -DCMAKE_INSTALL_PREFIX=/opt/hpx .. make -j make install
Note
To build HPX without examples use:
cmake -DCMAKE_INSTALL_PREFIX=/opt/hpx -DHPX_WITH_EXAMPLES=Off ..
Add the library path of HPX to ldconfig:
sudo echo /opt/hpx/lib > /etc/ld.so.conf.d/hpx.conf sudo ldconfig
How to install HPX on Arch distributions¶
Important
There are HPX packages for Arch in the AUR. Unless you want to customize your build you may want to start off with those. Instructions can be found on the HPX Downloads page.
Install all packages for a minimal installation:
sudo pacman -S gcc clang cmake boost hwloc gperftools
For building the documentation you will need to further install the following:
sudo pacman -S doxygen python-pip pip install --user sphinx sphinx_rtd_theme breathe
The rest of the installation steps are same as provided with Fedora or Unix variants.
How to install HPX on Debian-based distributions¶
Install all packages for a minimal installation:
sudo apt install cmake libboost-all-dev hwloc libgoogle-perftools-dev
For building the documentation you will need to further install the following:
sudo apt install doxygen python-pip pip install --user sphinx sphinx_rtd_theme breathe
or the following if you prefer to get Python packages from the Debian repositories:
sudo apt install doxygen python-sphinx python-sphinx-rtd-theme python-breathe
The rest of the installation steps are same as provided with Fedora or Unix variants.
CMake toolchains shipped with HPX¶
In order to compile HPX for various platforms, we provide a variety of toolchain
files that take care of setting up various CMake variables like compilers etc.
They are located in the cmake/toolchains
directory:
To use them pass the -DCMAKE_TOOLCHAIN_FILE=<toolchain>
argument to the
cmake invocation.
ARM-gcc¶
# Copyright (c) 2015 Thomas Heller
#
# Distributed under the Boost Software License, Version 1.0. (See accompanying
# file LICENSE_1_0.txt or copy at http://www.boost.org/LICENSE_1_0.txt)
set(CMAKE_SYSTEM_NAME Linux)
set(CMAKE_CROSSCOMPILING ON)
# Set the gcc Compiler
set(CMAKE_CXX_COMPILER arm-linux-gnueabihf-g++-4.8)
set(CMAKE_C_COMPILER arm-linux-gnueabihf-gcc-4.8)
set(HPX_WITH_GENERIC_CONTEXT_COROUTINES ON CACHE BOOL "enable generic coroutines")
set(CMAKE_FIND_ROOT_PATH_MODE_PROGRAM NEVER)
set(CMAKE_FIND_ROOT_PATH_MODE_LIBRARY ONLY)
set(CMAKE_FIND_ROOT_PATH_MODE_INCLUDE ONLY)
set(CMAKE_FIND_ROOT_PATH_MODE_PACKAGE ONLY)
BGION-gcc¶
# Copyright (c) 2014 John Biddiscombe
#
# Distributed under the Boost Software License, Version 1.0. (See accompanying
# file LICENSE_1_0.txt or copy at http://www.boost.org/LICENSE_1_0.txt)
# This is the default toolchain file to be used with CNK on a BlueGene/Q. It sets
# the appropriate compile flags and compiler such that HPX will compile.
# Note that you still need to provide Boost, hwloc and other utility libraries
# like a custom allocator yourself.
#
# Usage : cmake -DCMAKE_TOOLCHAIN_FILE=~/src/hpx/cmake/toolchains/BGION-gcc.cmake ~/src/hpx
#
set(CMAKE_SYSTEM_NAME Linux)
# Set the gcc Compiler
set(CMAKE_CXX_COMPILER g++)
set(CMAKE_C_COMPILER gcc)
#set(CMAKE_Fortran_COMPILER)
# Add flags we need for BGAS compilation
set(CMAKE_CXX_FLAGS_INIT
"-D__powerpc__ -D__bgion__ -I/gpfs/bbp.cscs.ch/home/biddisco/src/bgas/rdmahelper "
CACHE STRING "Initial compiler flags used to compile for BGAS"
)
# the V1R2M2 includes are necessary for some hardware specific features
#-DHPX_SMALL_STACK_SIZE=0x200000 -DHPX_MEDIUM_STACK_SIZE=0x200000 -DHPX_LARGE_STACK_SIZE=0x200000 -DHPX_HUGE_STACK_SIZE=0x200000
set(CMAKE_EXE_LINKER_FLAGS_INIT "-L/gpfs/bbp.cscs.ch/apps/bgas/tools/gcc/gcc-4.8.2/install/lib64 -latomic -lrt" CACHE STRING "BGAS flags")
set(CMAKE_C_FLAGS_INIT "-D__powerpc__ -I/gpfs/bbp.cscs.ch/home/biddisco/src/bgas/rdmahelper" CACHE STRING "BGAS flags")
# We do not perform cross compilation here ...
set(CMAKE_CROSSCOMPILING OFF)
# Set our platform name
set(HPX_PLATFORM "native")
# Disable generic coroutines (and use posix version)
set(HPX_WITH_GENERIC_CONTEXT_COROUTINES OFF CACHE BOOL "disable generic coroutines")
# BGAS nodes support ibverbs
set(HPX_WITH_PARCELPORT_IBVERBS ON CACHE BOOL "")
# Always disable the tcp parcelport as it is non-functional on the BGQ.
set(HPX_WITH_PARCELPORT_TCP ON CACHE BOOL "")
# Always enable the tcp parcelport as it is currently the only way to communicate on the BGQ.
set(HPX_WITH_PARCELPORT_MPI ON CACHE BOOL "")
# We have a bunch of cores on the A2 processor ...
set(HPX_WITH_MAX_CPU_COUNT "64" CACHE STRING "")
# We have no custom malloc yet
if(NOT DEFINED HPX_WITH_MALLOC)
set(HPX_WITH_MALLOC "system" CACHE STRING "")
endif()
set(HPX_HIDDEN_VISIBILITY OFF CACHE BOOL "")
#
# Convenience setup for jb @ bbpbg2.cscs.ch
#
set(BOOST_ROOT "/gpfs/bbp.cscs.ch/home/biddisco/apps/gcc-4.8.2/boost_1_56_0")
set(HWLOC_ROOT "/gpfs/bbp.cscs.ch/home/biddisco/apps/gcc-4.8.2/hwloc-1.8.1")
set(CMAKE_BUILD_TYPE "Debug" CACHE STRING "Default build")
#
# Testing flags
#
set(BUILD_TESTING ON CACHE BOOL "Testing enabled by default")
set(HPX_WITH_TESTS ON CACHE BOOL "Testing enabled by default")
set(HPX_WITH_TESTS_BENCHMARKS ON CACHE BOOL "Testing enabled by default")
set(HPX_WITH_TESTS_REGRESSIONS ON CACHE BOOL "Testing enabled by default")
set(HPX_WITH_TESTS_UNIT ON CACHE BOOL "Testing enabled by default")
set(HPX_WITH_TESTS_EXAMPLES ON CACHE BOOL "Testing enabled by default")
set(HPX_WITH_TESTS_EXTERNAL_BUILD OFF CACHE BOOL "Turn off build of cmake build tests")
set(DART_TESTING_TIMEOUT 45 CACHE STRING "Life is too short")
# HPX_WITH_STATIC_LINKING
BGQ¶
# Copyright (c) 2014 Thomas Heller
#
# Distributed under the Boost Software License, Version 1.0. (See accompanying
# file LICENSE_1_0.txt or copy at http://www.boost.org/LICENSE_1_0.txt)
#
# This is the default toolchain file to be used with CNK on a BlueGene/Q. It sets
# the appropriate compile flags and compiler such that HPX will compile.
# Note that you still need to provide Boost, hwloc and other utility libraries
# like a custom allocator yourself.
#
set(CMAKE_SYSTEM_NAME Linux)
# Set the Intel Compiler
set(CMAKE_CXX_COMPILER bgclang++11)
set(CMAKE_C_COMPILER bgclang)
#set(CMAKE_Fortran_COMPILER)
set(MPI_CXX_COMPILER mpiclang++11)
set(MPI_C_COMPILER mpiclang)
#set(MPI_Fortran_COMPILER)
set(CMAKE_C_FLAGS_INIT "" CACHE STRING "")
set(CMAKE_C_COMPILE_OBJECT "<CMAKE_C_COMPILER> -fPIC <DEFINES> <FLAGS> -o <OBJECT> -c <SOURCE>" CACHE STRING "")
set(CMAKE_C_LINK_EXECUTABLE "<CMAKE_C_COMPILER> -fPIC -dynamic <FLAGS> <CMAKE_C_LINK_FLAGS> <LINK_FLAGS> <OBJECTS> -o <TARGET> <LINK_LIBRARIES>" CACHE STRING "")
set(CMAKE_C_CREATE_SHARED_LIBRARY "<CMAKE_C_COMPILER> -fPIC -shared <CMAKE_SHARED_LIBRARY_CXX_FLAGS> <LANGUAGE_COMPILE_FLAGS> <LINK_FLAGS> <CMAKE_SHARED_LIBRARY_CREATE_CXX_FLAGS> <SONAME_FLAG><TARGET_SONAME> -o <TARGET> <OBJECTS> <LINK_LIBRARIES> " CACHE STRING "")
set(CMAKE_CXX_FLAGS_INIT "" CACHE STRING "")
set(CMAKE_CXX_COMPILE_OBJECT "<CMAKE_CXX_COMPILER> -fPIC <DEFINES> <FLAGS> -o <OBJECT> -c <SOURCE>" CACHE STRING "")
set(CMAKE_CXX_LINK_EXECUTABLE "<CMAKE_CXX_COMPILER> -fPIC -dynamic <FLAGS> <CMAKE_CXX_LINK_FLAGS> <LINK_FLAGS> <OBJECTS> -o <TARGET> <LINK_LIBRARIES>" CACHE STRING "")
set(CMAKE_CXX_CREATE_SHARED_LIBRARY "<CMAKE_CXX_COMPILER> -fPIC -shared <CMAKE_SHARED_LIBRARY_CXX_FLAGS> <LANGUAGE_COMPILE_FLAGS> <LINK_FLAGS> <CMAKE_SHARED_LIBRARY_CREATE_CXX_FLAGS> <SONAME_FLAG><TARGET_SONAME> -o <TARGET> <OBJECTS> <LINK_LIBRARIES>" CACHE STRING "")
set(CMAKE_Fortran_FLAGS_INIT "" CACHE STRING "")
set(CMAKE_Fortran_COMPILE_OBJECT "<CMAKE_Fortran_COMPILER> -fPIC <DEFINES> <FLAGS> -o <OBJECT> -c <SOURCE>" CACHE STRING "")
set(CMAKE_Fortran_LINK_EXECUTABLE "<CMAKE_Fortran_COMPILER> -fPIC -dynamic <FLAGS> <CMAKE_Fortran_LINK_FLAGS> <LINK_FLAGS> <OBJECTS> -o <TARGET> <LINK_LIBRARIES>")
set(CMAKE_Fortran_CREATE_SHARED_LIBRARY "<CMAKE_Fortran_COMPILER> -fPIC -shared <CMAKE_SHARED_LIBRARY_Fortran_FLAGS> <LANGUAGE_COMPILE_FLAGS> <LINK_FLAGS> <CMAKE_SHARED_LIBRARY_CREATE_Fortran_FLAGS> <SONAME_FLAG><TARGET_SONAME> -o <TARGET> <OBJECTS> <LINK_LIBRARIES> " CACHE STRING "")
# Disable searches in the default system paths. We are cross compiling after all
# and cmake might pick up wrong libraries that way
set(CMAKE_FIND_ROOT_PATH_MODE_PROGRAM BOTH)
set(CMAKE_FIND_ROOT_PATH_MODE_LIBRARY ONLY)
set(CMAKE_FIND_ROOT_PATH_MODE_INCLUDE ONLY)
set(CMAKE_FIND_ROOT_PATH_MODE_PACKAGE ONLY)
# We do a cross compilation here ...
set(CMAKE_CROSSCOMPILING ON)
# Set our platform name
set(HPX_PLATFORM "BlueGeneQ")
# Always disable the ibverbs parcelport as it is non-functional on the BGQ.
set(HPX_WITH_IBVERBS_PARCELPORT OFF)
# Always disable the tcp parcelport as it is non-functional on the BGQ.
set(HPX_WITH_TCP_PARCELPORT OFF)
# Always enable the tcp parcelport as it is currently the only way to communicate on the BGQ.
set(HPX_WITH_MPI_PARCELPORT ON)
# We have a bunch of cores on the BGQ ...
set(HPX_WITH_MAX_CPU_COUNT "64")
# We default to tbbmalloc as our allocator on the MIC
if(NOT DEFINED HPX_WITH_MALLOC)
set(HPX_WITH_MALLOC "system" CACHE STRING "")
endif()
Cray¶
# Copyright (c) 2014 Thomas Heller
#
# Distributed under the Boost Software License, Version 1.0. (See accompanying
# file LICENSE_1_0.txt or copy at http://www.boost.org/LICENSE_1_0.txt)
#
# This is the default toolchain file to be used with Intel Xeon PHIs. It sets
# the appropriate compile flags and compiler such that HPX will compile.
# Note that you still need to provide Boost, hwloc and other utility libraries
# like a custom allocator yourself.
#
#set(CMAKE_SYSTEM_NAME Cray-CNK-Intel)
if(HPX_WITH_STATIC_LINKING)
set_property(GLOBAL PROPERTY TARGET_SUPPORTS_SHARED_LIBS FALSE)
else()
endif()
# Set the Cray Compiler Wrapper
set(CMAKE_CXX_COMPILER CC)
set(CMAKE_C_COMPILER cc)
set(CMAKE_Fortran_COMPILER ftn)
if (CMAKE_VERSION VERSION_GREATER 3.3.9)
set(__includes "<INCLUDES>")
endif()
set(CMAKE_C_FLAGS_INIT "" CACHE STRING "")
set(CMAKE_SHARED_LIBRARY_C_FLAGS "-fPIC -shared" CACHE STRING "")
set(CMAKE_SHARED_LIBRARY_CREATE_C_FLAGS "-fPIC -shared" CACHE STRING "")
set(CMAKE_C_COMPILE_OBJECT "<CMAKE_C_COMPILER> -shared -fPIC <DEFINES> ${__includes} <FLAGS> -o <OBJECT> -c <SOURCE>" CACHE STRING "")
set(CMAKE_C_LINK_EXECUTABLE "<CMAKE_C_COMPILER> -fPIC -dynamic <FLAGS> <CMAKE_C_LINK_FLAGS> <LINK_FLAGS> <OBJECTS> -o <TARGET> <LINK_LIBRARIES>" CACHE STRING "")
set(CMAKE_C_CREATE_SHARED_LIBRARY "<CMAKE_C_COMPILER> -fPIC -shared <CMAKE_SHARED_LIBRARY_CXX_FLAGS> <LANGUAGE_COMPILE_FLAGS> <LINK_FLAGS> <CMAKE_SHARED_LIBRARY_CREATE_CXX_FLAGS> <SONAME_FLAG><TARGET_SONAME> -o <TARGET> <OBJECTS> <LINK_LIBRARIES> " CACHE STRING "")
set(CMAKE_CXX_FLAGS_INIT "" CACHE STRING "")
set(CMAKE_SHARED_LIBRARY_CXX_FLAGS "-fPIC -shared" CACHE STRING "")
set(CMAKE_SHARED_LIBRARY_CREATE_CXX_FLAGS "-fPIC -shared" CACHE STRING "")
set(CMAKE_SHARED_LIBRARY_CREATE_CXX_FLAGS "-fPIC -shared" CACHE STRING "")
set(CMAKE_CXX_COMPILE_OBJECT "<CMAKE_CXX_COMPILER> -shared -fPIC <DEFINES> ${__includes} <FLAGS> -o <OBJECT> -c <SOURCE>" CACHE STRING "")
set(CMAKE_CXX_LINK_EXECUTABLE "<CMAKE_CXX_COMPILER> -fPIC -dynamic <FLAGS> <CMAKE_CXX_LINK_FLAGS> <LINK_FLAGS> <OBJECTS> -o <TARGET> <LINK_LIBRARIES>" CACHE STRING "")
set(CMAKE_CXX_CREATE_SHARED_LIBRARY "<CMAKE_CXX_COMPILER> -fPIC -shared <CMAKE_SHARED_LIBRARY_CXX_FLAGS> <LANGUAGE_COMPILE_FLAGS> <LINK_FLAGS> <CMAKE_SHARED_LIBRARY_CREATE_CXX_FLAGS> <SONAME_FLAG><TARGET_SONAME> -o <TARGET> <OBJECTS> <LINK_LIBRARIES>" CACHE STRING "")
set(CMAKE_Fortran_FLAGS_INIT "" CACHE STRING "")
set(CMAKE_SHARED_LIBRARY_Fortran_FLAGS "-fPIC" CACHE STRING "")
set(CMAKE_SHARED_LIBRARY_CREATE_Fortran_FLAGS "-shared" CACHE STRING "")
set(CMAKE_Fortran_COMPILE_OBJECT "<CMAKE_Fortran_COMPILER> -shared -fPIC <DEFINES> ${__includes} <FLAGS> -o <OBJECT> -c <SOURCE>" CACHE STRING "")
set(CMAKE_Fortran_LINK_EXECUTABLE "<CMAKE_Fortran_COMPILER> -fPIC -dynamic <FLAGS> <CMAKE_Fortran_LINK_FLAGS> <LINK_FLAGS> <OBJECTS> -o <TARGET> <LINK_LIBRARIES>")
set(CMAKE_Fortran_CREATE_SHARED_LIBRARY "<CMAKE_Fortran_COMPILER> -fPIC -shared <CMAKE_SHARED_LIBRARY_Fortran_FLAGS> <LANGUAGE_COMPILE_FLAGS> <LINK_FLAGS> <CMAKE_SHARED_LIBRARY_CREATE_Fortran_FLAGS> <SONAME_FLAG><TARGET_SONAME> -o <TARGET> <OBJECTS> <LINK_LIBRARIES> " CACHE STRING "")
# Disable searches in the default system paths. We are cross compiling after all
# and cmake might pick up wrong libraries that way
set(CMAKE_FIND_ROOT_PATH_MODE_PROGRAM BOTH)
set(CMAKE_FIND_ROOT_PATH_MODE_LIBRARY ONLY)
set(CMAKE_FIND_ROOT_PATH_MODE_INCLUDE ONLY)
set(CMAKE_FIND_ROOT_PATH_MODE_PACKAGE ONLY)
set(HPX_WITH_PARCELPORT_TCP ON CACHE BOOL "")
set(HPX_WITH_PARCELPORT_MPI ON CACHE BOOL "")
set(HPX_WITH_PARCELPORT_MPI_MULTITHREADED OFF CACHE BOOL "")
set(HPX_WITH_PARCELPORT_LIBFABRIC ON CACHE BOOL "")
set(HPX_PARCELPORT_LIBFABRIC_PROVIDER "gni" CACHE STRING
"See libfabric docs for details, gni,verbs,psm2 etc etc")
set(HPX_PARCELPORT_LIBFABRIC_THROTTLE_SENDS "256" CACHE STRING
"Max number of messages in flight at once")
set(HPX_PARCELPORT_LIBFABRIC_WITH_DEV_MODE OFF CACHE BOOL
"Custom libfabric logging flag")
set(HPX_PARCELPORT_LIBFABRIC_WITH_LOGGING OFF CACHE BOOL
"Libfabric parcelport logging on/off flag")
set(HPX_WITH_ZERO_COPY_SERIALIZATION_THRESHOLD "4096" CACHE STRING
"The threshhold in bytes to when perform zero copy optimizations (default: 128)")
# We do a cross compilation here ...
set(CMAKE_CROSSCOMPILING ON CACHE BOOL "")
CrayKNL¶
# Copyright (c) 2014 Thomas Heller
#
# Distributed under the Boost Software License, Version 1.0. (See accompanying
# file LICENSE_1_0.txt or copy at http://www.boost.org/LICENSE_1_0.txt)
#
# This is the default toolchain file to be used with Intel Xeon PHIs. It sets
# the appropriate compile flags and compiler such that HPX will compile.
# Note that you still need to provide Boost, hwloc and other utility libraries
# like a custom allocator yourself.
#
if(HPX_WITH_STATIC_LINKING)
set_property(GLOBAL PROPERTY TARGET_SUPPORTS_SHARED_LIBS FALSE)
else()
endif()
# Set the Cray Compiler Wrapper
set(CMAKE_CXX_COMPILER CC)
set(CMAKE_C_COMPILER cc)
set(CMAKE_Fortran_COMPILER ftn)
if (CMAKE_VERSION VERSION_GREATER 3.3.9)
set(__includes "<INCLUDES>")
endif()
set(CMAKE_C_FLAGS_INIT "" CACHE STRING "")
set(CMAKE_SHARED_LIBRARY_C_FLAGS "-fPIC -shared" CACHE STRING "")
set(CMAKE_SHARED_LIBRARY_CREATE_C_FLAGS "-fPIC -shared" CACHE STRING "")
set(CMAKE_C_COMPILE_OBJECT "<CMAKE_C_COMPILER> -shared -fPIC <DEFINES> ${__includes} <FLAGS> -o <OBJECT> -c <SOURCE>" CACHE STRING "")
set(CMAKE_C_LINK_EXECUTABLE "<CMAKE_C_COMPILER> -fPIC <FLAGS> <CMAKE_C_LINK_FLAGS> <LINK_FLAGS> <OBJECTS> -o <TARGET> <LINK_LIBRARIES>" CACHE STRING "")
set(CMAKE_C_CREATE_SHARED_LIBRARY "<CMAKE_C_COMPILER> -fPIC -shared <CMAKE_SHARED_LIBRARY_CXX_FLAGS> <LANGUAGE_COMPILE_FLAGS> <LINK_FLAGS> <CMAKE_SHARED_LIBRARY_CREATE_CXX_FLAGS> <SONAME_FLAG><TARGET_SONAME> -o <TARGET> <OBJECTS> <LINK_LIBRARIES> " CACHE STRING "")
#
set(CMAKE_CXX_FLAGS_INIT "" CACHE STRING "")
set(CMAKE_SHARED_LIBRARY_CXX_FLAGS "-fPIC -shared" CACHE STRING "")
set(CMAKE_SHARED_LIBRARY_CREATE_CXX_FLAGS "-fPIC -shared" CACHE STRING "")
set(CMAKE_SHARED_LIBRARY_CREATE_CXX_FLAGS "-fPIC -shared" CACHE STRING "")
set(CMAKE_CXX_COMPILE_OBJECT "<CMAKE_CXX_COMPILER> -shared -fPIC <DEFINES> ${__includes} <FLAGS> -o <OBJECT> -c <SOURCE>" CACHE STRING "")
set(CMAKE_CXX_LINK_EXECUTABLE "<CMAKE_CXX_COMPILER> -fPIC -dynamic <FLAGS> <CMAKE_CXX_LINK_FLAGS> <LINK_FLAGS> <OBJECTS> -o <TARGET> <LINK_LIBRARIES>" CACHE STRING "")
set(CMAKE_CXX_CREATE_SHARED_LIBRARY "<CMAKE_CXX_COMPILER> -fPIC -shared <CMAKE_SHARED_LIBRARY_CXX_FLAGS> <LANGUAGE_COMPILE_FLAGS> <LINK_FLAGS> <CMAKE_SHARED_LIBRARY_CREATE_CXX_FLAGS> <SONAME_FLAG><TARGET_SONAME> -o <TARGET> <OBJECTS> <LINK_LIBRARIES>" CACHE STRING "")
#
set(CMAKE_Fortran_FLAGS_INIT "" CACHE STRING "")
set(CMAKE_SHARED_LIBRARY_Fortran_FLAGS "-fPIC" CACHE STRING "")
set(CMAKE_SHARED_LIBRARY_CREATE_Fortran_FLAGS "-shared" CACHE STRING "")
set(CMAKE_Fortran_COMPILE_OBJECT "<CMAKE_Fortran_COMPILER> -shared -fPIC <DEFINES> ${__includes} <FLAGS> -o <OBJECT> -c <SOURCE>" CACHE STRING "")
set(CMAKE_Fortran_LINK_EXECUTABLE "<CMAKE_Fortran_COMPILER> -fPIC <FLAGS> <CMAKE_Fortran_LINK_FLAGS> <LINK_FLAGS> <OBJECTS> -o <TARGET> <LINK_LIBRARIES>")
set(CMAKE_Fortran_CREATE_SHARED_LIBRARY "<CMAKE_Fortran_COMPILER> -fPIC -shared <CMAKE_SHARED_LIBRARY_Fortran_FLAGS> <LANGUAGE_COMPILE_FLAGS> <LINK_FLAGS> <CMAKE_SHARED_LIBRARY_CREATE_Fortran_FLAGS> <SONAME_FLAG><TARGET_SONAME> -o <TARGET> <OBJECTS> <LINK_LIBRARIES> " CACHE STRING "")
#
# Disable searches in the default system paths. We are cross compiling after all
# and cmake might pick up wrong libraries that way
set(CMAKE_FIND_ROOT_PATH_MODE_PROGRAM BOTH)
set(CMAKE_FIND_ROOT_PATH_MODE_LIBRARY ONLY)
set(CMAKE_FIND_ROOT_PATH_MODE_INCLUDE ONLY)
set(CMAKE_FIND_ROOT_PATH_MODE_PACKAGE ONLY)
set(HPX_WITH_PARCELPORT_TCP ON CACHE BOOL "")
set(HPX_WITH_PARCELPORT_MPI ON CACHE BOOL "")
set(HPX_WITH_PARCELPORT_MPI_MULTITHREADED OFF CACHE BOOL "")
set(HPX_WITH_PARCELPORT_LIBFABRIC ON CACHE BOOL "")
set(HPX_PARCELPORT_LIBFABRIC_PROVIDER "gni" CACHE STRING
"See libfabric docs for details, gni,verbs,psm2 etc etc")
set(HPX_PARCELPORT_LIBFABRIC_THROTTLE_SENDS "256" CACHE STRING
"Max number of messages in flight at once")
set(HPX_PARCELPORT_LIBFABRIC_WITH_DEV_MODE OFF CACHE BOOL
"Custom libfabric logging flag")
set(HPX_PARCELPORT_LIBFABRIC_WITH_LOGGING OFF CACHE BOOL
"Libfabric parcelport logging on/off flag")
set(HPX_WITH_ZERO_COPY_SERIALIZATION_THRESHOLD "4096" CACHE STRING
"The threshhold in bytes to when perform zero copy optimizations (default: 128)")
# Set the TBBMALLOC_PLATFORM correctly so that find_package(TBBMalloc) sets the
# right hints
set(TBBMALLOC_PLATFORM "mic-knl" CACHE STRING "")
# We have a bunch of cores on the MIC ... increase the default
set(HPX_WITH_MAX_CPU_COUNT "512" CACHE STRING "")
# We do a cross compilation here ...
set(CMAKE_CROSSCOMPILING ON CACHE BOOL "")
# RDTSCP is available on Xeon/Phis
set(HPX_WITH_RDTSCP ON CACHE BOOL "")
CrayKNLStatic¶
# Copyright (c) 2014-2017 Thomas Heller
# Copyright (c) 2017 Bryce Adelstein Lelbach
#
# Distributed under the Boost Software License, Version 1.0. (See accompanying
# file LICENSE_1_0.txt or copy at http://www.boost.org/LICENSE_1_0.txt)
set(HPX_WITH_STATIC_LINKING ON CACHE BOOL "")
set(HPX_WITH_STATIC_EXE_LINKING ON CACHE BOOL "")
set_property(GLOBAL PROPERTY TARGET_SUPPORTS_SHARED_LIBS FALSE)
# Set the Cray Compiler Wrapper
set(CMAKE_CXX_COMPILER CC)
set(CMAKE_C_COMPILER cc)
set(CMAKE_Fortran_COMPILER ftn)
if (CMAKE_VERSION VERSION_GREATER 3.3.9)
set(__includes "<INCLUDES>")
endif()
set(CMAKE_C_FLAGS_INIT "" CACHE STRING "")
set(CMAKE_C_COMPILE_OBJECT "<CMAKE_C_COMPILER> -static -fPIC <DEFINES> ${__includes} <FLAGS> -o <OBJECT> -c <SOURCE>" CACHE STRING "")
set(CMAKE_C_LINK_EXECUTABLE "<CMAKE_C_COMPILER> -fPIC <FLAGS> <CMAKE_C_LINK_FLAGS> <LINK_FLAGS> <OBJECTS> -o <TARGET> <LINK_LIBRARIES>" CACHE STRING "")
set(CMAKE_CXX_FLAGS_INIT "" CACHE STRING "")
set(CMAKE_CXX_COMPILE_OBJECT "<CMAKE_CXX_COMPILER> -static -fPIC <DEFINES> ${__includes} <FLAGS> -o <OBJECT> -c <SOURCE>" CACHE STRING "")
set(CMAKE_CXX_LINK_EXECUTABLE "<CMAKE_CXX_COMPILER> -fPIC <FLAGS> <CMAKE_CXX_LINK_FLAGS> <LINK_FLAGS> <OBJECTS> -o <TARGET> <LINK_LIBRARIES>" CACHE STRING "")
set(CMAKE_Fortran_FLAGS_INIT "" CACHE STRING "")
set(CMAKE_Fortran_COMPILE_OBJECT "<CMAKE_Fortran_COMPILER> -static -fPIC <DEFINES> ${__includes} <FLAGS> -o <OBJECT> -c <SOURCE>" CACHE STRING "")
set(CMAKE_Fortran_LINK_EXECUTABLE "<CMAKE_Fortran_COMPILER> -fPIC <FLAGS> <CMAKE_Fortran_LINK_FLAGS> <LINK_FLAGS> <OBJECTS> -o <TARGET> <LINK_LIBRARIES>")
# Disable searches in the default system paths. We are cross compiling after all
# and cmake might pick up wrong libraries that way
set(CMAKE_FIND_ROOT_PATH_MODE_PROGRAM BOTH)
set(CMAKE_FIND_ROOT_PATH_MODE_LIBRARY ONLY)
set(CMAKE_FIND_ROOT_PATH_MODE_INCLUDE ONLY)
set(CMAKE_FIND_ROOT_PATH_MODE_PACKAGE ONLY)
set(HPX_WITH_PARCELPORT_TCP ON CACHE BOOL "")
set(HPX_WITH_PARCELPORT_MPI ON CACHE BOOL "")
set(HPX_WITH_PARCELPORT_MPI_MULTITHREADED ON CACHE BOOL "")
set(HPX_WITH_PARCELPORT_LIBFABRIC ON CACHE BOOL "")
set(HPX_PARCELPORT_LIBFABRIC_PROVIDER "gni" CACHE STRING
"See libfabric docs for details, gni,verbs,psm2 etc etc")
set(HPX_PARCELPORT_LIBFABRIC_THROTTLE_SENDS "256" CACHE STRING
"Max number of messages in flight at once")
set(HPX_PARCELPORT_LIBFABRIC_WITH_DEV_MODE OFF CACHE BOOL
"Custom libfabric logging flag")
set(HPX_PARCELPORT_LIBFABRIC_WITH_LOGGING OFF CACHE BOOL
"Libfabric parcelport logging on/off flag")
set(HPX_WITH_ZERO_COPY_SERIALIZATION_THRESHOLD "4096" CACHE STRING
"The threshhold in bytes to when perform zero copy optimizations (default: 128)")
# Set the TBBMALLOC_PLATFORM correctly so that find_package(TBBMalloc) sets the
# right hints
set(TBBMALLOC_PLATFORM "mic-knl" CACHE STRING "")
# We have a bunch of cores on the MIC ... increase the default
set(HPX_WITH_MAX_CPU_COUNT "512" CACHE STRING "")
# We do a cross compilation here ...
set(CMAKE_CROSSCOMPILING ON CACHE BOOL "")
# RDTSCP is available on Xeon/Phis
set(HPX_WITH_RDTSCP ON CACHE BOOL "")
CrayStatic¶
# Copyright (c) 2014-2017 Thomas Heller
# Copyright (c) 2017 Bryce Adelstein Lelbach
#
# Distributed under the Boost Software License, Version 1.0. (See accompanying
# file LICENSE_1_0.txt or copy at http://www.boost.org/LICENSE_1_0.txt)
set(HPX_WITH_STATIC_LINKING ON CACHE BOOL "")
set(HPX_WITH_STATIC_EXE_LINKING ON CACHE BOOL "")
set_property(GLOBAL PROPERTY TARGET_SUPPORTS_SHARED_LIBS FALSE)
# Set the Cray Compiler Wrapper
set(CMAKE_CXX_COMPILER CC)
set(CMAKE_C_COMPILER cc)
set(CMAKE_Fortran_COMPILER ftn)
if (CMAKE_VERSION VERSION_GREATER 3.3.9)
set(__includes "<INCLUDES>")
endif()
set(CMAKE_C_FLAGS_INIT "" CACHE STRING "")
set(CMAKE_C_COMPILE_OBJECT "<CMAKE_C_COMPILER> -static -fPIC <DEFINES> ${__includes} <FLAGS> -o <OBJECT> -c <SOURCE>" CACHE STRING "")
set(CMAKE_C_LINK_EXECUTABLE "<CMAKE_C_COMPILER> -fPIC <FLAGS> <CMAKE_C_LINK_FLAGS> <LINK_FLAGS> <OBJECTS> -o <TARGET> <LINK_LIBRARIES>" CACHE STRING "")
set(CMAKE_CXX_FLAGS_INIT "" CACHE STRING "")
set(CMAKE_CXX_COMPILE_OBJECT "<CMAKE_CXX_COMPILER> -static -fPIC <DEFINES> ${__includes} <FLAGS> -o <OBJECT> -c <SOURCE>" CACHE STRING "")
set(CMAKE_CXX_LINK_EXECUTABLE "<CMAKE_CXX_COMPILER> -fPIC <FLAGS> <CMAKE_CXX_LINK_FLAGS> <LINK_FLAGS> <OBJECTS> -o <TARGET> <LINK_LIBRARIES>" CACHE STRING "")
set(CMAKE_Fortran_FLAGS_INIT "" CACHE STRING "")
set(CMAKE_Fortran_COMPILE_OBJECT "<CMAKE_Fortran_COMPILER> -static -fPIC <DEFINES> ${__includes} <FLAGS> -o <OBJECT> -c <SOURCE>" CACHE STRING "")
set(CMAKE_Fortran_LINK_EXECUTABLE "<CMAKE_Fortran_COMPILER> -fPIC <FLAGS> <CMAKE_Fortran_LINK_FLAGS> <LINK_FLAGS> <OBJECTS> -o <TARGET> <LINK_LIBRARIES>")
# Disable searches in the default system paths. We are cross compiling after all
# and cmake might pick up wrong libraries that way
set(CMAKE_FIND_ROOT_PATH_MODE_PROGRAM BOTH)
set(CMAKE_FIND_ROOT_PATH_MODE_LIBRARY ONLY)
set(CMAKE_FIND_ROOT_PATH_MODE_INCLUDE ONLY)
set(CMAKE_FIND_ROOT_PATH_MODE_PACKAGE ONLY)
# We do a cross compilation here ...
set(CMAKE_CROSSCOMPILING ON CACHE BOOL "")
# RDTSCP is available on Xeon/Phis
set(HPX_WITH_RDTSCP ON CACHE BOOL "")
set(HPX_WITH_PARCELPORT_TCP ON CACHE BOOL "")
set(HPX_WITH_PARCELPORT_MPI ON CACHE BOOL "")
set(HPX_WITH_PARCELPORT_MPI_MULTITHREADED ON CACHE BOOL "")
set(HPX_WITH_PARCELPORT_LIBFABRIC ON CACHE BOOL "")
set(HPX_PARCELPORT_LIBFABRIC_PROVIDER "gni" CACHE STRING
"See libfabric docs for details, gni,verbs,psm2 etc etc")
set(HPX_PARCELPORT_LIBFABRIC_THROTTLE_SENDS "256" CACHE STRING
"Max number of messages in flight at once")
set(HPX_PARCELPORT_LIBFABRIC_WITH_DEV_MODE OFF CACHE BOOL
"Custom libfabric logging flag")
set(HPX_PARCELPORT_LIBFABRIC_WITH_LOGGING OFF CACHE BOOL
"Libfabric parcelport logging on/off flag")
set(HPX_WITH_ZERO_COPY_SERIALIZATION_THRESHOLD "4096" CACHE STRING
"The threshhold in bytes to when perform zero copy optimizations (default: 128)")
XeonPhi¶
# Copyright (c) 2014 Thomas Heller
#
# Distributed under the Boost Software License, Version 1.0. (See accompanying
# file LICENSE_1_0.txt or copy at http://www.boost.org/LICENSE_1_0.txt)
#
# This is the default toolchain file to be used with Intel Xeon PHIs. It sets
# the appropriate compile flags and compiler such that HPX will compile.
# Note that you still need to provide Boost, hwloc and other utility libraries
# like a custom allocator yourself.
#
set(CMAKE_SYSTEM_NAME Linux)
# Set the Intel Compiler
set(CMAKE_CXX_COMPILER icpc)
set(CMAKE_C_COMPILER icc)
set(CMAKE_Fortran_COMPILER ifort)
# Add the -mmic compile flag such that everything will be compiled for the correct
# platform
set(CMAKE_CXX_FLAGS_INIT "-mmic" CACHE STRING "Initial compiler flags used to compile for the Xeon Phi")
set(CMAKE_C_FLAGS_INIT "-mmic" CACHE STRING "Initial compiler flags used to compile for the Xeon Phi")
set(CMAKE_Fortran_FLAGS_INIT "-mmic" CACHE STRING "Initial compiler flags used to compile for the Xeon Phi")
# Disable searches in the default system paths. We are cross compiling after all
# and cmake might pick up wrong libraries that way
set(CMAKE_FIND_ROOT_PATH_MODE_PROGRAM BOTH)
set(CMAKE_FIND_ROOT_PATH_MODE_LIBRARY ONLY)
set(CMAKE_FIND_ROOT_PATH_MODE_INCLUDE ONLY)
set(CMAKE_FIND_ROOT_PATH_MODE_PACKAGE ONLY)
# We do a cross compilation here ...
set(CMAKE_CROSSCOMPILING ON)
# Set our platform name
set(HPX_PLATFORM "XeonPhi")
# Always disable the ibverbs parcelport as it is non-functional on the BGQ.
set(HPX_WITH_PARCELPORT_IBVERBS OFF CACHE BOOL "Enable the ibverbs based parcelport. This is currently an experimental feature")
# We have a bunch of cores on the MIC ... increase the default
set(HPX_WITH_MAX_CPU_COUNT "256" CACHE STRING "")
# We default to tbbmalloc as our allocator on the MIC
if(NOT DEFINED HPX_WITH_MALLOC)
set(HPX_WITH_MALLOC "tbbmalloc" CACHE STRING "")
endif()
# Set the TBBMALLOC_PLATFORM correctly so that find_package(TBBMalloc) sets the
# right hints
set(TBBMALLOC_PLATFORM "mic" CACHE STRING "")
set(HPX_HIDDEN_VISIBILITY OFF CACHE BOOL "Use -fvisibility=hidden for builds on platforms which support it")
# RDTSC is available on Xeon/Phis
set(HPX_WITH_RDTSC ON CACHE BOOL "")
CMake variables used to configure HPX¶
In order to configure HPX, you can set a variety of options to allow cmake to generate your specific makefiles/project files.
Variables that influence how HPX is built¶
The options are split into these categories:
Generic options¶
HPX_WITH_AUTOMATIC_SERIALIZATION_REGISTRATION:BOOL
HPX_WITH_BENCHMARK_SCRIPTS_PATH:PATH
HPX_WITH_BUILD_BINARY_PACKAGE:BOOL
HPX_WITH_COMPILER_WARNINGS:BOOL
HPX_WITH_COMPILER_WARNINGS_AS_ERRORS:BOOL
HPX_WITH_COMPRESSION_BZIP2:BOOL
HPX_WITH_COMPRESSION_SNAPPY:BOOL
HPX_WITH_COMPRESSION_ZLIB:BOOL
HPX_WITH_CUDA:BOOL
HPX_WITH_CUDA_CLANG:BOOL
HPX_WITH_CXX14_RETURN_TYPE_DEDUCTION:BOOL
HPX_WITH_DATAPAR_BOOST_SIMD:BOOL
HPX_WITH_DATAPAR_VC:BOOL
HPX_WITH_DEPRECATION_WARNINGS:BOOL
HPX_WITH_DISABLED_SIGNAL_EXCEPTION_HANDLERS:BOOL
HPX_WITH_DYNAMIC_HPX_MAIN:BOOL
HPX_WITH_FAULT_TOLERANCE:BOOL
HPX_WITH_FORTRAN:BOOL
HPX_WITH_FULL_RPATH:BOOL
HPX_WITH_GCC_VERSION_CHECK:BOOL
HPX_WITH_GENERIC_CONTEXT_COROUTINES:BOOL
HPX_WITH_HCC:BOOL
HPX_WITH_HIDDEN_VISIBILITY:BOOL
HPX_WITH_INCLUSIVE_SCAN_COMPATIBILITY:BOOL
HPX_WITH_LOGGING:BOOL
HPX_WITH_MALLOC:STRING
HPX_WITH_NATIVE_TLS:BOOL
HPX_WITH_NICE_THREADLEVEL:BOOL
HPX_WITH_PARCEL_COALESCING:BOOL
HPX_WITH_RUN_MAIN_EVERYWHERE:BOOL
HPX_WITH_STACKOVERFLOW_DETECTION:BOOL
HPX_WITH_STATIC_LINKING:BOOL
HPX_WITH_SYCL:BOOL
HPX_WITH_THREAD_COMPATIBILITY:BOOL
HPX_WITH_UNWRAPPED_COMPATIBILITY:BOOL
HPX_WITH_VIM_YCM:BOOL
HPX_WITH_ZERO_COPY_SERIALIZATION_THRESHOLD:STRING
-
HPX_WITH_AUTOMATIC_SERIALIZATION_REGISTRATION:BOOL
¶
Use automatic serialization registration for actions and functions. This affects compatibility between HPX applications compiled with different compilers (default ON)
-
HPX_WITH_BENCHMARK_SCRIPTS_PATH:PATH
¶
Directory to place batch scripts in
-
HPX_WITH_BUILD_BINARY_PACKAGE:BOOL
¶
Build HPX on the build infrastructure on any LINUX distribution (default: OFF).
-
HPX_WITH_COMPILER_WARNINGS:BOOL
¶
Enable compiler warnings (default: ON)
-
HPX_WITH_COMPILER_WARNINGS_AS_ERRORS:BOOL
¶
Turn compiler warnings into errors (default: OFF)
-
HPX_WITH_COMPRESSION_BZIP2:BOOL
¶
Enable bzip2 compression for parcel data (default: OFF).
-
HPX_WITH_COMPRESSION_SNAPPY:BOOL
¶
Enable snappy compression for parcel data (default: OFF).
-
HPX_WITH_COMPRESSION_ZLIB:BOOL
¶
Enable zlib compression for parcel data (default: OFF).
-
HPX_WITH_CUDA:BOOL
¶
Enable CUDA support (default: OFF)
-
HPX_WITH_CUDA_CLANG:BOOL
¶
Use clang to compile CUDA code (default: OFF)
-
HPX_WITH_CXX14_RETURN_TYPE_DEDUCTION:BOOL
¶
Enable the use of auto as a return value in some places. Overriding this flag is only necessary if the C++ compiler is not standard compliant, e.g. nvcc.
-
HPX_WITH_DATAPAR_BOOST_SIMD:BOOL
¶
Enable data parallel algorithm support using the external Boost.SIMD library (default: OFF)
-
HPX_WITH_DATAPAR_VC:BOOL
¶
Enable data parallel algorithm support using the external Vc library (default: OFF)
-
HPX_WITH_DEPRECATION_WARNINGS:BOOL
¶
Enable warnings for deprecated facilities. (default: ON)
-
HPX_WITH_DISABLED_SIGNAL_EXCEPTION_HANDLERS:BOOL
¶
Disables the mechanism that produces debug output for caught signals and unhandled exceptions (default: OFF)
-
HPX_WITH_DYNAMIC_HPX_MAIN:BOOL
¶
Enable dynamic overload of system
main()
(Linux only, default: ON)
-
HPX_WITH_FAULT_TOLERANCE:BOOL
¶
Build HPX to tolerate failures of nodes, i.e. ignore errors in active communication channels (default: OFF)
-
HPX_WITH_FORTRAN:BOOL
¶
Enable or disable the compilation of Fortran examples using HPX
-
HPX_WITH_FULL_RPATH:BOOL
¶
Build and link HPX libraries and executables with full RPATHs (default: ON)
-
HPX_WITH_GCC_VERSION_CHECK:BOOL
¶
Don’t ignore version reported by gcc (default: ON)
-
HPX_WITH_GENERIC_CONTEXT_COROUTINES:BOOL
¶
Use Boost.Context as the underlying coroutines context switch implementation.
-
HPX_WITH_HCC:BOOL
¶
Enable hcc support (default: OFF)
Use -fvisibility=hidden for builds on platforms which support it (default OFF)
-
HPX_WITH_INCLUSIVE_SCAN_COMPATIBILITY:BOOL
¶
Enable old overloads for inclusive_scan (default: OFF)
-
HPX_WITH_LOGGING:BOOL
¶
Build HPX with logging enabled (default: ON).
-
HPX_WITH_MALLOC:STRING
¶
Define which allocator should be linked in. Options are: system, tcmalloc, jemalloc, tbbmalloc, and custom (default is: tcmalloc)
-
HPX_WITH_NATIVE_TLS:BOOL
¶
Use native TLS support if available (default: ON)
-
HPX_WITH_NICE_THREADLEVEL:BOOL
¶
Set HPX worker threads to have high NICE level (may impact performance) (default: OFF)
-
HPX_WITH_PARCEL_COALESCING:BOOL
¶
Enable the parcel coalescing plugin (default: ON).
-
HPX_WITH_RUN_MAIN_EVERYWHERE:BOOL
¶
Run hpx_main by default on all localities (default: OFF).
-
HPX_WITH_STACKOVERFLOW_DETECTION:BOOL
¶
Enable stackoverflow detection for HPX threads/coroutines. (default: OFF, debug: ON)
-
HPX_WITH_STATIC_LINKING:BOOL
¶
Compile HPX statically linked libraries (Default: OFF)
-
HPX_WITH_SYCL:BOOL
¶
Enable sycl support (default: OFF)
-
HPX_WITH_THREAD_COMPATIBILITY:BOOL
¶
Use a compatibility implementation of std::thread, i.e. fall back to Boost.Thread (default: OFF)
-
HPX_WITH_UNWRAPPED_COMPATIBILITY:BOOL
¶
Enable the deprecated unwrapped function (default: OFF)
-
HPX_WITH_VIM_YCM:BOOL
¶
Generate HPX completion file for VIM YouCompleteMe plugin
-
HPX_WITH_ZERO_COPY_SERIALIZATION_THRESHOLD:STRING
¶
The threshhold in bytes to when perform zero copy optimizations (default: 128)
Build Targets options¶
HPX_WITH_COMPILE_ONLY_TESTS:BOOL
HPX_WITH_DEFAULT_TARGETS:BOOL
HPX_WITH_DOCUMENTATION:BOOL
HPX_WITH_DOCUMENTATION_OUTPUT_FORMATS:STRING
HPX_WITH_EXAMPLES:BOOL
HPX_WITH_EXAMPLES_HDF5:BOOL
HPX_WITH_EXAMPLES_OPENMP:BOOL
HPX_WITH_EXAMPLES_QT4:BOOL
HPX_WITH_EXAMPLES_QTHREADS:BOOL
HPX_WITH_EXAMPLES_TBB:BOOL
HPX_WITH_EXECUTABLE_PREFIX:STRING
HPX_WITH_FAIL_COMPILE_TESTS:BOOL
HPX_WITH_IO_COUNTERS:BOOL
HPX_WITH_PSEUDO_DEPENDENCIES:BOOL
HPX_WITH_TESTS:BOOL
HPX_WITH_TESTS_BENCHMARKS:BOOL
HPX_WITH_TESTS_EXAMPLES:BOOL
HPX_WITH_TESTS_EXTERNAL_BUILD:BOOL
HPX_WITH_TESTS_HEADERS:BOOL
HPX_WITH_TESTS_REGRESSIONS:BOOL
HPX_WITH_TESTS_UNIT:BOOL
HPX_WITH_TOOLS:BOOL
-
HPX_WITH_COMPILE_ONLY_TESTS:BOOL
¶
Create build system support for compile time only HPX tests (default ON)
-
HPX_WITH_DEFAULT_TARGETS:BOOL
¶
Associate the core HPX library with the default build target (default: ON).
-
HPX_WITH_DOCUMENTATION:BOOL
¶
Build the HPX documentation (default OFF).
-
HPX_WITH_DOCUMENTATION_OUTPUT_FORMATS:STRING
¶
List of documentation output formats to generate. Valid options are html;singlehtml;latexpdf;man. Multiple values can be separated with semicolons. (default html).
-
HPX_WITH_EXAMPLES:BOOL
¶
Build the HPX examples (default ON)
-
HPX_WITH_EXAMPLES_HDF5:BOOL
¶
Enable examples requiring HDF5 support (default: OFF).
-
HPX_WITH_EXAMPLES_OPENMP:BOOL
¶
Enable examples requiring OpenMP support (default: OFF).
-
HPX_WITH_EXAMPLES_QT4:BOOL
¶
Enable examples requiring Qt4 support (default: OFF).
-
HPX_WITH_EXAMPLES_QTHREADS:BOOL
¶
Enable examples requiring QThreads support (default: OFF).
-
HPX_WITH_EXAMPLES_TBB:BOOL
¶
Enable examples requiring TBB support (default: OFF).
-
HPX_WITH_EXECUTABLE_PREFIX:STRING
¶
Executable prefix (default none), ‘hpx_’ useful for system install.
-
HPX_WITH_FAIL_COMPILE_TESTS:BOOL
¶
Create build system support for fail compile HPX tests (default ON)
-
HPX_WITH_IO_COUNTERS:BOOL
¶
Build HPX runtime (default: ON)
-
HPX_WITH_PSEUDO_DEPENDENCIES:BOOL
¶
Force creating pseudo targets and pseudo dependencies (default ON).
-
HPX_WITH_TESTS:BOOL
¶
Build the HPX tests (default ON)
-
HPX_WITH_TESTS_BENCHMARKS:BOOL
¶
Build HPX benchmark tests (default: ON)
-
HPX_WITH_TESTS_EXAMPLES:BOOL
¶
Add HPX examples as tests (default: ON)
-
HPX_WITH_TESTS_EXTERNAL_BUILD:BOOL
¶
Build external cmake build tests (default: ON)
-
HPX_WITH_TESTS_HEADERS:BOOL
¶
Build HPX header tests (default: OFF)
-
HPX_WITH_TESTS_REGRESSIONS:BOOL
¶
Build HPX regression tests (default: ON)
-
HPX_WITH_TESTS_UNIT:BOOL
¶
Build HPX unit tests (default: ON)
-
HPX_WITH_TOOLS:BOOL
¶
Build HPX tools (default: OFF)
Thread Manager options¶
HPX_SCHEDULER_MAX_TERMINATED_THREADS:STRING
HPX_WITH_IO_POOL:BOOL
HPX_WITH_MAX_CPU_COUNT:STRING
HPX_WITH_MAX_NUMA_DOMAIN_COUNT:STRING
HPX_WITH_MORE_THAN_64_THREADS:BOOL
HPX_WITH_SCHEDULER_LOCAL_STORAGE:BOOL
HPX_WITH_SPINLOCK_DEADLOCK_DETECTION:BOOL
HPX_WITH_SPINLOCK_POOL_NUM:STRING
HPX_WITH_STACKTRACES:BOOL
HPX_WITH_SWAP_CONTEXT_EMULATION:BOOL
HPX_WITH_THREAD_BACKTRACE_DEPTH:STRING
HPX_WITH_THREAD_BACKTRACE_ON_SUSPENSION:BOOL
HPX_WITH_THREAD_CREATION_AND_CLEANUP_RATES:BOOL
HPX_WITH_THREAD_CUMULATIVE_COUNTS:BOOL
HPX_WITH_THREAD_IDLE_RATES:BOOL
HPX_WITH_THREAD_LOCAL_STORAGE:BOOL
HPX_WITH_THREAD_MANAGER_IDLE_BACKOFF:BOOL
HPX_WITH_THREAD_QUEUE_WAITTIME:BOOL
HPX_WITH_THREAD_SCHEDULERS:STRING
HPX_WITH_THREAD_STACK_MMAP:BOOL
HPX_WITH_THREAD_STEALING_COUNTS:BOOL
HPX_WITH_THREAD_TARGET_ADDRESS:BOOL
HPX_WITH_TIMER_POOL:BOOL
-
HPX_SCHEDULER_MAX_TERMINATED_THREADS:STRING
¶
Maximum number of terminated threads collected before those are cleaned up (default: 100)
-
HPX_WITH_IO_POOL:BOOL
¶
Disable internal IO thread pool, do not change if not absolutely necessary (default: ON)
-
HPX_WITH_MAX_CPU_COUNT:STRING
¶
HPX applications will not use more that this number of OS-Threads (empty string means dynamic) (default: 64)
-
HPX_WITH_MAX_NUMA_DOMAIN_COUNT:STRING
¶
HPX applications will not run on machines with more NUMA domains (default: 8)
-
HPX_WITH_MORE_THAN_64_THREADS:BOOL
¶
HPX applications will be able to run on more than 64 cores (default: OFF)
-
HPX_WITH_SCHEDULER_LOCAL_STORAGE:BOOL
¶
Enable scheduler local storage for all HPX schedulers (default: OFF)
-
HPX_WITH_SPINLOCK_DEADLOCK_DETECTION:BOOL
¶
Enable spinlock deadlock detection (default: OFF)
-
HPX_WITH_SPINLOCK_POOL_NUM:STRING
¶
Number of elements a spinlock pool manages (default: 128)
-
HPX_WITH_STACKTRACES:BOOL
¶
Attach backtraces to HPX exceptions (default: ON)
-
HPX_WITH_SWAP_CONTEXT_EMULATION:BOOL
¶
Emulate SwapContext API for coroutines (default: OFF)
-
HPX_WITH_THREAD_BACKTRACE_DEPTH:STRING
¶
Thread stack back trace depth being captured (default: 5)
-
HPX_WITH_THREAD_BACKTRACE_ON_SUSPENSION:BOOL
¶
Enable thread stack back trace being captured on suspension (default: OFF)
-
HPX_WITH_THREAD_CREATION_AND_CLEANUP_RATES:BOOL
¶
Enable measuring thread creation and cleanup times (default: OFF)
-
HPX_WITH_THREAD_CUMULATIVE_COUNTS:BOOL
¶
Enable keeping track of cumulative thread counts in the schedulers (default: ON)
-
HPX_WITH_THREAD_IDLE_RATES:BOOL
¶
Enable measuring the percentage of overhead times spent in the scheduler (default: OFF)
-
HPX_WITH_THREAD_LOCAL_STORAGE:BOOL
¶
Enable thread local storage for all HPX threads (default: OFF)
-
HPX_WITH_THREAD_MANAGER_IDLE_BACKOFF:BOOL
¶
HPX scheduler threads do exponential backoff on idle queues (default: ON)
-
HPX_WITH_THREAD_QUEUE_WAITTIME:BOOL
¶
Enable collecting queue wait times for threads (default: OFF)
-
HPX_WITH_THREAD_SCHEDULERS:STRING
¶
Which thread schedulers are built. Options are: all, abp-priority, local, static-priority, static, shared-priority. For multiple enabled schedulers, separate with a semicolon (default: all)
-
HPX_WITH_THREAD_STACK_MMAP:BOOL
¶
Use mmap for stack allocation on appropriate platforms
-
HPX_WITH_THREAD_STEALING_COUNTS:BOOL
¶
Enable keeping track of counts of thread stealing incidents in the schedulers (default: OFF)
-
HPX_WITH_THREAD_TARGET_ADDRESS:BOOL
¶
Enable storing target address in thread for NUMA awareness (default: OFF)
-
HPX_WITH_TIMER_POOL:BOOL
¶
Disable internal timer thread pool, do not change if not absolutely necessary (default: ON)
AGAS options¶
-
HPX_WITH_AGAS_DUMP_REFCNT_ENTRIES:BOOL
¶
Enable dumps of the AGAS refcnt tables to logs (default: OFF)
Parcelport options¶
HPX_WITH_NETWORKING:BOOL
HPX_WITH_PARCELPORT_ACTION_COUNTERS:BOOL
HPX_WITH_PARCELPORT_LIBFABRIC:BOOL
HPX_WITH_PARCELPORT_MPI:BOOL
HPX_WITH_PARCELPORT_MPI_ENV:STRING
HPX_WITH_PARCELPORT_MPI_MULTITHREADED:BOOL
HPX_WITH_PARCELPORT_TCP:BOOL
HPX_WITH_PARCELPORT_VERBS:BOOL
HPX_WITH_PARCEL_PROFILING:BOOL
-
HPX_WITH_NETWORKING:BOOL
¶
Enable support for networking and multi-node runs (default: ON)
-
HPX_WITH_PARCELPORT_ACTION_COUNTERS:BOOL
¶
Enable performance counters reporting parcelport statistics on a per-action basis.
-
HPX_WITH_PARCELPORT_LIBFABRIC:BOOL
¶
Enable the libfabric based parcelport. This is currently an experimental feature
-
HPX_WITH_PARCELPORT_MPI:BOOL
¶
Enable the MPI based parcelport.
-
HPX_WITH_PARCELPORT_MPI_ENV:STRING
¶
List of environment variables checked to detect MPI (default: MV2_COMM_WORLD_RANK;PMI_RANK;OMPI_COMM_WORLD_SIZE;ALPS_APP_PE;PMIX_RANK).
-
HPX_WITH_PARCELPORT_MPI_MULTITHREADED:BOOL
¶
Turn on MPI multithreading support (default: ON).
-
HPX_WITH_PARCELPORT_TCP:BOOL
¶
Enable the TCP based parcelport.
-
HPX_WITH_PARCELPORT_VERBS:BOOL
¶
Enable the ibverbs based parcelport. This is currently an experimental feature
-
HPX_WITH_PARCEL_PROFILING:BOOL
¶
Enable profiling data for parcels
Profiling options¶
-
HPX_WITH_APEX:BOOL
¶
Enable APEX instrumentation support.
-
HPX_WITH_GOOGLE_PERFTOOLS:BOOL
¶
Enable Google Perftools instrumentation support.
-
HPX_WITH_ITTNOTIFY:BOOL
¶
Enable Amplifier (ITT) instrumentation support.
-
HPX_WITH_PAPI:BOOL
¶
Enable the PAPI based performance counter.
Debugging options¶
HPX_WITH_ATTACH_DEBUGGER_ON_TEST_FAILURE:BOOL
HPX_WITH_SANITIZERS:BOOL
HPX_WITH_TESTS_DEBUG_LOG:BOOL
HPX_WITH_TESTS_DEBUG_LOG_DESTINATION:STRING
HPX_WITH_THREAD_DEBUG_INFO:BOOL
HPX_WITH_THREAD_DESCRIPTION_FULL:BOOL
HPX_WITH_THREAD_GUARD_PAGE:BOOL
HPX_WITH_VALGRIND:BOOL
HPX_WITH_VERIFY_LOCKS:BOOL
HPX_WITH_VERIFY_LOCKS_BACKTRACE:BOOL
HPX_WITH_VERIFY_LOCKS_GLOBALLY:BOOL
-
HPX_WITH_ATTACH_DEBUGGER_ON_TEST_FAILURE:BOOL
¶
Break the debugger if a test has failed (default: OFF)
-
HPX_WITH_SANITIZERS:BOOL
¶
Configure with sanitizer instrumentation support.
-
HPX_WITH_TESTS_DEBUG_LOG:BOOL
¶
Turn on debug logs (–hpx:debug-hpx-log) for tests (default: OFF)
-
HPX_WITH_TESTS_DEBUG_LOG_DESTINATION:STRING
¶
Destination for test debug logs (default: cout)
-
HPX_WITH_THREAD_DEBUG_INFO:BOOL
¶
Enable thread debugging information (default: OFF, implicitly enabled in debug builds)
-
HPX_WITH_THREAD_DESCRIPTION_FULL:BOOL
¶
Use function address for thread description (default: OFF)
-
HPX_WITH_THREAD_GUARD_PAGE:BOOL
¶
Enable thread guard page (default: ON)
-
HPX_WITH_VALGRIND:BOOL
¶
Enable Valgrind instrumentation support.
-
HPX_WITH_VERIFY_LOCKS:BOOL
¶
Enable lock verification code (default: OFF, implicitly enabled in debug builds)
-
HPX_WITH_VERIFY_LOCKS_BACKTRACE:BOOL
¶
Enable thread stack back trace being captured on lock registration (to be used in combination with HPX_WITH_VERIFY_LOCKS=ON, default: OFF)
-
HPX_WITH_VERIFY_LOCKS_GLOBALLY:BOOL
¶
Enable global lock verification code (default: OFF, implicitly enabled in debug builds)
Modules options¶
HPX_PREPROCESSOR_WITH_COMPATIBILITY_HEADERS:BOOL
HPX_PREPROCESSOR_WITH_DEPRECATION_WARNINGS:BOOL
HPX_PREPROCESSOR_WITH_TESTS:BOOL
-
HPX_PREPROCESSOR_WITH_COMPATIBILITY_HEADERS:BOOL
¶
Enable compatibility headers for old headers
-
HPX_PREPROCESSOR_WITH_DEPRECATION_WARNINGS:BOOL
¶
Enable warnings for deprecated facilities. (default: Off)
-
HPX_PREPROCESSOR_WITH_TESTS:BOOL
¶
Build HPX preprocessor module tests. (default: ON)
Additional tools and libraries used by HPX¶
Here is a list of additional libraries and tools which are either optionally supported by the build system or are optionally required for certain examples or tests. These libraries and tools can be detected by the HPX build system.
Each of the tools or libraries listed here will be automatically detected if
they are installed in some standard location. If a tool or library is installed
in a different location you can specify its base directory by appending
_ROOT
to the variable name as listed below. For instance, to configure a
custom directory for BOOST
, specify BOOST_ROOT=/custom/boost/root
.
-
BOOST_ROOT:PATH
¶
Specifies where to look for the Boost installation to be used for compiling HPX Set this if CMake is not able to locate a suitable version of Boost The directory specified here can be either the root of a installed Boost distribution or the directory where you unpacked and built Boost without installing it (with staged libraries).
-
HWLOC_ROOT:PATH
¶
Specifies where to look for the Portable Hardware Locality (HWLOC) library. Set this if CMake is not able to locate a suitable version of Portable Hardware Locality (HWLOC) Portable Hardware Locality (HWLOC) provides platform independent support for extracting information about the used hardware architecture (number of cores, number of NUMA domains, hyperthreading, etc.). HPX utilizes this information if available.
-
PAPI_ROOT:PATH
¶
Specifies where to look for the Performance Application Programming Interface (PAPI) library. The PAPI library is necessary to compile a special component exposing PAPI hardware events and counters as HPX performance counters. This is not available on the Windows platform.
-
AMPLIFIER_ROOT:PATH
¶
Specifies where to look for one of the tools of the Intel Parallel Studio(tm) product, either Intel Amplifier(tm) or Intel Inspector(tm). This should be set if the CMake variable
HPX_USE_ITT_NOTIFY
is set toON
. Enabling ITT support in HPX will integrate any application with the mentioned Intel tools, which customizes the generated information for your application and improves the generated diagnostics.
In addition, some of the examples may need the following variables:
-
HDF5_ROOT:PATH
¶
Specifies where to look for the Hierarchical Data Format V5 (HDF5) include files and libraries.
Creating HPX projects¶
Using HPX with pkg-config¶
How to build HPX applications with pkg-config¶
After you are done installing HPX, you should be able to build the following
program. It prints Hello World!
on the locality you run it on.
// Copyright (c) 2007-2012 Hartmut Kaiser
//
// Distributed under the Boost Software License, Version 1.0. (See accompanying
// file LICENSE_1_0.txt or copy at http://www.boost.org/LICENSE_1_0.txt)
///////////////////////////////////////////////////////////////////////////////
// The purpose of this example is to execute a HPX-thread printing
// "Hello World!" once. That's all.
//[hello_world_1_getting_started
// Including 'hpx/hpx_main.hpp' instead of the usual 'hpx/hpx_init.hpp' enables
// to use the plain C-main below as the direct main HPX entry point.
#include <hpx/hpx_main.hpp>
#include <hpx/include/iostreams.hpp>
int main()
{
// Say hello to the world!
hpx::cout << "Hello World!\n" << hpx::flush;
return 0;
}
//]
Copy the text of this program into a file called hello_world.cpp.
Now, in the directory where you put hello_world.cpp, issue the following
commands (where $HPX_LOCATION
is the build directory or
CMAKE_INSTALL_PREFIX
you used while building HPX):
export PKG_CONFIG_PATH=$PKG_CONFIG_PATH:$HPX_LOCATION/lib/pkgconfig
c++ -o hello_world hello_world.cpp \
`pkg-config --cflags --libs hpx_application`\
-lhpx_iostreams -DHPX_APPLICATION_NAME=hello_world
Important
When using pkg-config with HPX, the pkg-config flags must go after the
-o
flag.
Note
HPX libraries have different names in debug and release mode. If you want
to link against a debug HPX library, you need to use the _debug
suffix
for the pkg-config name. That means instead of hpx_application
or
hpx_component
you will have to use hpx_application_debug
or
hpx_component_debug
Moreover, all referenced HPX components need to
have a appended d
suffix, e.g. instead of -lhpx_iostreams
you will
need to specify -lhpx_iostreamsd
.
Important
If the HPX libraries are in a path that is not found by the dynamic
linker. You need to add the path $HPX_LOCATION/lib
to your linker search
path (for example LD_LIBRARY_PATH
on Linux).
To test the program, type:
./hello_world
which should print Hello World!
and exit.
How to build HPX components with pkg-config¶
Let’s try a more complex example involving an HPX component. An HPX component is a class which exposes HPX actions. HPX components are compiled into dynamically loaded modules called component libraries. Here’s the source code:
hello_world_component.cpp
#include "hello_world_component.hpp"
#include <hpx/include/iostreams.hpp>
#include <iostream>
namespace examples { namespace server
{
void hello_world::invoke()
{
hpx::cout << "Hello HPX World!" << std::endl;
}
}}
HPX_REGISTER_COMPONENT_MODULE();
typedef hpx::components::component<
examples::server::hello_world
> hello_world_type;
HPX_REGISTER_COMPONENT(hello_world_type, hello_world);
HPX_REGISTER_ACTION(
examples::server::hello_world::invoke_action, hello_world_invoke_action);
hello_world_component.hpp
#if !defined(HELLO_WORLD_COMPONENT_HPP)
#define HELLO_WORLD_COMPONENT_HPP
#include <hpx/hpx.hpp>
#include <hpx/include/actions.hpp>
#include <hpx/include/lcos.hpp>
#include <hpx/include/components.hpp>
#include <hpx/include/serialization.hpp>
#include <utility>
namespace examples { namespace server
{
struct HPX_COMPONENT_EXPORT hello_world
: hpx::components::component_base<hello_world>
{
void invoke();
HPX_DEFINE_COMPONENT_ACTION(hello_world, invoke);
};
}}
HPX_REGISTER_ACTION_DECLARATION(
examples::server::hello_world::invoke_action, hello_world_invoke_action);
namespace examples
{
struct hello_world
: hpx::components::client_base<hello_world, server::hello_world>
{
typedef hpx::components::client_base<hello_world, server::hello_world>
base_type;
hello_world(hpx::future<hpx::naming::id_type> && f)
: base_type(std::move(f))
{}
hello_world(hpx::naming::id_type && f)
: base_type(std::move(f))
{}
void invoke()
{
hpx::async<server::hello_world::invoke_action>(this->get_id()).get();
}
};
}
#endif // HELLO_WORLD_COMPONENT_HPP
hello_world_client.cpp
// Copyright (c) 2012 Bryce Lelbach
//
// Distributed under the Boost Software License, Version 1.0. (See accompanying
// file LICENSE_1_0.txt or copy at http://www.boost.org/LICENSE_1_0.txt)
//[hello_world_client_getting_started
#include "hello_world_component.hpp"
#include <hpx/hpx_init.hpp>
int hpx_main(boost::program_options::variables_map&)
{
{
// Create a single instance of the component on this locality.
examples::hello_world client =
hpx::new_<examples::hello_world>(hpx::find_here());
// Invoke the component's action, which will print "Hello World!".
client.invoke();
}
return hpx::finalize(); // Initiate shutdown of the runtime system.
}
int main(int argc, char* argv[])
{
return hpx::init(argc, argv); // Initialize and run HPX.
}
//]
Copy the three source files above into three files (called
hello_world_component.cpp
, hello_world_component.hpp
and
hello_world_client.cpp
respectively).
Now, in the directory where you put the files, run the following command to
build the component library. (where $HPX_LOCATION
is the build directory or
CMAKE_INSTALL_PREFIX
you used while building HPX):
export PKG_CONFIG_PATH=$PKG_CONFIG_PATH:$HPX_LOCATION/lib/pkgconfig
c++ -o libhpx_hello_world.so hello_world_component.cpp \
`pkg-config --cflags --libs hpx_component` \
-lhpx_iostreams -DHPX_COMPONENT_NAME=hpx_hello_world
Now pick a directory in which to install your HPX component libraries. For
this example, we’ll choose a directory named my_hpx_libs
:
mkdir ~/my_hpx_libs
mv libhpx_hello_world.so ~/my_hpx_libs
Note
HPX libraries have different names in debug and release mode. If you want
to link against a debug HPX library, you need to use the _debug
suffix
for the pkg-config name. That means instead of hpx_application
or
hpx_component
you will have to use hpx_application_debug
or
hpx_component_debug
. Moreover, all referenced HPX components need to
have a appended d
suffix, e.g. instead of -lhpx_iostreams
you will
need to specify -lhpx_iostreamsd
.
Important
If the HPX libraries are in a path that is not found by the dynamic linker.
You need to add the path $HPX_LOCATION/lib
to your linker search path
(for example LD_LIBRARY_PATH
on Linux).
Now, to build the application that uses this component (hello_world_client.cpp
),
we do:
export PKG_CONFIG_PATH=$PKG_CONFIG_PATH:$HPX_LOCATION/lib/pkgconfig
c++ -o hello_world_client hello_world_client.cpp \
``pkg-config --cflags --libs hpx_application``\
-L${HOME}/my_hpx_libs -lhpx_hello_world -lhpx_iostreams
Important
When using pkg-config with HPX, the pkg-config flags must go after the
-o
flag.
Finally, you’ll need to set your LD_LIBRARY_PATH before you can run the program. To run the program, type:
export LD_LIBRARY_PATH="$LD_LIBRARY_PATH:$HOME/my_hpx_libs"
./hello_world_client
which should print Hello HPX World!
and exit.
Using HPX with CMake-based projects¶
In Addition to the pkg-config support discussed on the previous pages, HPX comes with full CMake support. In order to integrate HPX into your existing, or new CMakeLists.txt you can leverage the find_package command integrated into CMake. Following is a Hello World component example using CMake.
Let’s revisit what we have. We have three files which compose our example application:
hello_world_component.hpp
hello_world_component.cpp
hello_world_client.hpp
The basic structure to include HPX into your CMakeLists.txt is shown here:
# Require a recent version of cmake
cmake_minimum_required(VERSION 3.3.2 FATAL_ERROR)
# This project is C++ based.
project(your_app CXX)
# Instruct cmake to find the HPX settings
find_package(HPX)
In order to have CMake find HPX, it needs to be told where to look for the
HPXConfig.cmake
file that is generated when HPX is built or installed, it is
used by find_package(HPX)
to set up all the necessary macros needed to use
HPX in your project. The ways to achieve this are:
set the
HPX_DIR
cmake variable to point to the directory containing theHPXConfig.cmake
script on the command line when you invoke cmake:cmake -DHPX_DIR=$HPX_LOCATION/lib/cmake/HPX ...
where
$HPX_LOCATION
is the build directory orCMAKE_INSTALL_PREFIX
you used when building/configuring HPX.set the
CMAKE_PREFIX_PATH
variable to the root directory of your HPX build or install location on the command line when you invoke cmake:cmake -DCMAKE_PREFIX_PATH=$HPX_LOCATION ...
the difference between
CMAKE_PREFIX_PATH
andHPX_DIR
is that cmake will add common postfixes such aslib/cmake/<project
to theMAKE_PREFIX_PATH
and search in these locations too. Note that if your project uses HPX as well as other cmake managed projects, the paths to the locations of these multiple projects may be concatenated in theCMAKE_PREFIX_PATH
.The variables above may be set in the CMake GUI or curses ccmake interface instead of the command line.
Additionally, if you wish to require HPX for your project, replace the
find_package(HPX)
line with find_package(HPX REQUIRED)
.
You can check if HPX was successfully found with the HPX_FOUND
CMake variable.
The simplest way to add the HPX component is to use the add_hpx_component
macro and add it to the CMakeLists.txt
file:
# build your application using HPX
add_hpx_component(hello_world
SOURCES hello_world_component.cpp
HEADERS hello_world_component.hpp
COMPONENT_DEPENDENCIES iostreams)
Note
add_hpx_component
adds a _component
suffix to the target name. In the
example above a hello_world_component
target will be created.
The available options to add_hpx_component
are:
SOURCES
: The source files for that componentHEADERS
: The header files for that componentDEPENDENCIES
: Other libraries or targets this component depends onCOMPONENT_DEPENDENCIES
: The components this component depends onPLUGIN
: Treat this component as a plugin-able libraryCOMPILE_FLAGS
: Additional compiler flagsLINK_FLAGS
: Additional linker flagsFOLDER
: Add the headers and source files to this Source Group folder
EXCLUDE_FROM_ALL
: Do not build this component as part of theall
target
After adding the component, the way you add the executable is as follows:
# build your application using HPX
add_hpx_executable(hello_world
ESSENTIAL
SOURCES hello_world_client.cpp
COMPONENT_DEPENDENCIES hello_world)
Note
add_hpx_executable
automatically adds a _component
suffix to dependencies
specified in COMPONENT_DEPENDENCIES
, meaning you can directly use the name given
when adding a component using add_hpx_component
.
When you configure your application, all you need to do is set the HPX_DIR
variable to point to the installation of HPX!
Note
All library targets built with HPX are exported and readily available to be
used as arguments to target_link_libraries
in your targets. The HPX include directories are available with the
HPX_INCLUDE_DIRS
CMake variable.
CMake macros to integrate HPX into existing applications¶
In addition to the add_hpx_component
and add_hpx_executable
you can use
the hpx_setup_target
macro to have an already existing target to be used
with the HPX libraries:
hpx_setup_target(target)
Optional parameters are:
EXPORT
: Adds it to the CMake export list HPXTargetsINSTALL
: Generates a install rule for the targetPLUGIN
: Treat this component as a plugin-able libraryTYPE
: The type can be: EXECUTABLE, LIBRARY or COMPONENTDEPENDENCIES
: Other libraries or targets this component depends onCOMPONENT_DEPENDENCIES
: The components this component depends onCOMPILE_FLAGS
: Additional compiler flagsLINK_FLAGS
: Additional linker flags
If you do not use CMake, you can still build against HPX but you should refer to the section on How to build HPX components with pkg-config.
Note
Since HPX relies on dynamic libraries, the dynamic linker needs to know
where to look for them. If HPX isn’t installed into a path which is
configured as a linker search path, external projects need to either set
RPATH
or adapt LD_LIBRARY_PATH
to point to where the hpx libraries
reside. In order to set RPATH
s, you can include HPX_SetFullRPATH
in
your project after all libraries you want to link against have been added.
Please also consult the CMake documentation here.
Using HPX with Makefile¶
A basic project building with HPX is through creating makefiles. The process
of creating one can get complex depending upon the use of cmake parameter
HPX_WITH_HPX_MAIN
(which defaults to ON).
How to build HPX applications with makefile¶
If HPX is installed correctly, you should be able to build and run a simple
hello world program. It prints Hello World!
on the locality you
run it on.
// Copyright (c) 2007-2012 Hartmut Kaiser
//
// Distributed under the Boost Software License, Version 1.0. (See accompanying
// file LICENSE_1_0.txt or copy at http://www.boost.org/LICENSE_1_0.txt)
///////////////////////////////////////////////////////////////////////////////
// The purpose of this example is to execute a HPX-thread printing
// "Hello World!" once. That's all.
//[hello_world_1_getting_started
// Including 'hpx/hpx_main.hpp' instead of the usual 'hpx/hpx_init.hpp' enables
// to use the plain C-main below as the direct main HPX entry point.
#include <hpx/hpx_main.hpp>
#include <hpx/include/iostreams.hpp>
int main()
{
// Say hello to the world!
hpx::cout << "Hello World!\n" << hpx::flush;
return 0;
}
//]
Copy the content of this program into a file called hello_world.cpp.
Now in the directory where you put hello_world.cpp, create a Makefile. Add the following code:
CXX=(CXX) # Add your favourite compiler here or let makefile choose default.
CXXFLAGS=-O3 -std=c++17
BOOST_ROOT=/path/to/boost
HWLOC_ROOT=/path/to/hwloc
TCMALLOC_ROOT=/path/to/tcmalloc
HPX_ROOT=/path/to/hpx
INCLUDE_DIRECTIVES=$(HPX_ROOT)/include $(BOOST_ROOT)/include $(HWLOC_ROOT)/include
LIBRARY_DIRECTIVES=-L$(HPX_ROOT)/lib $(HPX_ROOT)/lib/libhpx_init.a $(HPX_ROOT)/lib/libhpx.so $(BOOST_ROOT)/lib/libboost_atomic-mt.so $(BOOST_ROOT)/lib/libboost_filesystem-mt.so $(BOOST_ROOT)/lib/libboost_program_options-mt.so $(BOOST_ROOT)/lib/libboost_regex-mt.so $(BOOST_ROOT)/lib/libboost_system-mt.so -lpthread $(TCMALLOC_ROOT)/libtcmalloc_minimal.so $(HWLOC_ROOT)/libhwloc.so -ldl -lrt
LINK_FLAGS=$(HPX_ROOT)/lib/libhpx_wrap.a -Wl,-wrap=main # should be left empty for HPX_WITH_HPX_MAIN=OFF
hello_world: hello_world.o
$(CXX) $(CXXFLAGS) -o hello_world hello_world.o $(LIBRARY_DIRECTIVES) $(LINK_FLAGS)
hello_world.o:
$(CXX) $(CXXFLAGS) -c -o hello_world.o hello_world.cpp $(INCLUDE_DIRECTIVES)
Important
LINK_FLAGS
should be left empty if HPX_WITH_HPX_MAIN is set to OFF.
Boost in the above example is build with --layout=tagged
. Actual boost
flags may vary on your build of boost.
To build the program, type:
make
A successfull build should result in hello_world binary. To test, type:
./hello_world
How to build HPX components with makefile¶
Let’s try a more complex example involving an HPX component. An HPX component is a class which exposes HPX actions. HPX components are compiled into dynamically loaded modules called component libraries. Here’s the source code:
hello_world_component.cpp
#include "hello_world_component.hpp"
#include <hpx/include/iostreams.hpp>
#include <iostream>
namespace examples { namespace server
{
void hello_world::invoke()
{
hpx::cout << "Hello HPX World!" << std::endl;
}
}}
HPX_REGISTER_COMPONENT_MODULE();
typedef hpx::components::component<
examples::server::hello_world
> hello_world_type;
HPX_REGISTER_COMPONENT(hello_world_type, hello_world);
HPX_REGISTER_ACTION(
examples::server::hello_world::invoke_action, hello_world_invoke_action);
hello_world_component.hpp
#if !defined(HELLO_WORLD_COMPONENT_HPP)
#define HELLO_WORLD_COMPONENT_HPP
#include <hpx/hpx.hpp>
#include <hpx/include/actions.hpp>
#include <hpx/include/lcos.hpp>
#include <hpx/include/components.hpp>
#include <hpx/include/serialization.hpp>
#include <utility>
namespace examples { namespace server
{
struct HPX_COMPONENT_EXPORT hello_world
: hpx::components::component_base<hello_world>
{
void invoke();
HPX_DEFINE_COMPONENT_ACTION(hello_world, invoke);
};
}}
HPX_REGISTER_ACTION_DECLARATION(
examples::server::hello_world::invoke_action, hello_world_invoke_action);
namespace examples
{
struct hello_world
: hpx::components::client_base<hello_world, server::hello_world>
{
typedef hpx::components::client_base<hello_world, server::hello_world>
base_type;
hello_world(hpx::future<hpx::naming::id_type> && f)
: base_type(std::move(f))
{}
hello_world(hpx::naming::id_type && f)
: base_type(std::move(f))
{}
void invoke()
{
hpx::async<server::hello_world::invoke_action>(this->get_id()).get();
}
};
}
#endif // HELLO_WORLD_COMPONENT_HPP
hello_world_client.cpp
// Copyright (c) 2012 Bryce Lelbach
//
// Distributed under the Boost Software License, Version 1.0. (See accompanying
// file LICENSE_1_0.txt or copy at http://www.boost.org/LICENSE_1_0.txt)
//[hello_world_client_getting_started
#include "hello_world_component.hpp"
#include <hpx/hpx_init.hpp>
int hpx_main(boost::program_options::variables_map&)
{
{
// Create a single instance of the component on this locality.
examples::hello_world client =
hpx::new_<examples::hello_world>(hpx::find_here());
// Invoke the component's action, which will print "Hello World!".
client.invoke();
}
return hpx::finalize(); // Initiate shutdown of the runtime system.
}
int main(int argc, char* argv[])
{
return hpx::init(argc, argv); // Initialize and run HPX.
}
//]
Now in the directory, create a Makefile. Add the following code:
CXX=(CXX) # Add your favourite compiler here or let makefile choose default.
CXXFLAGS=-O3 -std=c++17
BOOST_ROOT=/path/to/boost
HWLOC_ROOT=/path/to/hwloc
TCMALLOC_ROOT=/path/to/tcmalloc
HPX_ROOT=/path/to/hpx
INCLUDE_DIRECTIVES=$(HPX_ROOT)/include $(BOOST_ROOT)/include $(HWLOC_ROOT)/include
LIBRARY_DIRECTIVES=-L$(HPX_ROOT)/lib $(HPX_ROOT)/lib/libhpx_init.a $(HPX_ROOT)/lib/libhpx.so $(BOOST_ROOT)/lib/libboost_atomic-mt.so $(BOOST_ROOT)/lib/libboost_filesystem-mt.so $(BOOST_ROOT)/lib/libboost_program_options-mt.so $(BOOST_ROOT)/lib/libboost_regex-mt.so $(BOOST_ROOT)/lib/libboost_system-mt.so -lpthread $(TCMALLOC_ROOT)/libtcmalloc_minimal.so $(HWLOC_ROOT)/libhwloc.so -ldl -lrt
LINK_FLAGS=$(HPX_ROOT)/lib/libhpx_wrap.a -Wl,-wrap=main # should be left empty for HPX_WITH_HPX_MAIN=OFF
hello_world_client: libhpx_hello_world hello_world_client.o
$(CXX) $(CXXFLAGS) -o hello_world_client $(LIBRARY_DIRECTIVES) libhpx_hello_world $(LINK_FLAGS)
hello_world_client.o: hello_world_client.cpp
$(CXX) $(CXXFLAGS) -o hello_world_client.o hello_world_client.cpp $(INCLUDE_DIRECTIVES)
libhpx_hello_world: hello_world_component.o
$(CXX) $(CXXFLAGS) -o libhpx_hello_world hello_world_component.o $(LIBRARY_DIRECTIVES)
hello_world_component.o: hello_world_component.cpp
$(CXX) $(CXXFLAGS) -c -o hello_world_component.o hello_world_component.cpp $(INCLUDE_DIRECTIVES)
To build the program, type:
make
A successfull build should result in hello_world binary. To test, type:
./hello_world
Note
Due to high variations in CMake flags and library dependencies, it is recommended to build HPX applications and components with pkg-config or CMakeLists.txt. Writing Makefile may result in broken builds if due care is not taken. pkg-config files and CMake systems are configured with CMake build of HPX. Hence, they are stable and provides with better support overall.
Starting the HPX runtime¶
In order to write an application which uses services from the HPX runtime system you need to initialize the HPX library by inserting certain calls into the code of your application. Depending on your use case, this can be done in 3 different ways:
- Minimally invasive: Re-use the
main()
function as the main HPX entry point. - Balanced use case: Supply your own main HPX entry point while blocking the main thread.
- Most flexibility: Supply your own main HPX entry point while avoiding to block the main thread.
- Suspend and resume: As above but suspend and resume the HPX runtime to allow for other runtimes to be used.
Re-use the main()
function as the main HPX entry point¶
This method is the least intrusive to your code. It however provides you with the smallest flexibility in terms of initializing the HPX runtime system. The following code snippet shows what a minimal HPX application using this technique looks like:
#include <hpx/hpx_main.hpp>
int main(int argc, char* argv[])
{
return 0;
}
The only change to your code you have to make is to include the file
hpx/hpx_main.hpp
. In this case the function main()
will be invoked as
the first HPX thread of the application. The runtime system will be
initialized behind the scenes before the function main()
is executed and
will automatically stop after main()
has returned. All HPX API functions
can be used from within this function now.
Note
The function main()
does not need to expect receiving argc
argv
as shown above, but could expose the signature int main()
. This is
consistent with the usually allowed prototypes for the function main()
in
C++ applications.
All command line arguments specific to HPX will still be processed by the
HPX runtime system as usual. However, those command line options will be
removed from the list of values passed to argc
/argv
of the function
main()
. The list of values passed to main()
will hold only the
commandline options which are not recognized by the HPX runtime system (see
the section HPX Command Line Options for more details on what options are recognized
by HPX).
Note
In this mode all one-letter-shortcuts are disabled which are normally
available on the HPX command line (such as -t
or -l
see
HPX Command Line Options). This is done to minimize any possible interaction
between the command line options recognized by the HPX runtime system and
any command line options defined by the application.
The value returned from the function main()
as shown above will be returned
to the operating system as usual.
Important
To achieve this seamless integration, the header file hpx/hpx_main.hpp
defines a macro:
#define main hpx_startup::user_main
which could result in unexpected behavior.
Important
To achieve this seamless integration, we use different implementations for
different Operating Systems. In case of Linux or Mac OSX, the code present in
hpx_wrap.cpp
is put into action. We hook into the system function in case
of Linux and provide alternate entry point in case of Mac OSX. For other
Operating Systems we rely on a macro:
#define main hpx_startup::user_main
provided in the header file hpx/hpx_main.hpp
. This implementation can
result in unexpected behavior.
Caution
We make use of an override variable include_libhpx_wrap
in the header
file hpx/hpx_main.hpp
to swiftly choose the function call stack at
runtime. Therefore, the header file should only be included in the main
executable. Including it in the components will result in multiple definition
of the variable.
Supply your own main HPX entry point while blocking the main thread¶
With this method you need to provide an explicit main thread function named
hpx_main
at global scope. This function will be invoked as the main entry
point of your HPX application on the console locality only (this
function will be invoked as the first HPX thread of your application). All
HPX API functions can be used from within this function.
The thread executing the function hpx::init
will block waiting for
the runtime system to exit. The value returned from hpx_main
will be
returned from hpx::init
after the runtime system has stopped.
The function hpx::finalize
has to be called on one of the HPX
localities in order to signal that all work has been scheduled and the runtime
system should be stopped after the scheduled work has been executed.
This method of invoking HPX has the advantage of you being able to decide
which version of hpx::init
to call. This allows to pass
additional configuration parameters while initializing the HPX runtime system.
#include <hpx/hpx_init.hpp>
int hpx_main(int argc, char* argv[])
{
// Any HPX application logic goes here...
return hpx::finalize();
}
int main(int argc, char* argv[])
{
// Initialize HPX, run hpx_main as the first HPX thread, and
// wait for hpx::finalize being called.
return hpx::init(argc, argv);
}
Note
The function hpx_main
does not need to expect receiving argc
/argv
as shown above, but could expose one of the following signatures:
int hpx_main();
int hpx_main(int argc, char* argv[]);
int hpx_main(boost::program_options::variables_map& vm);
This is consistent with (and extends) the usually allowed prototypes for the
function main()
in C++ applications.
The header file to include for this method of using HPX is
hpx/hpx_init.hpp
.
There are many additional overloads of hpx::init
available, such as
for instance to provide your own entry point function instead of hpx_main
.
Please refer to the function documentation for more details (see: hpx/hpx_init.hpp
).
Supply your own main HPX entry point while avoiding to block the main thread¶
With this method you need to provide an explicit main thread function named
hpx_main
at global scope. This function will be invoked as the main entry
point of your HPX application on the console locality only (this
function will be invoked as the first HPX thread of your application). All
HPX API functions can be used from within this function.
The thread executing the function hpx::start
will not block
waiting for the runtime system to exit, but will return immediately.
Important
You cannot use any of the HPX API functions other that
hpx::stop
from inside your main()
function.
The function hpx::finalize
has to be called on one of the HPX
localities in order to signal that all work has been scheduled and the runtime
system should be stopped after the scheduled work has been executed.
This method of invoking HPX is useful for applications where the main thread
is used for special operations, such a GUIs. The function hpx::stop
can be used to wait for the HPX runtime system to exit and should be at least
used as the last function called in main()
. The value returned from
hpx_main
will be returned from hpx::stop
after the runtime
system has stopped.
#include <hpx/hpx_start.hpp>
int hpx_main(int argc, char* argv[])
{
// Any HPX application logic goes here...
return hpx::finalize();
}
int main(int argc, char* argv[])
{
// Initialize HPX, run hpx_main.
hpx::start(argc, argv);
// ...Execute other code here...
// Wait for hpx::finalize being called.
return hpx::stop();
}
Note
The function hpx_main
does not need to expect receiving argc
/argv
as shown above, but could expose one of the following signatures:
int hpx_main();
int hpx_main(int argc, char* argv[]);
int hpx_main(boost::program_options::variables_map& vm);
This is consistent with (and extends) the usually allowed prototypes for the
function main()
in C++ applications.
The header file to include for this method of using HPX is
hpx/hpx_start.hpp
.
There are many additional overloads of hpx::start
available, such as
for instance to provide your own entry point function instead of hpx_main
.
Please refer to the function documentation for more details (see:
hpx/hpx_start.hpp
).
Suspending and resuming the HPX runtime¶
In some applications it is required to combine HPX with other runtimes. To
support this use case HPX provides two functions: hpx::suspend
and
hpx::resume
. hpx::suspend
is a blocking call which will
wait for all scheduled tasks to finish executing and then put the thread pool OS
threads to sleep. hpx::resume
simply wakes up the sleeping threads
so that they are ready to accept new work. hpx::suspend
and
hpx::resume
can be found in the header hpx/hpx_suspend.hpp
.
#include <hpx/hpx_start.hpp>
#include <hpx/hpx_suspend.hpp>
int main(int argc, char* argv[])
{
// Initialize HPX, don't run hpx_main
hpx::start(nullptr, argc, argv);
// Schedule a function on the HPX runtime
hpx::apply(&my_function, ...);
// Wait for all tasks to finish, and suspend the HPX runtime
hpx::suspend();
// Execute non-HPX code here
// Resume the HPX runtime
hpx::resume();
// Schedule more work on the HPX runtime
// hpx::finalize has to be called from the HPX runtime before hpx::stop
hpx::apply([]() { hpx::finalize(); });
return hpx::stop();
}
Note
hpx::suspend
does not wait for hpx::finalize
to be
called. Only call hpx::finalize
when you wish to fully stop the
HPX runtime.
HPX also supports suspending individual thread pools and threads. For details
on how to do that see the documentation for hpx::threads::thread_pool_base
.
Automatically suspending worker threads¶
The previous method guarantees that the worker threads are suspended when you ask for it and that they stay suspended. An alternative way to achieve the same effect is to tweak how quickly HPX suspends its worker threads when they run out of work. The following configuration values make sure that HPX idles very quickly:
hpx.max_idle_backoff_time = 1000
hpx.max_idle_loop_count = 0
They can be set on the command line using
--hpx:ini=hpx.max_idle_backoff_time=1000
and
--hpx:ini=hpx.max_idle_loop_count=0
. See Launching and configuring HPX applications
for more details on how to set configuration parameters.
After setting idling parameters the previous example could now be written like this instead:
#include <hpx/hpx_start.hpp>
int main(int argc, char* argv[])
{
// Initialize HPX, don't run hpx_main
hpx::start(nullptr, argc, argv);
// Schedule some functions on the HPX runtime
// NOTE: run_as_hpx_thread blocks until completion.
hpx::run_as_hpx_thread(&my_function, ...);
hpx::run_as_hpx_thread(&my_other_function, ...);
// hpx::finalize has to be called from the HPX runtime before hpx::stop
hpx::apply([]() { hpx::finalize(); });
return hpx::stop();
}
In this example each call to hpx::run_as_hpx_thread
acts as a
“parallel region”.
Working of hpx_main.hpp
¶
In order to initialize HPX from main()
, we make use of linker tricks.
It is implemented differently for different Operating Systems. Method of implementation is as follows:
- Linux: Using linker
--wrap
option. - Mac OSX: Using the linker
-e
option. - Windows: Using
#define main hpx_startup::user_main
Linux implementation¶
We make use of the Linux linker ld
’s --wrap
option to wrap the
main()
function. This way any call to main()
are redirected to our own
implementation of main. It is here that we check for the existence of
hpx_main.hpp
by making use of a shadow variable include_libhpx_wrap
. The
value of this variable determines the function stack at runtime.
The implementation can be found in libhpx_wrap.a
.
Important
It is necessary that hpx_main.hpp
be not included more than once.
Multiple inclusions can result in multiple definition of
include_libhpx_wrap
.
Mac OSX implementation¶
Here we make use of yet another linker option -e
to change the entry point
to our custom entry function initialize_main
. We initialize the HPX
runtime system from this function and call main from the initialized system. We
determine the function stack at runtime by making use of the shadow variable
include_libhpx_wrap
.
The implementation can be found in libhpx_wrap.a
.
Important
It is necessary that hpx_main.hpp
be not included more than once.
Multiple inclusions can result in multiple definition of
include_libhpx_wrap
.
Windows implementation¶
We make use of a macro #define main hpx_startup::user_main
to take care of
the initializations.
This implementation could result in unexpected behaviors.
Launching and configuring HPX applications¶
Configuring HPX applications¶
All HPX applications can be configured using special command line options and/or using special configuration files. This section describes the available options, the configuration file format, and the algorithm used to locate possible predefined configuration files. Additionally this section describes the defaults assumed if no external configuration information is supplied.
During startup any HPX application applies a predefined search pattern to locate one or more configuration files. All found files will be read and merged in the sequence they are found into one single internal database holding all configuration properties. This database is used during the execution of the application to configure different aspects of the runtime system.
In addition to the ini files, any application can supply its own configuration
files, which will be merged with the configuration database as well. Moreover,
the user can specify additional configuration parameters on the command line
when executing an application. The HPX runtime system will merge all command
line configuration options (see the description of the --hpx:ini
,
--hpx:config
, and --hpx:app-config
command line options).
The HPX INI File Format¶
All HPX applications can be configured using a special file format which is
similar to the well-known Windows INI file format. This is a structured text format
allowing to group key/value pairs (properties) into sections. The basic element
contained in an ini file is the property. Every property has a name and a
value, delimited by an equals sign '='
. The name appears to the left of the
equals sign:
name=value
The value may contain equal signs as only the first '='
character
is interpreted as the delimiter between name
and value
Whitespace before
the name, after the value and immediately before and after the delimiting equal
sign is ignored. Whitespace inside the value is retained.
Properties may be grouped into arbitrarily named sections. The section name
appears on a line by itself, in square brackets [
and ]
. All properties
after the section declaration are associated with that section. There is no
explicit “end of section” delimiter; sections end at the next section
declaration, or the end of the file:
[section]
In HPX sections can be nested. A nested section has a name composed of
all section names it is embedded in. The section names are concatenated using
a dot '.'
:
[outer_section.inner_section]
Here inner_section
is logically nested within outer_section
.
It is possible to use the full section name concatenated with the property name to refer to a particular property. For example in:
[a.b.c]
d = e
the property value of d
can be referred to as a.b.c.d=e
.
In HPX ini files can contain comments. Hash signs '#'
at the beginning
of a line indicate a comment. All characters starting with the '#'
until the
end of line are ignored.
If a property with the same name is reused inside a section, the second occurrence of this property name will override the first occurrence (discard the first value). Duplicate sections simply merge their properties together, as if they occurred contiguously.
In HPX ini files, a property value ${FOO:default}
will use the environmental
variable FOO
to extract the actual value if it is set and default
otherwise.
No default has to be specified. Therefore ${FOO}
refers to the environmental
variable FOO
. If FOO
is not set or empty the overall expression will evaluate
to an empty string. A property value $[section.key:default]
refers to the value
held by the property section.key
if it exists and default
otherwise. No
default has to be specified. Therefore $[section.key]
refers to the property
section.key
. If the property section.key
is not set or empty, the overall
expression will evaluate to an empty string.
Note
Any property $[section.key:default]
is evaluated whenever it is queried
and not when the configuration data is initialized. This allows for lazy
evaluation and relaxes initialization order of different sections. The only
exception are recursive property values, e.g. values referring to the very
key they are associated with. Those property values are evaluated at
initialization time to avoid infinite recursion.
Built-in Default Configuration Settings¶
During startup any HPX application applies a predefined search pattern to locate one or more configuration files. All found files will be read and merged in the sequence they are found into one single internal data structure holding all configuration properties.
As a first step the internal configuration database is filled with a set of default configuration properties. Those settings are described on a section by section basis below.
Note
You can print the default configuration settings used for an executable
by specifying the command line option --hpx:dump-config
.
system
configuration section¶[system]
pid = <process-id>
prefix = <current prefix path of core HPX library>
executable = <current prefix path of executable>
Property | Description |
system.pid |
This is initialized to store the current OS-process id of the application instance. |
system.prefix |
This is initialized to the base directory HPX has been loaded from. |
system.executable_prefix |
This is initialized to the base directory the current executable has been loaded from. |
hpx
configuration section¶[hpx]
location = ${HPX_LOCATION:$[system.prefix]}
component_path = $[hpx.location]/lib/hpx:$[system.executable_prefix]/lib/hpx:$[system.executable_prefix]/../lib/hpx
master_ini_path = $[hpx.location]/share/hpx-<version>:$[system.executable_prefix]/share/hpx-<version>:$[system.executable_prefix]/../share/hpx-<version>
ini_path = $[hpx.master_ini_path]/ini
os_threads = 1
localities = 1
program_name =
cmd_line =
lock_detection = ${HPX_LOCK_DETECTION:0}
throw_on_held_lock = ${HPX_THROW_ON_HELD_LOCK:1}
minimal_deadlock_detection = <debug>
spinlock_deadlock_detection = <debug>
spinlock_deadlock_detection_limit = ${HPX_SPINLOCK_DEADLOCK_DETECTION_LIMIT:1000000}
max_background_threads = ${HPX_MAX_BACKGROUND_THREADS:$[hpx.os_threads]}
max_idle_loop_count = ${HPX_MAX_IDLE_LOOP_COUNT:<hpx_idle_loop_count_max>}
max_busy_loop_count = ${HPX_MAX_BUSY_LOOP_COUNT:<hpx_busy_loop_count_max>}
max_idle_backoff_time = ${HPX_MAX_IDLE_BACKOFF_TIME:<hpx_idle_backoff_time_max>}
[hpx.stacks]
small_size = ${HPX_SMALL_STACK_SIZE:<hpx_small_stack_size>}
medium_size = ${HPX_MEDIUM_STACK_SIZE:<hpx_medium_stack_size>}
large_size = ${HPX_LARGE_STACK_SIZE:<hpx_large_stack_size>}
huge_size = ${HPX_HUGE_STACK_SIZE:<hpx_huge_stack_size>}
use_guard_pages = ${HPX_THREAD_GUARD_PAGE:1}
Property | Description |
hpx.location |
This is initialized to the id of the locality this application instance is running on. |
hpx.component_path |
Duplicates are discarded.
This property can refer to a list of directories separated by ':'
(Linux, Android, and MacOS) or using ';' (Windows). |
hpx.master_ini_path |
This is initialized to the list of default paths of the main hpx.ini
configuration files. This property can refer to a list of directories
separated by ':' (Linux, Android, and MacOS) or using ';'
(Windows). |
hpx.ini_path |
This is initialized to the default path where HPX will look for more
ini configuration files. This property can refer to a list of directories
separated by ':' (Linux, Android, and MacOS) or using ';'
(Windows). |
hpx.os_threads |
This setting reflects the number of OS-threads used for running HPX-threads. Defaults to number of detected cores (not hyperthreads/PUs). |
hpx.localities |
This setting reflects the number of localities the application is running
on. Defaults to 1 . |
hpx.program_name |
This setting reflects the program name of the application instance.
Initialized from the command line argv[0] . |
hpx.cmd_line |
This setting reflects the actual command line used to launch this application instance. |
hpx.lock_detection |
This setting verifies that no locks are being held while a HPX thread
is suspended. This setting is applicable only if
HPX_WITH_VERIFY_LOCKS is set during configuration in CMake. |
hpx.throw_on_held_lock |
This setting causes an exception if during lock detection at least one
lock is being held while a HPX thread is suspended. This setting is
applicable only if HPX_WITH_VERIFY_LOCKS is set during configuration
in CMake. This setting has no effect if hpx.lock_detection=0 . |
hpx.minimal_deadlock_detection |
This setting enables support for minimal deadlock detection for
HPX-threads. By default this is set to 1 (for Debug builds) or to
0 (for Release, RelWithDebInfo, RelMinSize builds), this setting is
effective only if HPX_WITH_THREAD_DEADLOCK_DETECTION is set during
configuration in CMake. |
hpx.spinlock_deadlock_detection |
This setting verifies that spinlocks don’t spin longer than specified
using the hpx.spinlock_deadlock_detection_limit . This setting is
applicable only if HPX_WITH_SPINLOCK_DEADLOCK_DETECTION is set during
configuration in CMake. By default this is set to 1 (for Debug
builds) or to 0 (for Release, RelWithDebInfo, RelMinSize builds). |
hpx.spinlock_deadlock_detection_limit |
This setting specifies the upper limit of allowed number of spins that
spinlocks are allowed to perform. This setting is applicable only if
HPX_WITH_SPINLOCK_DEADLOCK_DETECTION is set during configuration in
CMake. By default this is set to 1000000 . |
hpx.max_background_threads |
This setting defines the number of threads in the scheduler which are used to execute background work. By default this is the same as the number of cores used for the scheduler. |
hpx.max_idle_loop_count |
By default this is defined by the preprocessor constant
HPX_IDLE_LOOP_COUNT_MAX . This is an internal setting which you should
change only if you know exactly what you are doing. |
hpx.max_busy_loop_count |
This setting defines the maximum value of the busy-loop counter in the
scheduler. By default this is defined by the preprocessor constant
HPX_BUSY_LOOP_COUNT_MAX . This is an internal setting which you should
change only if you know exactly what you are doing. |
hpx.max_idle_backoff_time |
This setting defines the maximum time (in milliseconds) for the scheduler
to sleep after being idle for hpx.max_idle_loop_count iterations.
This setting is applicable only if
HPX_WITH_THREAD_MANAGER_IDLE_BACKOFF is set during configuration in
CMake. By default this is defined by the preprocessor constant
HPX_IDLE_BACKOFF_TIME_MAX . This is an internal setting which you
should change only if you know exactly what you are doing. |
hpx.stacks.small_size |
This is initialized to the small stack size to be used by HPX-threads.
Set by default to the value of the compile time preprocessor constant
HPX_SMALL_STACK_SIZE (defaults to 0x8000 ). This value is used for
all HPX threads by default, except for the thread running hpx_main
(which runs on a large stack). |
hpx.stacks.medium_size |
This is initialized to the medium stack size to be used by HPX-threads.
Set by default to the value of the compile time preprocessor constant
HPX_MEDIUM_STACK_SIZE (defaults to 0x20000 ). |
hpx.stacks.large_size |
This is initialized to the large stack size to be used by HPX-threads.
Set by default to the value of the compile time preprocessor constant
HPX_LARGE_STACK_SIZE (defaults to 0x200000 ). This setting is used
by default for the thread running hpx_main only. |
hpx.stacks.huge_size |
This is initialized to the huge stack size to be used by HPX-threads.
Set by default to the value of the compile time preprocessor constant
HPX_HUGE_STACK_SIZE (defaults to 0x2000000 ). |
hpx.stacks.use_guard_pages |
This entry controls whether the coroutine library will generate stack
guard pages or not. This entry is applicable on Linux only and only if
the HPX_USE_GENERIC_COROUTINE_CONTEXT option is not enabled and the
HPX_WITH_THREAD_GUARD_PAGE is set to 1 while configuring the build
system. It is set by default to 1 . |
hpx.threadpools
configuration section¶[hpx.threadpools]
io_pool_size = ${HPX_NUM_IO_POOL_SIZE:2}
parcel_pool_size = ${HPX_NUM_PARCEL_POOL_SIZE:2}
timer_pool_size = ${HPX_NUM_TIMER_POOL_SIZE:2}
Property | Description |
hpx.threadpools.io_pool_size |
The value of this property defines the number of OS-threads created for the internal I/O thread pool. |
hpx.threadpools.parcel_pool_size |
The value of this property defines the number of OS-threads created for the internal parcel thread pool. |
hpx.threadpools.timer_pool_size |
The value of this property defines the number of OS-threads created for the internal timer thread pool. |
hpx.thread_queue
configuration section¶Important
These setting control internal values used by the thread scheduling queues in the HPX scheduler. You should not modify these settings except if you know exactly what you are doing]
[hpx.thread_queue]
min_tasks_to_steal_pending = ${HPX_THREAD_QUEUE_MIN_TASKS_TO_STEAL_PENDING:0}
min_tasks_to_steal_staged = ${HPX_THREAD_QUEUE_MIN_TASKS_TO_STEAL_STAGED:10}
min_add_new_count = ${HPX_THREAD_QUEUE_MIN_ADD_NEW_COUNT:10}
max_add_new_count = ${HPX_THREAD_QUEUE_MAX_ADD_NEW_COUNT:10}
max_delete_count = ${HPX_THREAD_QUEUE_MAX_DELETE_COUNT:1000}
Property | Description |
hpx.thread_queue.min_tasks_to_steal_pending |
The value of this property defines the number of pending HPX threads which have to be available before neighboring cores are allowed to steal work. The default is to allow stealing always. |
hpx.thread_queue.min_tasks_to_steal_staged |
The value of this property defines the number of staged HPX tasks have which to be available before neighboring cores are allowed to steal work. The default is to allow stealing only if there are more tan 10 tasks available. |
hpx.thread_queue.min_add_new_count |
The value of this property defines the minimal number tasks to be converted into HPX threads whenever the thread queues for a core have run empty. |
hpx.thread_queue.max_add_new_count |
The value of this property defines the maximal number tasks to be converted into HPX threads whenever the thread queues for a core have run empty. |
hpx.thread_queue.max_delete_count |
The value of this property defines the number number of terminated HPX threads to discard during each invocation of the corresponding function. |
hpx.components
configuration section¶[hpx.components]
load_external = ${HPX_LOAD_EXTERNAL_COMPONENTS:1}
Property | Description |
hpx.components.load_external |
This entry defines whether external components will be loaded on this
locality. This entry normally is set to 1 and usually there is
no need to directly change this value. It is automatically set to 0 for
a dedicated AGAS server locality. |
Additionally, the section hpx.components
will be populated with the
information gathered from all found components. The information loaded for each
of the components will contain at least the following properties:
[hpx.components.<component_instance_name>]
name = <component_name>
path = <full_path_of_the_component_module>
enabled = $[hpx.components.load_external]
Property | Description |
hpx.components.<component_instance_name>.name |
This is the name of a component, usually the same as the second argument
to the macro used while registering the component with
HPX_REGISTER_COMPONENT . Set by the component factory. |
hpx.components.<component_instance_name>.path |
This is either the full path file name of the component module or the
directory the component module is located in. In this case, the component
module name will be derived from the property
hpx.components.<component_instance_name>.name . Set by the component
factory. |
hpx.components.<component_instance_name>.enabled |
This setting explicitly enables or disables the component. This is an optional property, HPX assumed that the component is enabled if it is not defined. |
The value for <component_instance_name>
is usually the same as for the
corresponding name
property. However generally it can be defined to any
arbitrary instance name. It is used to distinguish between different ini
sections, one for each component.
hpx.parcel
configuration section¶[hpx.parcel]
address = ${HPX_PARCEL_SERVER_ADDRESS:<hpx_initial_ip_address>}
port = ${HPX_PARCEL_SERVER_PORT:<hpx_initial_ip_port>}
bootstrap = ${HPX_PARCEL_BOOTSTRAP:<hpx_parcel_bootstrap>}
max_connections = ${HPX_PARCEL_MAX_CONNECTIONS:<hpx_parcel_max_connections>}
max_connections_per_locality = ${HPX_PARCEL_MAX_CONNECTIONS_PER_LOCALITY:<hpx_parcel_max_connections_per_locality>}
max_message_size = ${HPX_PARCEL_MAX_MESSAGE_SIZE:<hpx_parcel_max_message_size>}
max_outbound_message_size = ${HPX_PARCEL_MAX_OUTBOUND_MESSAGE_SIZE:<hpx_parcel_max_outbound_message_size>}
array_optimization = ${HPX_PARCEL_ARRAY_OPTIMIZATION:1}
zero_copy_optimization = ${HPX_PARCEL_ZERO_COPY_OPTIMIZATION:$[hpx.parcel.array_optimization]}
async_serialization = ${HPX_PARCEL_ASYNC_SERIALIZATION:1}
message_handlers = ${HPX_PARCEL_MESSAGE_HANDLERS:0}
Property | Description |
hpx.parcel.address |
This property defines the default IP address to be used for the parcel
layer to listen to. This IP address will be used as long as no other
values are specified (for instance using the --hpx:hpx command
line option). The expected format is any valid IP address or domain name
format which can be resolved into an IP address. The default depends on
the compile time preprocessor constant HPX_INITIAL_IP_ADDRESS
("127.0.0.1" ). |
hpx.parcel.port |
This property defines the default IP port to be used for the parcel layer
to listen to. This IP port will be used as long as no other values are
specified (for instance using the --hpx:hpx command line
option). The default depends on the compile time preprocessor
constant HPX_INITIAL_IP_PORT (7910 ). |
hpx.parcel.bootstrap |
This property defines which parcelport type should be used during
application bootstrap. The default depends on the compile time
preprocessor constant HPX_PARCEL_BOOTSTRAP ("tcp" ). |
hpx.parcel.max_connections |
This property defines how many network connections between different
localities are overall kept alive by each of locality. The
default depends on the compile time preprocessor constant
HPX_PARCEL_MAX_CONNECTIONS (512 ). |
hpx.parcel.max_connections_per_locality |
This property defines the maximum number of network connections that one
locality will open to another locality. The default depends
on the compile time preprocessor constant
HPX_PARCEL_MAX_CONNECTIONS_PER_LOCALITY (4 ). |
hpx.parcel.max_message_size |
This property defines the maximum allowed message size which will be
transferrable through the parcel layer. The default depends on the
compile time preprocessor constant HPX_PARCEL_MAX_MESSAGE_SIZE
(1000000000 bytes). |
hpx.parcel.max_outbound_message_size |
This property defines the maximum allowed outbound coalesced message size
which will be transferrable through the parcel layer. The default depends
on the compile time preprocessor constant
HPX_PARCEL_MAX_OUTBOUND_MESSAGE_SIZE (1000000 bytes). |
hpx.parcel.array_optimization |
This property defines whether this locality is allowed to utilize
array optimizations during serialization of parcel data. The default is
1 . |
hpx.parcel.zero_copy_optimization |
This property defines whether this locality is allowed to utilize
zero copy optimizations during serialization of parcel data. The default
is the same value as set for hpx.parcel.array_optimization . |
hpx.parcel.async_serialization |
This property defines whether this locality is allowed to spawn a
new thread for serialization (this is both for encoding and decoding
parcels). The default is 1 . |
hpx.parcel.message_handlers |
This property defines whether message handlers are loaded. The default is
0 . |
The following settings relate to the TCP/IP parcelport.
[hpx.parcel.tcp]
enable = ${HPX_HAVE_PARCELPORT_TCP:$[hpx.parcel.enabled]}
array_optimization = ${HPX_PARCEL_TCP_ARRAY_OPTIMIZATION:$[hpx.parcel.array_optimization]}
zero_copy_optimization = ${HPX_PARCEL_TCP_ZERO_COPY_OPTIMIZATION:$[hpx.parcel.zero_copy_optimization]}
async_serialization = ${HPX_PARCEL_TCP_ASYNC_SERIALIZATION:$[hpx.parcel.async_serialization]}
parcel_pool_size = ${HPX_PARCEL_TCP_PARCEL_POOL_SIZE:$[hpx.threadpools.parcel_pool_size]}
max_connections = ${HPX_PARCEL_TCP_MAX_CONNECTIONS:$[hpx.parcel.max_connections]}
max_connections_per_locality = ${HPX_PARCEL_TCP_MAX_CONNECTIONS_PER_LOCALITY:$[hpx.parcel.max_connections_per_locality]}
max_message_size = ${HPX_PARCEL_TCP_MAX_MESSAGE_SIZE:$[hpx.parcel.max_message_size]}
max_outbound_message_size = ${HPX_PARCEL_TCP_MAX_OUTBOUND_MESSAGE_SIZE:$[hpx.parcel.max_outbound_message_size]}
Property | Description |
hpx.parcel.tcp.enable |
Enable the use of the default TCP parcelport. Note that the initial bootstrap of the overall HPX application will be performed using the default TCP connections. This parcelport is enabled by default. This will be disabled only if MPI is enabled (see below). |
hpx.parcel.tcp.array_optimization |
This property defines whether this locality is allowed to utilize
array optimizations in the TCP/IP parcelport during serialization of
parcel data. The default is the same value as set for
hpx.parcel.array_optimization . |
hpx.parcel.tcp.zero_copy_optimization |
This property defines whether this locality is allowed to utilize
zero copy optimizations in the TCP/IP parcelport during serialization of
parcel data. The default is the same value as set for
hpx.parcel.zero_copy_optimization . |
hpx.parcel.tcp.async_serialization |
This property defines whether this locality is allowed to spawn a
new thread for serialization in the TCP/IP parcelport (this is both for
encoding and decoding parcels). The default is the same value as set for
hpx.parcel.async_serialization . |
hpx.parcel.tcp.parcel_pool_size |
The value of this property defines the number of OS-threads created for
the internal parcel thread pool of the TCP parcel port. The default is
taken from hpx.threadpools.parcel_pool_size . |
hpx.parcel.tcp.max_connections |
This property defines how many network connections between different
localities are overall kept alive by each of locality. The
default is taken from hpx.parcel.max_connections . |
hpx.parcel.tcp.max_connections_per_locality |
This property defines the maximum number of network connections that one
locality will open to another locality. The default is
taken from hpx.parcel.max_connections_per_locality . |
hpx.parcel.tcp.max_message_size |
This property defines the maximum allowed message size which will be
transferrable through the parcel layer. The default is taken from
hpx.parcel.max_message_size . |
hpx.parcel.tcp.max_outbound_message_size |
This property defines the maximum allowed outbound coalesced message size
which will be transferrable through the parcel layer. The default is
taken from hpx.parcel.max_outbound_connections . |
The following settings relate to the MPI parcelport. These settings take effect
only if the compile time constant HPX_HAVE_PARCELPORT_MPI
is set (the
equivalent cmake variable is HPX_WITH_PARCELPORT_MPI
and has to be set to
ON
.
[hpx.parcel.mpi]
enable = ${HPX_HAVE_PARCELPORT_MPI:$[hpx.parcel.enabled]}
env = ${HPX_HAVE_PARCELPORT_MPI_ENV:MV2_COMM_WORLD_RANK,PMI_RANK,OMPI_COMM_WORLD_SIZE,ALPS_APP_PE}
multithreaded = ${HPX_HAVE_PARCELPORT_MPI_MULTITHREADED:0}
rank = <MPI_rank>
processor_name = <MPI_processor_name>
array_optimization = ${HPX_HAVE_PARCEL_MPI_ARRAY_OPTIMIZATION:$[hpx.parcel.array_optimization]}
zero_copy_optimization = ${HPX_HAVE_PARCEL_MPI_ZERO_COPY_OPTIMIZATION:$[hpx.parcel.zero_copy_optimization]}
use_io_pool = ${HPX_HAVE_PARCEL_MPI_USE_IO_POOL:$1}
async_serialization = ${HPX_HAVE_PARCEL_MPI_ASYNC_SERIALIZATION:$[hpx.parcel.async_serialization]}
parcel_pool_size = ${HPX_HAVE_PARCEL_MPI_PARCEL_POOL_SIZE:$[hpx.threadpools.parcel_pool_size]}
max_connections = ${HPX_HAVE_PARCEL_MPI_MAX_CONNECTIONS:$[hpx.parcel.max_connections]}
max_connections_per_locality = ${HPX_HAVE_PARCEL_MPI_MAX_CONNECTIONS_PER_LOCALITY:$[hpx.parcel.max_connections_per_locality]}
max_message_size = ${HPX_HAVE_PARCEL_MPI_MAX_MESSAGE_SIZE:$[hpx.parcel.max_message_size]}
max_outbound_message_size = ${HPX_HAVE_PARCEL_MPI_MAX_OUTBOUND_MESSAGE_SIZE:$[hpx.parcel.max_outbound_message_size]}
Property | Description |
hpx.parcel.mpi.enable |
Enable the use of the MPI parcelport. HPX tries to detect if the application was started within a parallel MPI environment. If the detection was succesful, the MPI parcelport is enabled by default. To explicitly disable the MPI parcelport, set to 0. Note that the initial bootstrap of the overall HPX application will be performed using MPI as well. |
hpx.parcel.mpi.env |
This property influences which environment variables (comma separated) will be analyzed to find out whether the application was invoked by MPI. |
hpx.parcel.mpi.multithreaded |
This property is used to determine what threading mode to use when
initializing MPI. If this setting is 0 HPX will initialize MPI with
MPI_THREAD_SINGLE if the value is not equal to 0 HPX will
initialize MPI with MPI_THREAD_MULTI . |
hpx.parcel.mpi.rank |
This property will be initialized to the MPI rank of the locality. |
hpx.parcel.mpi.processor_name |
This property will be initialized to the MPI processor name of the locality. |
hpx.parcel.mpi.array_optimization |
This property defines whether this locality is allowed to utilize
array optimizations in the MPI parcelport during serialization of
parcel data. The default is the same value as set for
hpx.parcel.array_optimization . |
hpx.parcel.mpi.zero_copy_optimization |
This property defines whether this locality is allowed to utilize
zero copy optimizations in the MPI parcelport during serialization of
parcel data. The default is the same value as set for
hpx.parcel.zero_copy_optimization . |
hpx.parcel.mpi.use_io_pool |
This property can be set to run the progress thread inside of HPX threads
instead of a separate thread pool. The default is 1 . |
hpx.parcel.mpi.async_serialization |
This property defines whether this locality is allowed to spawn a
new thread for serialization in the MPI parcelport (this is both for
encoding and decoding parcels). The default is the same value as set for
hpx.parcel.async_serialization . |
hpx.parcel.mpi.parcel_pool_size |
The value of this property defines the number of OS-threads created for
the internal parcel thread pool of the MPI parcel port. The default is
taken from hpx.threadpools.parcel_pool_size . |
hpx.parcel.mpi.max_connections |
This property defines how many network connections between different
localities are overall kept alive by each of locality. The
default is taken from hpx.parcel.max_connections . |
hpx.parcel.mpi.max_connections_per_locality |
This property defines the maximum number of network connections that one
locality will open to another locality. The default is
taken from hpx.parcel.max_connections_per_locality . |
hpx.parcel.mpi.max_message_size |
This property defines the maximum allowed message size which will be
transferrable through the parcel layer. The default is taken from
hpx.parcel.max_message_size . |
hpx.parcel.mpi.max_outbound_message_size |
This property defines the maximum allowed outbound coalesced message size
which will be transferrable through the parcel layer. The default is
taken from hpx.parcel.max_outbound_connections . |
hpx.agas
configuration section¶[hpx.agas]
address = ${HPX_AGAS_SERVER_ADDRESS:<hpx_initial_ip_address>}
port = ${HPX_AGAS_SERVER_PORT:<hpx_initial_ip_port>}
service_mode = hosted
dedicated_server = 0
max_pending_refcnt_requests = ${HPX_AGAS_MAX_PENDING_REFCNT_REQUESTS:<hpx_initial_agas_max_pending_refcnt_requests>}
use_caching = ${HPX_AGAS_USE_CACHING:1}
use_range_caching = ${HPX_AGAS_USE_RANGE_CACHING:1}
local_cache_size = ${HPX_AGAS_LOCAL_CACHE_SIZE:<hpx_agas_local_cache_size>}
Property | Description |
hpx.agas.address |
This property defines the default IP address to be used for the
AGAS root server. This IP address will be used as long as no
other values are specified (for instance using the --hpx:agas
command line option). The expected format is any valid IP address or
domain name format which can be resolved into an IP address. The default
depends on the compile time preprocessor constant
HPX_INITIAL_IP_ADDRESS ("127.0.0.1" ). |
hpx.agas.port |
This property defines the default IP port to be used for the AGAS
root server. This IP port will be used as long as no other values are
specified (for instance using the --hpx:agas command line
option). The default depends on the compile time preprocessor constant
HPX_INITIAL_IP_PORT (7009 ). |
hpx.agas.service_mode |
This property specifies what type of AGAS service is running on
this locality. Currently, two modes exist. The locality
that acts as the AGAS server runs in bootstrap mode. All other
localities are in hosted mode. |
hpx.agas.dedicated_server |
This property specifies whether the AGAS server is exclusively
running AGAS services and not hosting any application components.
It is a boolean value. Set to 1 if
--hpx:run-agas-server-only is present. |
hpx.agas.max_pending_refcnt_requests |
This property defines the number of reference counting requests
(increments or decrements) to buffer. The default depends on the compile
time preprocessor constant
HPX_INITIAL_AGAS_MAX_PENDING_REFCNT_REQUESTS (4096 ). |
hpx.agas.use_caching |
This property specifies whether a software address translation cache is
used. It is a boolean value. Defaults to 1 . |
hpx.agas.use_range_caching |
This property specifies whether range-based caching is used by the
software address translation cache. This property is ignored if
hpx.agas.use_caching is false. It is a boolean value. Defaults to 1 . |
hpx.agas.local_cache_size |
This property defines the size of the software address translation cache
for AGAS services. This property is ignored
if hpx.agas.use_caching is false. Note that if
hpx.agas.use_range_caching is true, this size will refer to the
maximum number of ranges stored in the cache, not the number of entries
spanned by the cache. The default depends on the compile time
preprocessor constant HPX_AGAS_LOCAL_CACHE_SIZE (4096 ). |
hpx.commandline
configuration section¶The following table lists the definition of all pre-defined command line option shortcuts. For more information about commandline options see the section HPX Command Line Options.
[hpx.commandline]
aliasing = ${HPX_COMMANDLINE_ALIASING:1}
allow_unknown = ${HPX_COMMANDLINE_ALLOW_UNKNOWN:0}
[hpx.commandline.aliases]
-a = --hpx:agas
-c = --hpx:console
-h = --hpx:help
-I = --hpx:ini
-l = --hpx:localities
-p = --hpx:app-config
-q = --hpx:queuing
-r = --hpx:run-agas-server
-t = --hpx:threads
-v = --hpx:version
-w = --hpx:worker
-x = --hpx:hpx
-0 = --hpx:node=0
-1 = --hpx:node=1
-2 = --hpx:node=2
-3 = --hpx:node=3
-4 = --hpx:node=4
-5 = --hpx:node=5
-6 = --hpx:node=6
-7 = --hpx:node=7
-8 = --hpx:node=8
-9 = --hpx:node=9
Property | Description |
hpx.commandline.aliasing |
Enable command line aliases as defined in the section
hpx.commandline.aliases (see below). Defaults to 1 . |
hpx.commandline.allow_unknown |
Allow for unknown command line options to be passed through to
hpx_main() Defaults to 0 . |
hpx.commandline.aliases.-a |
On the commandline, -a expands to: --hpx:agas . |
hpx.commandline.aliases.-c |
On the commandline, -c expands to: --hpx:console . |
hpx.commandline.aliases.-h |
On the commandline, -h expands to: --hpx:help . |
hpx.commandline.aliases.--help |
On the commandline, --help expands to: --hpx:help . |
hpx.commandline.aliases.-I |
On the commandline, -I expands to: --hpx:ini . |
hpx.commandline.aliases.-l |
On the commandline, -l expands to: --hpx:localities . |
hpx.commandline.aliases.-p |
On the commandline, -p expands to: --hpx:app-config . |
hpx.commandline.aliases.-q |
On the commandline, -q expands to: --hpx:queuing . |
hpx.commandline.aliases.-r |
On the commandline, -r expands to: --hpx:run-agas-server . |
hpx.commandline.aliases.-t |
On the commandline, -t expands to: --hpx:threads . |
hpx.commandline.aliases.-v |
On the commandline, -v expands to: --hpx:version . |
hpx.commandline.aliases.--version |
On the commandline, --version expands to: --hpx:version . |
hpx.commandline.aliases.-w |
On the commandline, -w expands to: --hpx:worker . |
hpx.commandline.aliases.-x |
On the commandline, -x expands to: --hpx:hpx . |
hpx.commandline.aliases.-0 |
On the commandline, -0 expands to: --hpx:node =0 . |
hpx.commandline.aliases.-1 |
On the commandline, -1 expands to: --hpx:node =1 . |
hpx.commandline.aliases.-2 |
On the commandline, -2 expands to: --hpx:node =2 . |
hpx.commandline.aliases.-3 |
On the commandline, -3 expands to: --hpx:node =3 . |
hpx.commandline.aliases.-4 |
On the commandline, -4 expands to: --hpx:node =4 . |
hpx.commandline.aliases.-5 |
On the commandline, -5 expands to: --hpx:node =5 . |
hpx.commandline.aliases.-6 |
On the commandline, -6 expands to: --hpx:node =6 . |
hpx.commandline.aliases.-7 |
On the commandline, -7 expands to: --hpx:node =7 . |
hpx.commandline.aliases.-8 |
On the commandline, -8 expands to: --hpx:node =8 . |
hpx.commandline.aliases.-9 |
On the commandline, -9 expands to: --hpx:node =9 . |
Loading INI files¶
During startup and after the internal database has been initialized as described in the section Built-in Default Configuration Settings, HPX will try to locate and load additional ini files to be used as a source for configuration properties. This allows for a wide spectrum of additional customization possibilities by the user and system administrators. The sequence of locations where HPX will try loading the ini files is well defined and documented in this section. All ini files found are merged into the internal configuration database. The merge operation itself conforms to the rules as described in the section The HPX INI File Format.
- Load all component shared libraries found in the directories specified by the
property
hpx.component_path
and retrieve their default configuration information (see section Loading components for more details). This property can refer to a list of directories separated by':'
(Linux, Android, and MacOS) or using';'
(Windows). - Load all files named
hpx.ini
in the directories referenced by the propertyhpx.master_ini_path
This property can refer to a list of directories separated by':'
(Linux, Android, and MacOS) or using';'
(Windows). - Load a file named
.hpx.ini
in the current working directory, e.g. the directory the application was invoked from. - Load a file referenced by the environment variable
HPX_INI
. This variable is expected to provide the full path name of the ini configuration file (if any). - Load a file named
/etc/hpx.ini
. This lookup is done on non-Windows systems only. - Load a file named
.hpx.ini
in the home directory of the current user, e.g. the directory referenced by the environment variableHOME
. - Load a file named
.hpx.ini
in the directory referenced by the environment variablePWD
. - Load the file specified on the command line using the option
--hpx:config
. - Load all properties specified on the command line using the option
--hpx:ini
. The properties will be added to the database in the same sequence as they are specified on the command line. The format for those options is for instance--hpx:ini
=hpx.default_stack_size=0x4000
. In addition to the explicit command line options, this will set the following properties as implied from other settings:hpx.parcel.address
andhpx.parcel.port
as set by--hpx:hpx
hpx.agas.address
,hpx.agas.port
andhpx.agas.service_mode
as set by--hpx:agas
hpx.program_name
andhpx.cmd_line
will be derived from the actual command linehpx.os_threads
andhpx.localities
as set by--hpx:threads
and--hpx:localities
hpx.runtime_mode
will be derived from any explicit--hpx:console
,--hpx:worker
, or--hpx:connect
, or it will be derived from other settings, such as--hpx:node
=0
which implies--hpx:console
- Load files based on the pattern
*.ini
in all directories listed by the propertyhpx.ini_path
. All files found during this search will be merged. The propertyhpx.ini_path
can hold a list of directories separated by':'
(on Linux or Mac) or';'
(on Windows). - Load the file specified on the command line using the option
--hpx:app-config
. Note that this file will be merged as the content for a top level section[application]
.
Note
Any changes made to the configuration database caused by one of the steps
will influence the loading process for all subsequent steps. For instance, if
one of the ini files loaded changes the property hpx.ini_path
this will
influence the directories searched in step 9 as described above.
Important
The HPX core library will verify that all configuration settings specified
on the command line (using the --hpx:ini
option) will be checked
for validity. That means that the library will accept only known
configuration settings. This is to protect the user from unintentional typos
while specifying those settings. This behavior can be overwritten by
appending a '!'
to the configuration key, thus forcing the setting to be
entered into the configuration database, for instance: --hpx:ini
=hpx.foo! = 1
If any of the environment variables or files listed above is not found the corresponding loading step will be silently skipped.
Loading components¶
HPX relies on loading application specific components during the runtime of an application. Moreover, HPX comes with a set of preinstalled components supporting basic functionalities useful for almost every application. Any component in HPX is loaded from a shared library, where any of the shared libraries can contain more than one component type. During startup, HPX tries to locate all available components (e.g. their corresponding shared libraries) and creates an internal component registry for later use. This section describes the algorithm used by HPX to locate all relevant shared libraries on a system. As described, this algorithm is customizable by the configuration properties loaded from the ini files (see section Loading INI files).
Loading components is a two stage process. First HPX tries to locate all component shared libraries, loads those, and generates default configuration section in the internal configuration database for each component found. For each found component the following information is generated:
[hpx.components.<component_instance_name>]
name = <name_of_shared_library>
path = $[component_path]
enabled = $[hpx.components.load_external]
default = 1
The values in this section correspond to the expected configuration information for a component as described in the section Built-in Default Configuration Settings.
In order to locate component shared libraries, HPX will try loading all
shared libraries (files with the platform specific extension of a shared
library, Linux: *.so
, Windows: *.dll
, MacOS: *.dylib
found in the
directory referenced by the ini property hpx.component_path
).
This first step corresponds to step 1) during the process of filling the internal configuration database with default information as described in section Loading INI files.
After all of the configuration information has been loaded, HPX performs the
second step in terms of loading components. During this step, HPX scans all
existing configuration sections
[hpx.component.<some_component_instance_name>]
and instantiates a special
factory object for each of the successfully located and loaded components.
During the application’s life time, these factory objects will be responsible to
create new and discard old instances of the component they are associated with.
This step is performed after step 11) of the process of filling the internal
configuration database with default information as described in section
Loading INI files.
Application specific component example¶
In this section we assume to have a simple application component which exposes
one member function as a component action. The header file app_server.hpp
declares the C++ type to be exposed as a component. This type has a member
function print_greeting()
which is exposed as an action
print_greeting_action
. We assume the source files for this example are
located in a directory referenced by $APP_ROOT
:
// file: $APP_ROOT/app_server.hpp
#include <hpx/hpx.hpp>
#include <hpx/include/iostreams.hpp>
namespace app
{
// Define a simple component exposing one action 'print_greeting'
class HPX_COMPONENT_EXPORT server
: public hpx::components::component_base<server>
{
void print_greeting ()
{
hpx::cout << "Hey, how are you?\n" << hpx::flush;
}
// Component actions need to be declared, this also defines the
// type 'print_greeting_action' representing the action.
HPX_DEFINE_COMPONENT_ACTION(server, print_greeting, print_greeting_action);
};
}
// Declare boilerplate code required for each of the component actions.
HPX_REGISTER_ACTION_DECLARATION(app::server::print_greeting_action);
The corresponding source file contains mainly macro invocations which define boilerplate code needed for HPX to function properly:
// file: $APP_ROOT/app_server.cpp
#include "app_server.hpp"
// Define boilerplate required once per component module.
HPX_REGISTER_COMPONENT_MODULE();
// Define factory object associated with our component of type 'app::server'.
HPX_REGISTER_COMPONENT(app::server, app_server);
// Define boilerplate code required for each of the component actions. Use the
// same argument as used for HPX_REGISTER_ACTION_DECLARATION above.
HPX_REGISTER_ACTION(app::server::print_greeting_action);
The following gives an example of how the component can be used. We create one
instance of the app::server
component on the current locality and
invoke the exposed action print_greeting_action
using the global id of the
newly created instance. Note, that no special code is required to delete the
component instance after it is not needed anymore. It will be deleted
automatically when its last reference goes out of scope, here at the closing
brace of the block surrounding the code:
// file: $APP_ROOT/use_app_server_example.cpp
#include <hpx/hpx_init.hpp>
#include "app_server.hpp"
int hpx_main()
{
{
// Create an instance of the app_server component on the current locality.
hpx::naming:id_type app_server_instance =
hpx::create_component<app::server>(hpx::find_here());
// Create an instance of the action 'print_greeting_action'.
app::server::print_greeting_action print_greeting;
// Invoke the action 'print_greeting' on the newly created component.
print_greeting(app_server_instance);
}
return hpx::finalize();
}
int main(int argc, char* argv[])
{
return hpx::init(argc, argv);
}
In order to make sure that the application will be able to use the component
app::server
, special configuration information must be passed to HPX. The
simples way to allow HPX to ‘find’ the component is to provide special ini
configuration files, which add the necessary information to the internal
configuration database. The component should have a special ini file containing
the information specific to the component app_server
.
# file: $APP_ROOT/app_server.ini
[hpx.components.app_server]
name = app_server
path = $APP_LOCATION/
Here $APP_LOCATION
is the directory where the (binary) component shared
library is located. HPX will attempt to load the shared library from there.
The section name hpx.components.app_server
reflects the instance name of the
component (app_server
is an arbitrary, but unique name). The property value
for hpx.components.app_server.name
should be the same as used for the second
argument to the macro HPX_REGISTER_COMPONENT
above.
Additionally a file .hpx.ini
which could be located in the current working
directory (see step 3 as described in the section Loading INI files) can
be used to add to the ini search path for components:
# file: $PWD/.hpx.ini
[hpx]
ini_path = $[hpx.ini_path]:$APP_ROOT/
This assumes that the above ini file specific to the component is located in
the directory $APP_ROOT
.
Note
It is possible to reference the defined property from inside its value. HPX
will gracefully use the previous value of hpx.ini_path
for the reference
on the right hand side and assign the overall (now expanded) value to the
property.
Logging¶
HPX uses a sophisticated logging framework allowing to follow in detail what operations have been performed inside the HPX library in what sequence. This information proves to be very useful for diagnosing problems or just for improving the understanding what is happening in HPX as a consequence of invoking HPX API functionality.
Default logging¶
Enabling default logging is a simple process. The detailed description in the remainder of this section explains different ways to customize the defaults. Default logging can be enabled by using one of the following:
- a command line switch
--hpx:debug-hpx-log
, which will enable logging to the console terminal - the command line switch
--hpx:debug-hpx-log
=<filename>
, which enables logging to a given file<filename>
, or - setting an environment variable
HPX_LOGLEVEL=<loglevel>
while running the HPX application. In this case<loglevel>
should be a number between (or equal to)1
and5
where1
means minimal logging and5
causes to log all available messages. When setting the environment variable the logs will be written to a file namedhpx.<PID>.lo
in the current working directory, where<PID>
is the process id of the console instance of the application.
Customizing logging¶
Generally, logging can be customized either using environment variable settings or using by an ini configuration file. Logging is generated in several categories, each of which can be customized independently. All customizable configuration parameters have reasonable defaults, allowing to use logging without any additional configuration effort. The following table lists the available categories.
Category | Category shortcut | Information to be generated | Environment variable |
General | None | Logging information generated by different subsystems of HPX, such as thread-manager, parcel layer, LCOs, etc. | HPX_LOGLEVEL |
AGAS | AGAS |
Logging output generated by the AGAS subsystem | HPX_AGAS_LOGLEVEL |
Application | APP |
Logging generated by applications. | HPX_APP_LOGLEVEL |
By default, all logging output is redirected to the console instance of an application, where it is collected and written to a file, one file for each logging category.
Each logging category can be customized at two levels, the parameters for each
are stored in the ini configuration sections hpx.logging.CATEGORY
and
hpx.logging.console.CATEGORY
(where CATEGORY
is the category shortcut as
listed in the table above). The former influences logging at the source
locality and the latter modifies the logging behaviour for each of the
categories at the console instance of an application.
Levels¶
All HPX logging output have seven different logging levels. These levels can be set explicitly or through environmental variables in the main HPX ini file as shown below. The logging levels and their associated integral values are shown in the table below, ordered from most verbose to least verbose. By default, all HPX logs are set to 0, e.g. all logging output is disabled by default.
Logging level | Integral value |
---|---|
<debug> |
5 |
<info> |
4 |
<warning> |
3 |
<error> |
2 |
<fatal> |
1 |
No logging | 0 |
Tip
The easiest way to enable logging output is to set the environment variable
corresponding to the logging category to an integral value as described in
the table above. For instance, setting HPX_LOGLEVEL=5
will enable full
logging output for the general category. Please note that the syntax and
means of setting environment variables varies between operating systems.
Configuration¶
Logs will be saved to destinations as configured by the user. By default,
logging output is saved on the console instance of an application to
hpx.<CATEGORY>.<PID>.lo
(where CATEGORY
and PID>
are placeholders
for the category shortcut and the OS process id). The output for the general
logging category is saved to hpx.<PID>.log
. The default settings for the
general logging category are shown here (the syntax is described in the section
The HPX INI File Format):
[hpx.logging]
level = ${HPX_LOGLEVEL:0}
destination = ${HPX_LOGDESTINATION:console}
format = ${HPX_LOGFORMAT:(T%locality%/%hpxthread%.%hpxphase%/%hpxcomponent%) P%parentloc%/%hpxparent%.%hpxparentphase% %time%($hh:$mm.$ss.$mili) [%idx%]|\\n}
The logging level is taken from the environment variable HPX_LOGLEVEL
and
defaults to zero, e.g. no logging. The default logging destination is read from
the environment variable HPX_LOGDESTINATION
On any of the localities it
defaults to console
which redirects all generated logging output to the
console instance of an application. The following table lists the possible
destinations for any logging output. It is possible to specify more than one
destination separated by whitespace.
Logging destination | Description |
file(<filename> ) |
Direct all output to a file with the given <filename>. |
cout | Direct all output to the local standard output of the application instance on this locality. |
cerr | Direct all output to the local standard error output of the application instance on this locality. |
console | Direct all output to the console instance of the application. The console instance has its logging destinations configured separately. |
android_log | Direct all output to the (Android) system log (available on Android systems only). |
The logging format is read from the environment variable HPX_LOGFORMAT
and
it defaults to a complex format description. This format consists of several
placeholder fields (for instance %locality%
which will be replaced by
concrete values when the logging output is generated. All other information is
transferred verbatim to the output. The table below describes the available
field placeholders. The separator character |
separates the logging message
prefix formatted as shown and the actual log message which will replace the
separator.
Name | Description |
locality | The id of the locality on which the logging message was generated. |
hpxthread | The id of the HPX-thread generating this logging output. |
hpxphase | The phase [1] of the HPX-thread generating this logging output. |
hpxcomponent | The local virtual address of the component which the current HPX-thread is accessing. |
parentloc | The id of the locality where the HPX thread was running which initiated the current HPX-thread. The current HPX-thread is generating this logging output. |
hpxparent | The id of the HPX-thread which initiated the current HPX-thread. The current HPX-thread is generating this logging output. |
hpxparentphase | The phase of the HPX-thread when it initiated the current HPX-thread. The current HPX-thread is generating this logging output. |
time | The time stamp for this logging outputline as generated by the source locality. |
idx | The sequence number of the logging output line as generated on the source locality. |
osthread | The sequence number of the OS-thread which executes the current HPX-thread. |
Note
Not all of the field placeholder may be expanded for all generated logging
output. If no value is available for a particular field it is replaced with a
sequence of '-'
characters.]
Here is an example line from a logging output generated by one of the HPX examples (please note that this is generated on a single line, without line break):
(T00000000/0000000002d46f90.01/00000000009ebc10) P--------/0000000002d46f80.02 17:49.37.320 [000000000000004d]
<info> [RT] successfully created component {0000000100ff0001, 0000000000030002} of type: component_barrier[7(3)]
The default settings for the general logging category on the console is shown here:
[hpx.logging.console]
level = ${HPX_LOGLEVEL:$[hpx.logging.level]}
destination = ${HPX_CONSOLE_LOGDESTINATION:file(hpx.$[system.pid].log)}
format = ${HPX_CONSOLE_LOGFORMAT:|}
These settings define how the logging is customized once the logging output is
received by the console instance of an application. The logging level is read
from the environment variable HPX_LOGLEVEL
(as set for the console instance
of the application). The level defaults to the same values as the corresponding
settings in the general logging configuration shown before. The destination on
the console instance is set to be a file which name is generated based from its
OS process id. Setting the environment variable HPX_CONSOLE_LOGDESTINATION
allows customization of the naming scheme for the output file. The logging
format is set to leave the original logging output unchanged, as received from
one of the localities the application runs on.
HPX Command Line Options¶
The predefined command line options for any application using
hpx::init
are described in the following subsections.
HPX options (allowed on command line only)¶
-
--hpx:help
¶
print out program usage (default: this message), possible values:
full
(additionally prints options from components)
-
--hpx:version
¶
print out HPX version and copyright information
-
--hpx:info
¶
print out HPX configuration information
-
--hpx:options-file
arg
¶ specify a file containing command line options (alternatively: @filepath)
HPX options (additionally allowed in an options file)¶
-
--hpx:worker
¶
run this instance in worker mode
-
--hpx:console
¶
run this instance in console mode
-
--hpx:connect
¶
run this instance in worker mode, but connecting late
-
--hpx:hpx
arg
¶ the IP address the HPX parcelport is listening on, expected format:
address:port
(default:127.0.0.1:7910
)
-
--hpx:agas
arg
¶ the IP address the AGAS root server is running on, expected format:
address:port
(default:127.0.0.1:7910
)
-
--hpx:nodefile
arg
¶ the file name of a node file to use (list of nodes, one node name per line and core)
-
--hpx:nodes
arg
¶ the (space separated) list of the nodes to use (usually this is extracted from a node file)
-
--hpx:endnodes
¶
this can be used to end the list of nodes specified using the option
--hpx:nodes
-
--hpx:ifsuffix
arg
¶ suffix to append to host names in order to resolve them to the proper network interconnect
-
--hpx:ifprefix
arg
¶ prefix to prepend to host names in order to resolve them to the proper network interconnect
-
--hpx:iftransform
arg
¶ sed-style search and replace (
s/search/replace/
) used to transform host names to the proper network interconnect
-
--hpx:localities
arg
¶ the number of localities to wait for at application startup (default:
1
)
-
--hpx:ignore-batch-env
¶
ignore batch environment variables
-
--hpx:expect-connecting-localities
¶
this locality expects other localities to dynamically connect (this is implied if the number of initial localities is larger than 1)
-
--hpx:pu-offset
¶
the first processing unit this instance of HPX should be run on (default:
0
)
-
--hpx:pu-step
¶
the step between used processing unit numbers for this instance of HPX (default:
1
)
-
--hpx:threads
arg
¶ the number of operating system threads to spawn for this HPX locality. Possible values are: numeric values
1
,2
,3
and so on,all
(which spawns one thread per processing unit, includes hyperthreads), orcores
(which spawns one thread per core) (default:cores
).
-
--hpx:cores
arg
¶ the number of cores to utilize for this HPX locality (default:
all
, i.e. the number of cores is based on the number of threads--hpx:threads
assuming--hpx:bind
=compact
-
--hpx:affinity
arg
¶ the affinity domain the OS threads will be confined to, possible values:
pu
,core
,numa
,machine
(default:pu
)
-
--hpx:bind
arg
¶ the detailed affinity description for the OS threads, see More details about HPX command line options for a detailed description of possible values. Do not use with
--hpx:pu-step
,--hpx:pu-offset
or--hpx:affinity
options. Implies--hpx:numa-sensitive
(--hpx:bind
=none
) disables defining thread affinities).
-
--hpx:print-bind
¶
print to the console the bit masks calculated from the arguments specified to all
--hpx:bind
options.
-
--hpx:queuing
arg
¶ the queue scheduling policy to use, options are
local
,local-priority-fifo
,local-priority-lifo
,static
,static-priority
,abp-priority-fifo
andabp-priority-lifo
(default:local-priority-fifo
)
-
--hpx:high-priority-threads
arg
¶ the number of operating system threads maintaining a high priority queue (default: number of OS threads), valid for
--hpx:queuing
=abp-priority
,--hpx:queuing
=static-priority
and--hpx:queuing
=local-priority
only
-
--hpx:numa-sensitive
¶
makes the scheduler NUMA sensitive
HPX configuraton options¶
-
--hpx:app-config
arg
¶ load the specified application configuration (ini) file
-
--hpx:config
arg
¶ load the specified hpx configuration (ini) file
-
--hpx:ini
arg
¶ add a configuration definition to the default runtime configuration
-
--hpx:exit
¶
exit after configuring the runtime
HPX debugging options¶
-
--hpx:list-symbolic-names
¶
list all registered symbolic names after startup
-
--hpx:list-component-types
¶
list all dynamic component types after startup
-
--hpx:dump-config-initial
¶
print the initial runtime configuration
-
--hpx:dump-config
¶
print the final runtime configuration
-
--hpx:debug-hpx-log
[arg]
¶ enable all messages on the HPX log channel and send all HPX logs to the target destination (default:
cout
)
-
--hpx:debug-agas-log
[arg]
¶ enable all messages on the AGAS log channel and send all AGAS logs to the target destination (default:
cout
)
-
--hpx:debug-parcel-log
[arg]
¶ enable all messages on the parcel transport log channel and send all parcel transport logs to the target destination (default:
cout
)
-
--hpx:debug-timing-log
[arg]
¶ enable all messages on the timing log channel and send all timing logs to the target destination (default:
cout
)
-
--hpx:debug-app-log
[arg]
¶ enable all messages on the application log channel and send all application logs to the target destination (default:
cout
)
-
--hpx:debug-clp
¶
debug command line processing
-
--hpx:attach-debugger
arg
¶ wait for a debugger to be attached, possible arg values:
startup
orexception
(default:startup
)
Command line argument shortcuts¶
Additionally, the following shortcuts are available from every HPX application.
Shortcut option | Equivalent long option |
---|---|
-a |
--hpx:agas |
-c |
--hpx:console |
-h |
--hpx:help |
-I |
--hpx:ini |
-l |
--hpx:localities |
-p |
--hpx:app-config |
-q |
--hpx:queuing |
-r |
--hpx:run-agas-server |
-t |
--hpx:threads |
-v |
--hpx:version |
-w |
--hpx:worker |
-x |
--hpx:hpx |
-0 |
--hpx:node =0 |
-1 |
--hpx:node =1 |
-2 |
--hpx:node =2 |
-3 |
--hpx:node =3 |
-4 |
--hpx:node =4 |
-5 |
--hpx:node =5 |
-6 |
--hpx:node =6 |
-7 |
--hpx:node =7 |
-8 |
--hpx:node =8 |
-9 |
--hpx:node =9 |
It is possible to define your own shortcut options. In fact, all of the shortcuts listed above are pre-defined using the technique described here. Also, it is possible to redefine any of the pre-defined shortcuts to expand differently as well.
Shortcut options are obtained from the internal configuration database. They are
stored as key-value properties in a special properties section named
hpx.commandline
. You can define your own shortcuts by adding the
corresponding definitions to one of the ini
configuration files as described
in the section Configuring HPX applications. For instance, in order to define a command
line shortcut --p
which should expand to -hpx:print-counter
, the
following configuration information needs to be added to one of the ini
configuration files:
[hpx.commandline.aliases]
--pc = --hpx:print-counter
Note
Any arguments for shortcut options passed on the command line are retained and passed as arguments to the corresponding expanded option. For instance, given the definition above, the command line option:
--pc=/threads{locality#0/total}/count/cumulative
would be expanded to:
--hpx:print-counter=/threads{locality#0/total}/count/cumulative
Important
Any shortcut option should either start with a single '-'
or with two
'--'
characters. Shortcuts starting with a single '-'
are interpreted
as short options (i.e. everything after the first character following the
'-'
is treated as the argument). Shortcuts starting with '--'
are
interpreted as long options. No other shortcut formats are supported.
Specifying options for single localities only¶
For runs involving more than one locality it is sometimes desirable to
supply specific command line options to single localities only. When the HPX
application is launched using a scheduler (like PBS, for more details see
section How to use HPX applications with PBS), specifying dedicated command line options for single
localities may be desirable. For this reason all of the command line options
which have the general format --hpx:<some_key>
can be used in a more general
form: --hpx:<N>:<some_key>
, where <N> is the number of the locality
this command line options will be applied to, all other localities will simply
ignore the option. For instance, the following PBS script passes the option
--hpx:pu-offset
=4
to the locality '1'
only.
#!/bin/bash
#
#PBS -l nodes=2:ppn=4
APP_PATH=~/packages/hpx/bin/hello_world_distributed
APP_OPTIONS=
pbsdsh -u $APP_PATH $APP_OPTIONS --hpx:1:pu-offset=4 --hpx:nodes=`cat $PBS_NODEFILE`
Caution
If the first application specific argument (inside $APP_OPTIONS
is a
non-option (i.e. does not start with a -
or a --
, then it must be
placed before the option --hpx:nodes
, which, in this case,
should be the last option on the command line.
Alternatively, use the option --hpx:endnodes
to explicitly
mark the end of the list of node names:
pbsdsh -u $APP_PATH --hpx:1:pu-offset=4 --hpx:nodes=`cat $PBS_NODEFILE` --hpx:endnodes $APP_OPTIONS
More details about HPX command line options¶
This section documents the following list of the command line options in more detail:
--hpx:bind
¶This command line option allows one to specify the required affinity of the
HPX worker threads to the underlying processing units. As a result the worker
threads will run only on the processing units identified by the corresponding
bind specification. The affinity settings are to be specified using
--hpx:bind
=<BINDINGS>
, where <BINDINGS>
have to be formatted as
described below.
In addition to the syntax described below one can use --hpx:bind
=none
to disable all binding of any threads to a particular core. This is
mostly supported for debugging purposes.
The specified affinities refer to specific regions within a machine hardware topology. In order to understand the hardware topology of a particular machine it may be useful to run the lstopo tool which is part of Portable Hardware Locality (HWLOC) to see the reported topology tree. Seeing and understanding a topology tree will definitely help in understanding the concepts that are discussed below.
Affinities can be specified using HWLOC (Portable Hardware Locality (HWLOC)) tuples. Tuples of HWLOC
objects and associated indexes can be specified in the form
object:index
, object:index-index
or object:index,...,index
. HWLOC
objects represent types of mapped items in a topology tree. Possible values for
objects are socket
, numanode
, core
and pu
(processing unit).
Indexes are non-negative integers that specify a unique physical object in a
topology tree using its logical sequence number.
Chaining multiple tuples together in the more general form
object1:index1[.object2:index2[...]]
is permissible. While the first tuple’s
object may appear anywhere in the topology, the Nth tuple’s object must have a
shallower topology depth than the (N+1)th tuple’s object. Put simply: as you
move right in a tuple chain, objects must go deeper in the topology tree.
Indexes specified in chained tuples are relative to the scope of the parent
object. For example, socket:0.core:1
refers to the second core in the first
socket (all indices are zero based).
Multiple affinities can be specified using several --hpx:bind
command
line options or by appending several affinities separated by a ';'
By
default, if multiple affinities are specified, they are added.
"all"
is a special affinity consisting in the entire current topology.
Note
All ‘names’ in an affinity specification, such as thread
, socket
,
numanode
, pu
or all
can be abbreviated. Thus the affinity
specification threads:0-3=socket:0.core:1.pu:1
is fully equivalent to its
shortened form t:0-3=s:0.c:1.p:1
.
Here is a full grammar describing the possible format of mappings:
mappings ::=distribution
|mapping
(";"mapping
)* distribution ::= "compact" | "scatter" | "balanced" | "numa-balanced" mapping ::=thread_spec
"="pu_specs
thread_spec ::= "thread:"range_specs
pu_specs ::=pu_spec
("."pu_spec
)* pu_spec ::=type
":"range_specs
| "~"pu_spec
range_specs ::=range_spec
(","range_spec
)* range_spec ::= int | int "-" int | "all" type ::= "socket" | "numanode" | "core" | "pu"
The following example assumes a system with at least 4 cores, where each core
has more than 1 processing unit (hardware threads). Running
hello_world_distributed
with 4 OS-threads (on 4 processing units), where
each of those threads is bound to the first processing unit of each of the
cores, can be achieved by invoking:
hello_world_distributed -t4 --hpx:bind=thread:0-3=core:0-3.pu:0
Here thread:0-3
specifies the OS threads for which to define affinity
bindings, and core:0-3.pu:
defines that for each of the cores (core:0-3
)
only their first processing unit pu:0
should be used.
Note
The command line option --hpx:print-bind
can be used to print the
bitmasks generated from the affinity mappings as specified with
--hpx:bind
. For instance, on a system with hyperthreading enabled
(i.e. 2 processing units per core), the command line:
hello_world_distributed -t4 --hpx:bind=thread:0-3=core:0-3.pu:0 --hpx:print-bind
will cause this output to be printed:
0: PU L#0(P#0), Core L#0, Socket L#0, Node L#0(P#0)
1: PU L#2(P#2), Core L#1, Socket L#0, Node L#0(P#0)
2: PU L#4(P#4), Core L#2, Socket L#0, Node L#0(P#0)
3: PU L#6(P#6), Core L#3, Socket L#0, Node L#0(P#0)
where each bit in the bitmasks corresponds to a processing unit the listed worker thread will be bound to run on.
The difference between the four possible predefined distribution schemes
(compact
, scatter
, balanced
and numa-balanced
) is best explained
with an example. Imagine that we have a system with 4 cores and 4 hardware
threads per core on 2 sockets. If we place 8 threads the assignments produced by
the compact
, scatter
, balanced
and numa-balanced
types are shown
in the figure below. Notice that compact
does not fully utilize all the
cores in the system. For this reason it is recommended that applications are run
using the scatter
or balanced
/numa-balanced
options in most cases.
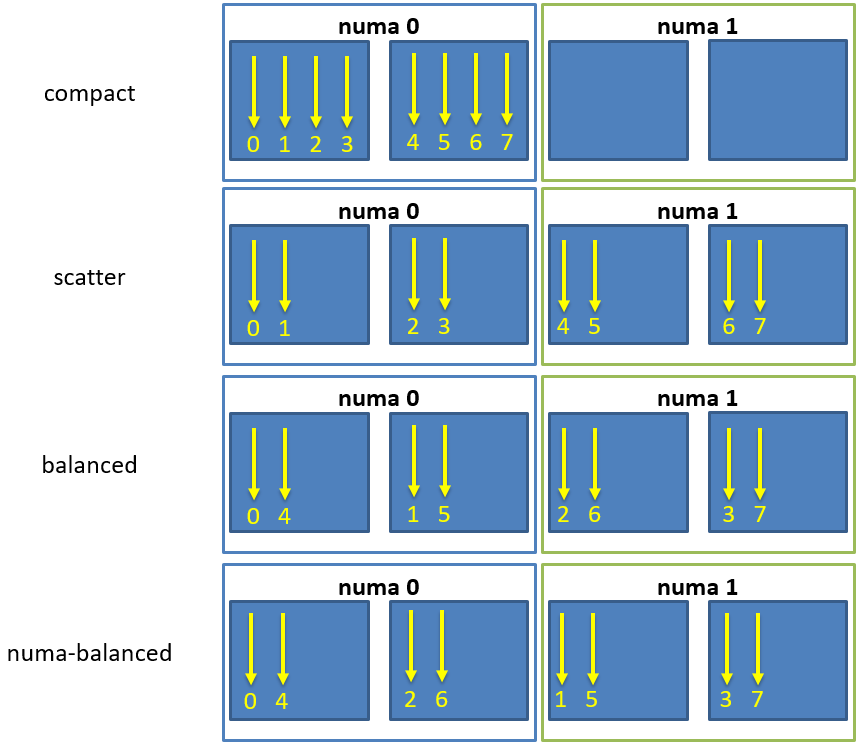
Fig. 7 Schematic of thread affinity type distributions.
[1] | The phase of a HPX-thread counts how often this thread has been activated. |
Writing single-node HPX applications¶
HPX is a C++ Standard Library for Concurrency and Parallelism. This means that it implements all of the corresponding facilities as defined by the C++ Standard. Additionally, in HPX we implement functionalities proposed as part of the ongoing C++ standardization process. This section focuses on the features available in HPX for parallel and concurrent computation on a single node, although many of the features presented here are also implemented to work in the distributed case.
Using LCOs¶
Lightweight Control Objects provide synchronization for HPX applications. Most of them are familiar from other frameworks, but a few of them work in slightly special different ways adapted to HPX.
future
queue
object_semaphore
barrier
Channels¶
Channels combine communication (the exchange of a value) with synchronization (guaranteeing that two calculations (tasks) are in a known state). A channel can transport any number of values of a given type from a sender to a receiver:
hpx::lcos::local::channel<int> c;
c.set(42);
cout << c.get(); // will print '42'
Channels can be handed to another thread (or in case of channel components, to other localities), thus establishing a communication channel between two independent places in the program:
void do_something(
hpx::lcos::local::receive_channel<int> c,
hpx::lcos::local::send_channel<> done)
{
cout << c.get(); // prints 42
done.set(); // signal back
}
{
hpx::lcos::local::channel<int> c;
hpx::lcos::local::channel<> done;
hpx::apply(&do_something, c, done);
c.set(42); // send some value
done.get(); // wait for thread to be done
}
A channel component is created on one locality and can be send to another locality using an action. This example also demonstrates how a channel can be used as a range of values:
// channel components need to be registered for each used type (not needed
// for hpx::lcos::local::channel)
HPX_REGISTER_CHANNEL(double);
void some_action(hpx::lcos::channel<double> c)
{
for (double d : c)
hpx::cout << d << std::endl;
}
HPX_REGISTER_ACTION(some_action);
{
// create the channel on this locality
hpx::lcos::channel<double> c(hpx::find_here());
// pass the channel to a (possibly remote invoked) action
hpx::apply(some_action(), hpx::find_here(), c);
// send some values to the receiver
std::vector<double> v = { 1.2, 3.4, 5.0 };
for (double d : v)
c.set(d);
// explicitly close the communication channel (implicit at destruction)
c.close();
}
Composable guards¶
Composable guards operate in a manner similar to locks, but are applied only to asynchronous functions. The guard (or guards) is automatically locked at the beginning of a specified task and automatically unlocked at the end. Because guards are never added to an existing task’s execution context, the calling of guards is freely composable and can never deadlock.
To call an application with a single guard, simply declare the guard and call run_guarded() with a function (task):
hpx::lcos::local::guard gu;
run_guarded(gu,task);
If a single method needs to run with multiple guards, use a guard set:
boost::shared<hpx::lcos::local::guard> gu1(new hpx::lcos::local::guard());
boost::shared<hpx::lcos::local::guard> gu2(new hpx::lcos::local::guard());
gs.add(*gu1);
gs.add(*gu2);
run_guarded(gs,task);
Guards use two atomic operations (which are not called repeatedly) to manage what they do, so overhead should be extremely low.
conditional_trigger
counting_semaphore
dataflow
event
mutex
once
recursive_mutex
spinlock
spinlock_no_backoff
trigger
Extended facilities for futures¶
Concurrency is about both decomposing and composing the program from the parts that work well individually and together. It is in the composition of connected and multicore components where today’s C++ libraries are still lacking.
The functionality of std::future
offers a partial solution. It allows for
the separation of the initiation of an operation and the act of waiting for its
result; however the act of waiting is synchronous. In communication-intensive
code this act of waiting can be unpredictable, inefficient and simply
frustrating. The example below illustrates a possible synchronous wait using
futures:
#include <future>
using namespace std;
int main()
{
future<int> f = async([]() { return 123; });
int result = f.get(); // might block
}
For this reason, HPX implements a set of extensions to std::future
(as
proposed by __cpp11_n4107__). This proposal introduces the following key
asynchronous operations to hpx::future
, hpx::shared_future
and
hpx::async
, which enhance and enrich these facilities.
Facility | Description |
hpx::future::then |
In asynchronous programming, it is very common for one asynchronous operation, on completion, to invoke a second operation and pass data to it. The current C++ standard does not allow one to register a continuation to a future. With``then`` instead of waiting for the result, a continuation is “attached” to the asynchronous operation, which is invoked when the result is ready. Continuations registered using then function will help to avoid blocking waits or wasting threads on polling, greatly improving the responsiveness and scalability of an application. |
unwrapping constructor for hpx::future |
In some scenarios, you might want to create a future that returns another future, resulting in nested futures. Although it is possible to write code to unwrap the outer future and retrieve the nested future and its result, such code is not easy to write because you must handle exceptions and it may cause a blocking call. Unwrapping can allow us to mitigate this problem by doing an asynchronous call to unwrap the outermost future. |
hpx::future::is_ready |
There are often situations where a get() call on a future may not be
a blocking call, or is only a blocking call under certain circumstances.
This function gives the ability to test for early completion and allows
us to avoid associating a continuation, which needs to be scheduled with
some non-trivial overhead and near-certain loss of cache efficiency. |
hpx::make_ready_future |
Some functions may know the value at the point of construction. In these cases the value is immediately available, but needs to be returned as a future. By using``hpx::make_ready_future``a future can be created which holds a pre-computed result in its shared state. In the current standard it is non-trivial to create a future directly from a value. First a promise must be created, then the promise is set, and lastly the future is retrieved from the promise. This can now be done with one operation. |
The standard also omits the ability to compose multiple futures. This is a common pattern that is ubiquitous in other asynchronous frameworks and is absolutely necessary in order to make C++ a powerful asynchronous programming language. Not including these functions is synonymous to Boolean algebra without AND/OR.
In addition to the extensions proposed by N4313, HPX adds functions allowing to compose several futures in a more flexible way.
Facility | Description | Comment |
hpx::when_any , hpx::when_any_n |
Asynchronously wait for at least one of multiple future or shared_future objects to finish. | N4313, ..._n versions are HPX only |
hpx::wait_any , hpx::wait_any_n |
Synchronously wait for at least one of multiple future or shared_future objects to finish. | HPX only |
hpx::when_all , hpx::when_all_n |
Asynchronously wait for all future and shared_future objects to finish. | N4313, ..._n versions are HPX only |
hpx::wait_all , hpx::wait_all_n |
Synchronously wait for all future and shared_future objects to finish. | HPX only |
hpx::when_some , hpx::when_some_n |
Asynchronously wait for multiple future and shared_future objects to finish. | HPX only |
hpx::wait_some , hpx::wait_some_n |
Synchronously wait for multiple future and shared_future objects to finish. | HPX only |
hpx::when_each |
Asynchronously wait for multiple future and shared_future objects to finish and call a function for each of the future objects as soon as it becomes ready. | HPX only |
hpx::wait_each , hpx::wait_each_n |
Synchronously wait for multiple future and shared_future objects to finish and call a function for each of the future objects as soon as it becomes ready. | HPX only |
High level parallel facilities¶
In preparation for the upcoming C++ Standards we currently see several proposals targeting different facilities supporting parallel programming. HPX implements (and extends) some of those proposals. This is well aligned with our strategy to align the APIs exposed from HPX with current and future C++ Standards.
At this point, HPX implements several of the C++ Standardization working papers, most notably N4409 (Working Draft, Technical Specification for C++ Extensions for Parallelism), N4411 (Task Blocks), and N4406 (Parallel Algorithms Need Executors).
Using parallel algorithms¶
A parallel algorithm is a function template described by this document
which is declared in the (inline) namespace hpx::parallel::v1
.
Note
For compilers which do not support inline namespaces, all of the namespace
v1
is imported into the namespace hpx::parallel
. The effect is similar
to what inline namespaces would do, namely all names defined in
hpx::parallel::v1
are accessible from the namespace hpx::parallel
as
well.
All parallel algorithms are very similar in semantics to their sequential
counterparts (as defined in the namespace std
with an additional formal
template parameter named ExecutionPolicy
. The execution policy is generally
passed as the first argument to any of the parallel algorithms and describes the
manner in which the execution of these algorithms may be parallelized and the
manner in which they apply user-provided function objects.
The applications of function objects in parallel algorithms invoked with an
execution policy object of type hpx::parallel::execution::sequenced_policy
or
hpx::parallel::execution::sequenced_task_policy
execute in sequential order. For
hpx::parallel::execution::sequenced_policy
the execution happens in the calling thread.
The applications of function objects in parallel algorithms invoked with an
execution policy object of type hpx::parallel::execution::parallel_policy
or
hpx::parallel::execution::parallel_task_policy
are permitted to execute in an unordered
fashion in unspecified threads, and indeterminately sequenced within each
thread.
Important
It is the caller’s responsibility to ensure correctness, for example that the invocation does not introduce data races or deadlocks.
The applications of function objects in parallel algorithms invoked with an
execution policy of type hpx::parallel::execution::parallel_unsequenced_policy
is in HPX
equivalent to the use of the execution policy hpx::parallel::execution::parallel_policy
.
Algorithms invoked with an execution policy object of type hpx::parallel::v1::execution_policy
execute internally as if invoked with the contained execution policy object. No
exception is thrown when an hpx::parallel::v1::execution_policy
contains an execution policy of
type hpx::parallel::execution::sequenced_task_policy
or hpx::parallel::execution::parallel_task_policy
(which normally turn the algorithm into its asynchronous version). In this case
the execution is semantically equivalent to the case of passing a
hpx::parallel::execution::sequenced_policy
or hpx::parallel::execution::parallel_policy
contained in the
hpx::parallel::v1::execution_policy
object respectively.
Parallel exceptions¶
During the execution of a standard parallel algorithm, if temporary memory
resources are required by any of the algorithms and no memory are available, the
algorithm throws a std::bad_alloc
exception.
During the execution of any of the parallel algorithms, if the application of a function object terminates with an uncaught exception, the behavior of the program is determined by the type of execution policy used to invoke the algorithm:
- If the execution policy object is of type
hpx::parallel::execution::parallel_unsequenced_policy
,hpx::terminate
shall be called. - If the execution policy object is of type
hpx::parallel::execution::sequenced_policy
,hpx::parallel::execution::sequenced_task_policy
,hpx::parallel::execution::parallel_policy
, orhpx::parallel::execution::parallel_task_policy
the execution of the algorithm terminates with anhpx::exception_list
exception. All uncaught exceptions thrown during the application of user-provided function objects shall be contained in thehpx::exception_list
For example, the number of invocations of the user-provided function object in
for_each is unspecified. When hpx::parallel::v1::for_each
is executed sequentially, only one
exception will be contained in the hpx::exception_list
object.
These guarantees imply that, unless the algorithm has failed to allocate memory
and terminated with std::bad_alloc
all exceptions thrown during the execution
of the algorithm are communicated to the caller. It is unspecified whether an
algorithm implementation will “forge ahead” after encountering and capturing a
user exception.
The algorithm may terminate with the std::bad_alloc
exception even if one or
more user-provided function objects have terminated with an exception. For
example, this can happen when an algorithm fails to allocate memory while
creating or adding elements to the hpx::exception_list
object.
Parallel algorithms¶
HPX provides implementations of the following parallel algorithms:
Name | Description | In header | Algorithm page at cppreference.com |
hpx::parallel::v1::adjacent_find |
Computes the differences between adjacent elements in a range. | <hpx/include/parallel_adjacent_find.hpp> |
adjacent_find |
hpx::parallel::v1::all_of |
Checks if a predicate is true for all of the elements in a range. |
<hpx/include/parallel_all_any_none.hpp> |
all_any_none_of |
hpx::parallel::v1::any_of |
Checks if a predicate is true for any of the elements in a range. |
<hpx/include/parallel_all_any_none.hpp> |
all_any_none_of |
hpx::parallel::v1::count |
Returns the number of elements equal to a given value. | <hpx/include/parallel_count.hpp> |
count |
hpx::parallel::v1::count_if |
Returns the number of elements satisfying a specific criteria. | <hpx/include/parallel_count.hpp> |
count_if |
hpx::parallel::v1::equal |
Determines if two sets of elements are the same. | <hpx/include/parallel_equal.hpp> |
equal |
hpx::parallel::v1::exclusive_scan |
Does an exclusive parallel scan over a range of elements. | <hpx/include/parallel_scan.hpp> |
exclusive_scan |
hpx::parallel::v1::find |
Finds the first element equal to a given value. | <hpx/include/parallel_find.hpp> |
find |
hpx::parallel::v1::find_end |
Finds the last sequence of elements in a certain range. | <hpx/include/parallel_find.hpp> |
find_end |
hpx::parallel::v1::find_first_of |
Searches for any one of a set of elements. | <hpx/include/parallel_find.hpp> |
find_first_of |
hpx::parallel::v1::find_if |
Finds the first element satisfying a specific criteria. | <hpx/include/parallel_find.hpp> |
find |
hpx::parallel::v1::find_if_not |
Finds the first element not satisfying a specific criteria. | <hpx/include/parallel_find.hpp> |
find_if_not |
hpx::parallel::v1::for_each |
Applies a function to a range of elements. | <hpx/include/parallel_for_each.hpp> |
for_each |
hpx::parallel::v1::for_each_n |
Applies a function to a number of elements. | <hpx/include/parallel_for_each.hpp> |
for_each_n |
hpx::parallel::v1::inclusive_scan |
Does an inclusive parallel scan over a range of elements. | <hpx/include/parallel_scan.hpp> |
inclusive_scan |
hpx::parallel::v1::lexicographical_compare |
Checks if a range of values is lexicographically less than another range of values. | <hpx/include/parallel_lexicographical_compare.hpp> |
lexicographical_compare |
hpx::parallel::v1::mismatch |
Finds the first position where two ranges differ. | <hpx/include/parallel_mismatch.hpp> |
mismatch |
hpx::parallel::v1::none_of |
Checks if a predicate is true for none of the elements in a range. |
<hpx/include/parallel_all_any_none.hpp> |
all_any_none_of |
hpx::parallel::v1::search |
Searches for a range of elements. | <hpx/include/parallel_search.hpp> |
search |
hpx::parallel::v1::search_n |
Searches for a number consecutive copies of an element in a range. | <hpx/include/parallel_search.hpp> |
search_n |
Name | Description | In header | Algorithm page at cppreference.com |
hpx::parallel::v1::copy |
Copies a range of elements to a new location. | <hpx/include/parallel_copy.hpp> |
exclusive_scan |
hpx::parallel::v1::copy_n |
Copies a number of elements to a new location. | <hpx/include/parallel_copy.hpp> |
copy_n |
hpx::parallel::v1::copy_if |
Copies the elements from a range to a new location for which the given predicate is true |
<hpx/include/parallel_copy.hpp> |
copy |
hpx::parallel::v1::move |
Moves a range of elements to a new location. | <hpx/include/parallel_fill.hpp> |
move |
hpx::parallel::v1::fill |
Assigns a range of elements a certain value. | <hpx/include/parallel_fill.hpp> |
fill |
hpx::parallel::v1::fill_n |
Assigns a value to a number of elements. | <hpx/include/parallel_fill.hpp> |
fill_n |
hpx::parallel::v1::generate |
Saves the result of a function in a range. | <hpx/include/parallel_generate.hpp> |
generate |
hpx::parallel::v1::generate_n |
Saves the result of N applications of a function. | <hpx/include/parallel_generate.hpp> |
generate_n |
hpx::parallel::v1::remove |
Removes the elements from a range that are equal to the given value. | <hpx/include/parallel_remove.hpp> |
remove |
hpx::parallel::v1::remove_if |
Removes the elements from a range that are equal to the given predicate is false |
<hpx/include/parallel_remove.hpp> |
remove |
hpx::parallel::v1::remove_copy |
Copies the elements from a range to a new location that are not equal to the given value. | <hpx/include/parallel_remove_copy.hpp> |
remove_copy |
hpx::parallel::v1::remove_copy_if |
Copies the elements from a range to a new location for which the given predicate is false |
<hpx/include/parallel_remove_copy.hpp> |
remove_copy |
hpx::parallel::v1::replace |
Replaces all values satisfying specific criteria with another value. | <hpx/include/parallel_replace.hpp> |
replace |
hpx::parallel::v1::replace_if |
Replaces all values satisfying specific criteria with another value. | <hpx/include/parallel_replace.hpp> |
replace |
hpx::parallel::v1::replace_copy |
Copies a range, replacing elements satisfying specific criteria with another value. | <hpx/include/parallel_replace.hpp> |
replace_copy |
hpx::parallel::v1::replace_copy_if |
Copies a range, replacing elements satisfying specific criteria with another value. | <hpx/include/parallel_replace.hpp> |
replace_copy |
hpx::parallel::v1::reverse |
Reverses the order elements in a range. | <hpx/include/parallel_reverse.hpp> |
reverse |
hpx::parallel::v1::reverse_copy |
Creates a copy of a range that is reversed. | <hpx/include/parallel_reverse.hpp> |
reverse_copy |
hpx::parallel::v1::rotate |
Rotates the order of elements in a range. | <hpx/include/parallel_rotate.hpp> |
rotate |
hpx::parallel::v1::rotate_copy |
Copies and rotates a range of elements. | <hpx/include/parallel_rotate.hpp> |
rotate_copy |
hpx::parallel::v1::swap_ranges |
Swaps two ranges of elements. | <hpx/include/parallel_swap_ranges.hpp> |
swap_ranges |
hpx::parallel::v1::transform |
Applies a function to a range of elements. | <hpx/include/parallel_transform.hpp> |
transform |
hpx::parallel::v1::unique_copy |
Eliminates all but the first element from every consecutive group of equivalent elements from a range. | <hpx/include/parallel_unique.hpp> |
unique |
hpx::parallel::v1::unique_copy |
Eliminates all but the first element from every consecutive group of equivalent elements from a range. | <hpx/include/parallel_unique.hpp> |
unique_copy |
Name | Description | In header | Algorithm page at cppreference.com |
hpx::parallel::v1::merge |
Merges two sorted ranges. | <hpx/include/parallel_merge.hpp> |
merge |
hpx::parallel::v1::inplace_merge |
Merges two ordered ranges in-place. | <hpx/include/parallel_merge.hpp> |
inplace_merge |
hpx::parallel::v1::includes |
Returns true if one set is a subset of another. | <hpx/include/parallel_set_operations.hpp> |
includes |
hpx::parallel::v1::set_difference |
Computes the difference between two sets. | <hpx/include/parallel_set_operations.hpp> |
set_difference |
hpx::parallel::v1::set_intersection |
Computes the intersection of two sets. | <hpx/include/parallel_set_operations.hpp> |
set_intersection |
hpx::parallel::v1::set_symmetric_difference |
Computes the symmetric difference between two sets. | <hpx/include/parallel_set_operations.hpp> |
set_symmetric_difference |
hpx::parallel::v1::set_union |
Computes the union of two sets. | <hpx/include/parallel_set_operations.hpp> |
set_union |
Name | Description | In header | Algorithm page at cppreference.com |
hpx::parallel::v1::is_heap |
Returns true if the range is max heap. |
<hpx/include/is_heap.hpp> |
is_heap |
hpx::parallel::v1::is_heap_until |
Returns the first element that breaks a max heap. | <hpx/include/is_heap.hpp> |
is_heap_until |
Name | Description | In header | Algorithm page at cppreference.com |
hpx::parallel::v1::max_element |
Returns the largest element in a range. | <hpx/include/parallel_minmax.hpp> |
max_element |
hpx::parallel::v1::min_element |
Returns the smallest element in a range. | <hpx/include/parallel_minmax.hpp> |
min_element |
hpx::parallel::v1::minmax_element |
Returns the smallest and the largest element in a range. | <hpx/include/parallel_minmax.hpp> |
minmax_element |
Name | Description | In header | Algorithm page at cppreference.com |
hpx::parallel::v1::is_partitioned |
Returns true if each true element for a predicate precedes the false elements in a range |
<hpx/include/parallel_is_partitioned.hpp> |
is_partitioned |
hpx::parallel::v1::partition |
Divides elements into two groups while don’t preserve their relative order | <hpx/include/parallel_partition.hpp> |
partition |
hpx::parallel::v1::partition_copy |
Copies a range dividing the elements into two groups | <hpx/include/parallel_partition.hpp> |
partition_copy |
hpx::parallel::v1::stable_partition |
Divides elements into two groups while preserving their relative order | <hpx/include/parallel_partition.hpp> |
stable_partition |
Name | Description | In header | Algorithm page at cppreference.com |
hpx::parallel::v1::is_sorted |
Returns true if each element in a range is sorted |
<hpx/include/parallel_is_sorted.hpp> |
is_sorted |
hpx::parallel::v1::is_sorted_until |
Returns the first unsorted element | <hpx/include/parallel_is_sorted.hpp> |
is_sorted_until |
hpx::parallel::v1::sort |
Sorts the elements in a range | <hpx/include/parallel_sort.hpp> |
sort |
hpx::parallel::v1::sort_by_key |
Sorts one range of data using keys supplied in another range | <hpx/include/parallel_sort.hpp> |
Name | Description | In header | Algorithm page at cppreference.com |
hpx::parallel::v1::adjacent_difference |
Calculates the difference between each element in an input range and the preceding element. | <hpx/include/parallel_adjacent_difference.hpp> |
adjacent_difference |
hpx::parallel::v1::reduce |
Sums up a range of elements. | <hpx/include/parallel_reduce.hpp> |
reduce |
hpx::parallel::v1::reduce_by_key |
Performs an inclusive scan on consecutive elements with matching keys,
with a reduction to output only the final sum for each key. The key
sequence {1,1,1,2,3,3,3,3,1} and value sequence
{2,3,4,5,6,7,8,9,10} would be reduced to keys={1,2,3,1} ,
values={9,5,30,10} |
<hpx/include/parallel_reduce.hpp> |
|
hpx::parallel::v1::transform_reduce |
Sums up a range of elements after applying a function. Also, accumulates the inner products of two input ranges. | <hpx/include/parallel_transform_reduce.hpp> |
transform_reduce |
hpx::parallel::v1::transform_inclusive_scan |
Does an inclusive parallel scan over a range of elements after applying a function. | <hpx/include/parallel_scan.hpp> |
transform_inclusive_scan |
hpx::parallel::v1::transform_exclusive_scan |
Does an exclusive parallel scan over a range of elements after applying a function. | <hpx/include/parallel_scan.hpp> |
transform_exclusive_scan |
Name | Description | In header | Algorithm page at cppreference.com |
hpx::parallel::v1::destroy |
Destroys a range of objects. | <hpx/include/parallel_destroy.hpp> |
destroy |
hpx::parallel::v1::destroy_n |
Destroys a range of objects. | <hpx/include/parallel_destroy.hpp> |
destroy_n |
hpx::parallel::v1::uninitialized_copy |
Copies a range of objects to an uninitialized area of memory. | <hpx/include/parallel_uninitialized_copy.hpp> |
uninitialized_copy |
hpx::parallel::v1::uninitialized_copy_n |
Copies a number of objects to an uninitialized area of memory. | <hpx/include/parallel_uninitialized_copy.hpp> |
uninitialized_copy_n |
hpx::parallel::v1::uninitialized_default_construct |
Copies a range of objects to an uninitialized area of memory. | <hpx/include/parallel_uninitialized_default_construct.hpp> |
uninitialized_default_construct |
hpx::parallel::v1::uninitialized_default_construct_n |
Copies a number of objects to an uninitialized area of memory. | <hpx/include/parallel_uninitialized_default_construct.hpp> |
uninitialized_default_construct_n |
hpx::parallel::v1::uninitialized_fill |
Copies an object to an uninitialized area of memory. | <hpx/include/parallel_uninitialized_fill.hpp> |
uninitialized_fill |
hpx::parallel::v1::uninitialized_fill_n |
Copies an object to an uninitialized area of memory. | <hpx/include/parallel_uninitialized_fill.hpp> |
uninitialized_fill_n |
hpx::parallel::v1::uninitialized_move |
Moves a range of objects to an uninitialized area of memory. | <hpx/include/parallel_uninitialized_move.hpp> |
uninitialized_move |
hpx::parallel::v1::uninitialized_move_n |
Moves a number of objects to an uninitialized area of memory. | <hpx/include/parallel_uninitialized_move.hpp> |
uninitialized_move_n |
hpx::parallel::v1::uninitialized_value_construct |
Constructs objects in an uninitialized area of memory. | <hpx/include/parallel_uninitialized_value_construct.hpp> |
uninitialized_value_construct |
hpx::parallel::v1::uninitialized_value_construct_n |
Constructs objects in an uninitialized area of memory. | <hpx/include/uninitialized_value_construct.hpp> |
uninitialized_value_construct_n |
Name | Description | In header |
hpx::parallel::v2::for_loop |
Implements loop functionality over a range specified by integral or iterator bounds. | <hpx/include/parallel_for_loop.hpp> |
hpx::parallel::v2::for_loop_strided |
Implements loop functionality over a range specified by integral or iterator bounds. | <hpx/include/parallel_for_loop.hpp> |
hpx::parallel::v2::for_loop_n |
Implements loop functionality over a range specified by integral or iterator bounds. | <hpx/include/parallel_for_loop.hpp> |
hpx::parallel::v2::for_loop_n_strided |
Implements loop functionality over a range specified by integral or iterator bounds. | <hpx/include/parallel_for_loop.hpp> |
Executor parameters and executor parameter traits¶
In HPX we introduce the notion of execution parameters and execution parameter traits. At this point, the only parameter which can be customized is the size of the chunks of work executed on a single HPX-thread (such as the number of loop iterations combined to run as a single task).
An executor parameter object is responsible for exposing the calculation of the size of the chunks scheduled. It abstracts the (potential platform-specific) algorithms of determining those chunks sizes.
The way executor parameters are implemented is aligned with the way executors
are implemented. All functionalities of concrete executor parameter types are
exposed and accessible through a corresponding
hpx::parallel::executor_parameter_traits
type.
With executor_parameter_traits
clients access all types of executor
parameters uniformly:
std::size_t chunk_size =
executor_parameter_traits<my_parameter_t>::get_chunk_size(my_parameter,
my_executor, [](){ return 0; }, num_tasks);
This call synchronously retrieves the size of a single chunk of loop iterations
(or similar) to combine for execution on a single HPX-thread if the overall
number of tasks to schedule is given by num_tasks
. The lambda function
exposes a means of test-probing the execution of a single iteration for
performance measurement purposes (the execution parameter type might dynamically
determine the execution time of one or more tasks in order to calculate the
chunk size, see hpx::parallel::execution::auto_chunk_size
for an
example of such a executor parameter type).
Other functions in the interface exist to discover whether a executor parameter
type should be invoked once (i.e. returns a static chunk size, see
hpx::parallel::execution::static_chunk_size
) or whether it should
be invoked for each scheduled chunk of work (i.e. it returns a variable chunk
size, for an example, see
hpx::parallel::execution::guided_chunk_size
).
Though this interface appears to require executor parameter type authors to implement all different basic operations, there is really none required. In practice, all operations have sensible defaults. However, some executor parameter types will naturally specialize all operations for maximum efficiency.
In HPX we have implemented the following executor parameter types:
hpx::parallel::execution::auto_chunk_size
: Loop iterations are divided into pieces and then assigned to threads. The number of loop iterations combined is determined based on measurements of how long the execution of 1% of the overall number of iterations takes. This executor parameters type makes sure that as many loop iterations are combined as necessary to run for the amount of time specified.hpx::parallel::execution::static_chunk_size
: Loop iterations are divided into pieces of a given size and then assigned to threads. If the size is not specified, the iterations are evenly (if possible) divided contiguously among the threads. This executor parameters type is equivalent to OpenMP’s STATIC scheduling directive.hpx::parallel::execution::dynamic_chunk_size
: Loop iterations are divided into pieces of a given size and then dynamically scheduled among the cores; when an core finishes one chunk, it is dynamically assigned another If the size is not specified, the default chunk size is 1. This executor parameters type is equivalent to OpenMP’s DYNAMIC scheduling directive.hpx::parallel::execution::guided_chunk_size
: Iterations are dynamically assigned to cores in blocks as cores request them until no blocks remain to be assigned. Similar todynamic_chunk_size
except that the block size decreases each time a number of loop iterations is given to a thread. The size of the initial block is proportional tonumber_of_iterations / number_of_cores
. Subsequent blocks are proportional tonumber_of_iterations_remaining / number_of_cores
. The optional chunk size parameter defines the minimum block size. The default minimal chunk size is 1. This executor parameters type is equivalent to OpenMP’s GUIDED scheduling directive.
Using task blocks¶
The define_task_block
, run
and the wait
functions implemented based
on N4411 are based on the task_block
concept that is a part of the
common subset of the Microsoft Parallel Patterns Library (PPL) and the Intel Threading Building Blocks (TBB) libraries.
This implementations adopts a simpler syntax than exposed by those libraries— one that is influenced by language-based concepts such as spawn and sync from Cilk++ and async and finish from X10. It improves on existing practice in the following ways:
- The exception handling model is simplified and more consistent with normal C++ exceptions.
- Most violations of strict fork-join parallelism can be enforced at compile time (with compiler assistance, in some cases).
- The syntax allows scheduling approaches other than child stealing.
Consider an example of a parallel traversal of a tree, where a user-provided function compute is applied to each node of the tree, returning the sum of the results:
template <typename Func>
int traverse(node& n, Func && compute)
{
int left = 0, right = 0;
define_task_block(
[&](task_block<>& tr) {
if (n.left)
tr.run([&] { left = traverse(*n.left, compute); });
if (n.right)
tr.run([&] { right = traverse(*n.right, compute); });
});
return compute(n) + left + right;
}
The example above demonstrates the use of two of the functions,
hpx::parallel::define_task_block
and the
hpx::parallel::task_block::run
member function of a
hpx::parallel::task_block
.
The task_block
function delineates a region in a program code potentially
containing invocations of threads spawned by the run
member function of the
task_block
class. The run
function spawns an HPX thread, a unit of
work that is allowed to execute in parallel with respect to the caller. Any
parallel tasks spawned by run
within the task block are joined back to a
single thread of execution at the end of the define_task_block
. run
takes a user-provided function object f
and starts it asynchronously—i.e.
it may return before the execution of f
completes. The HPX scheduler may
choose to run f
immediately or delay running f
until compute resources
become available.
A task_block
can be constructed only by define_task_block
because it has
no public constructors. Thus, run
can be invoked (directly or indirectly)
only from a user-provided function passed to define_task_block
:
void g();
void f(task_block<>& tr)
{
tr.run(g); // OK, invoked from within task_block in h
}
void h()
{
define_task_block(f);
}
int main()
{
task_block<> tr; // Error: no public constructor
tr.run(g); // No way to call run outside of a define_task_block
return 0;
}
Extensions for task blocks¶
In HPX we implemented some extensions for task_block
beyond the actual
standards proposal N4411. The main addition is that a task_block
can be invoked with a execution policy as its first argument, very similar to
the parallel algorithms.
An execution policy is an object that expresses the requirements on the
ordering of functions invoked as a consequence of the invocation of a
task block. Enabling passing an execution policy to define_task_block
gives the user control over the amount of parallelism employed by the
created task_block
. In the following example the use of an explicit
par
execution policy makes the user’s intent explicit:
template <typename Func>
int traverse(node *n, Func&& compute)
{
int left = 0, right = 0;
define_task_block(
execution::par, // execution::parallel_policy
[&](task_block<>& tb) {
if (n->left)
tb.run([&] { left = traverse(n->left, compute); });
if (n->right)
tb.run([&] { right = traverse(n->right, compute); });
});
return compute(n) + left + right;
}
This also causes the hpx::parallel::v2::task_block
object to be a
template in our implementation. The template argument is the type of the
execution policy used to create the task block. The template argument defaults
to hpx::parallel::execution::parallel_policy
.
HPX still supports calling hpx::parallel::v2::define_task_block
without an explicit execution policy. In this case the task block will run using
the hpx::parallel::execution::parallel_policy
.
HPX also adds the ability to access the execution policy which was used to
create a given task_block
.
Often, we want to be able to not only define an execution policy to use by
default for all spawned tasks inside the task block, but in addition to
customize the execution context for one of the tasks executed by
task_block::run
. Adding an optionally passed executor instance to that
function enables this use case:
template <typename Func>
int traverse(node *n, Func&& compute)
{
int left = 0, right = 0;
define_task_block(
execution::par, // execution::parallel_policy
[&](auto& tb) {
if (n->left)
{
// use explicitly specified executor to run this task
tb.run(my_executor(), [&] { left = traverse(n->left, compute); });
}
if (n->right)
{
// use the executor associated with the par execution policy
tb.run([&] { right = traverse(n->right, compute); });
}
});
return compute(n) + left + right;
}
HPX still supports calling hpx::parallel::v2::task_block::run
without an explicit executor object. In this case the task will be run using the
executor associated with the execution policy which was used to call
hpx::parallel::v2::define_task_block
.
Writing distributed HPX applications¶
This section focuses on the features of HPX needed to write distributed applications, namely the Active Global Address Space (AGAS), remotely executable functions (i.e. actions), and distributed objects (i.e. components).
Global names¶
HPX implements an Active Global Address Space (AGAS) which is exposing a single uniform address space spanning all localities an application runs on. AGAS is a fundamental component of the ParalleX execution model. Conceptually, there is no rigid demarcation of local or global memory in AGAS; all available memory is a part of the same address space. AGAS enables named objects to be moved (migrated) across localities without having to change the object’s name, i.e., no references to migrated objects have to be ever updated. This feature has significance for dynamic load balancing and in applications where the workflow is highly dynamic, allowing work to be migrated from heavily loaded nodes to less loaded nodes. In addition, immutability of names ensures that AGAS does not have to keep extra indirections (“bread crumbs”) when objects move, hence minimizing complexity of code management for system developers as well as minimizing overheads in maintaining and managing aliases.
The AGAS implementation in HPX does not automatically expose every local address to the global address space. It is the responsibility of the programmer to explicitly define which of the objects have to be globally visible and which of the objects are purely local.
In HPX global addresses (global names) are represented using the
hpx::id_type
data type. This data type is conceptually very similar to
void*
pointers as it does not expose any type information of the object it
is referring to.
The only predefined global addresses are assigned to all localities. The following HPX API functions allow one to retrieve the global addresses of localities:
hpx::find_here
: retrieve the global address of the locality this function is called on.hpx::find_all_localities
: retrieve the global addresses of all localities available to this application (including the locality the function is being called on).hpx::find_remote_localities
: retrieve the global addresses of all remote localities available to this application (not including the locality the function is being called on)hpx::get_num_localities
: retrieve the number of localities available to this application.hpx::find_locality
: retrieve the global address of any locality supporting the given component type.hpx::get_colocation_id
: retrieve the global address of the locality currently hosting the object with the given global address.
Additionally, the global addresses of localities can be used to create new instances of components using the following HPX API function:
hpx::components::new_
: Create a new instance of the givenComponent
type on the specified locality.
Note
HPX does not expose any functionality to delete component instances. All
global addresses (as represented using hpx::id_type
) are automatically
garbage collected. When the last (global) reference to a particular component
instance goes out of scope the corresponding component instance is
automatically deleted.
Applying actions¶
Action type definition¶
Actions are special types we use to describe possibly remote operations. For
every global function and every member function which has to be invoked
distantly, a special type must be defined. For any global function the special
macro HPX_PLAIN_ACTION
can be used to define the
action type. Here is an example demonstrating this:
namespace app
{
void some_global_function(double d)
{
cout << d;
}
}
// This will define the action type 'some_global_action' which represents
// the function 'app::some_global_function'.
HPX_PLAIN_ACTION(app::some_global_function, some_global_action);
Important
The macro HPX_PLAIN_ACTION
has to be placed in
global namespace, even if the wrapped function is located in some other
namespace. The newly defined action type is placed in the global namespace as
well.
If the action type should be defined somewhere not in global namespace, the
action type definition has to be split into two macro invocations
(HPX_DEFINE_PLAIN_ACTION
and HPX_REGISTER_ACTION
) as shown
in the next example:
namespace app
{
void some_global_function(double d)
{
cout << d;
}
// On conforming compilers the following macro expands to:
//
// typedef hpx::actions::make_action<
// decltype(&some_global_function), &some_global_function
// >::type some_global_action;
//
// This will define the action type 'some_global_action' which represents
// the function 'some_global_function'.
HPX_DEFINE_PLAIN_ACTION(some_global_function, some_global_action);
}
// The following macro expands to a series of definitions of global objects
// which are needed for proper serialization and initialization support
// enabling the remote invocation of the function``some_global_function``
HPX_REGISTER_ACTION(app::some_global_action, app_some_global_action);
The shown code defines an action type some_global_action
inside the namespace
app
.
Important
If the action type definition is split between two macros as shown above, the
name of the action type to create has to be the same for both macro
invocations (here some_global_action
).
Important
The second argument passed to HPX_REGISTER_ACTION
(app_some_global_action
) has
to comprise a globally unique C++ identifier representing the action. This is
used for serialization purposes.
For member functions of objects which have been registered with AGAS
(e.g. ‘components’) a different registration macro
HPX_DEFINE_COMPONENT_ACTION
has to be utilized. Any component needs
to be declared in a header file and have some special support macros defined in
a source file. Here is an example demonstrating this. The first snippet has to
go into the header file:
namespace app
{
struct some_component
: hpx::components::component_base<some_component>
{
int some_member_function(std::string s)
{
return boost::lexical_cast<int>(s);
}
// This will define the action type 'some_member_action' which
// represents the member function 'some_member_function' of the
// object type 'some_component'.
HPX_DEFINE_COMPONENT_ACTION(some_component, some_member_function,
some_member_action);
};
}
// Note: The second argument to the macro below has to be systemwide-unique
// C++ identifiers
HPX_REGISTER_ACTION_DECLARATION(app::some_component::some_member_action, some_component_some_action);
The next snippet belongs into a source file (e.g. the main application source file) in the simplest case:
typedef hpx::components::component<app::some_component> component_type;
typedef app::some_component some_component;
HPX_REGISTER_COMPONENT(component_type, some_component);
// The parameters for this macro have to be the same as used in the corresponding
// HPX_REGISTER_ACTION_DECLARATION() macro invocation above
typedef some_component::some_member_action some_component_some_action;
HPX_REGISTER_ACTION(some_component_some_action);
Granted, these macro invocations are a bit more complex than for simple global functions, however we believe they are still manageable.
The most important macro invocation is the HPX_DEFINE_COMPONENT_ACTION
in the header file
as this defines the action type we need to invoke the member function. For a
complete example of a simple component action see [hpx_link
examples/quickstart/component_in_executable.cpp..component_in_executable.cpp]
Action invocation¶
The process of invoking a global function (or a member function of an object) with the help of the associated action is called ‘applying the action’. Actions can have arguments, which will be supplied while the action is applied. At the minimum, one parameter is required to apply any action - the id of the locality the associated function should be invoked on (for global functions), or the id of the component instance (for member functions). Generally, HPX provides several ways to apply an action, all of which are described in the following sections.
Generally, HPX actions are very similar to ‘normal’ C++ functions except that actions can be invoked remotely. Fig. 8 below shows an overview of the main API exposed by HPX. This shows the function invocation syntax as defined by the C++ language (dark gray), the additional invocation syntax as provided through C++ Standard Library features (medium gray), and the extensions added by HPX (light gray) where:
f
function to invoke,p..
: (optional) arguments,R
: return type off
,action
: action type defined by,HPX_DEFINE_PLAIN_ACTION
orHPX_DEFINE_COMPONENT_ACTION
encapsulatingf
,a
: an instance of the type`action
,id
: the global address the action is applied to.
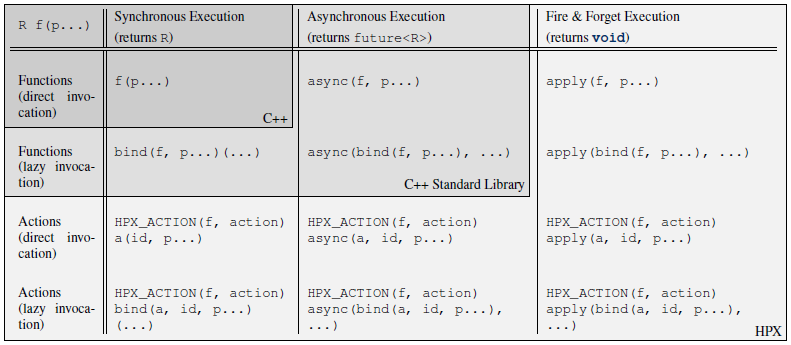
Fig. 8 Overview of the main API exposed by HPX.
This figure shows that HPX allows the user to apply actions with a syntax
similar to the C++ standard. In fact, all action types have an overloaded
function operator allowing to synchronously apply the action. Further, HPX
implements hpx::async
which semantically works similar to the
way std::async
works for plain C++ function.
Note
The similarity of applying an action to conventional function invocations
extends even further. HPX implements hpx::bind
and hpx::function
two facilities which are semantically equivalent to the std::bind
and
std::function
types as defined by the C++11 Standard. While
hpx::async
extends beyond the conventional semantics by supporting
actions and conventional C++ functions, the HPX facilities hpx::bind
and hpx::function
extend beyond the conventional standard facilities too.
The HPX facilities not only support conventional functions, but can be used
for actions as well.
Additionally, HPX exposes hpx::apply
and hpx::async_continue
both of
which refine and extend the standard C++ facilities.
The different ways to invoke a function in HPX will be explained in more detail in the following sections.
Applying an action asynchronously without any synchronization¶
This method (‘fire and forget’) will make sure the function associated with the action is scheduled to run on the target locality. Applying the action does not wait for the function to start running, instead it is a fully asynchronous operation. The following example shows how to apply the action as defined in the previous section on the local locality (the locality this code runs on):
some_global_action act; // define an instance of some_global_action
hpx::apply(act, hpx::find_here(), 2.0);
(the function hpx::find_here()
returns the id of the local locality,
i.e. the locality this code executes on).
Any component member function can be invoked using the same syntactic construct.
Given that id
is the global address for a component instance created
earlier, this invocation looks like:
some_component_action act; // define an instance of some_component_action
hpx::apply(act, id, "42");
In this case any value returned from this action (e.g. in this case the integer
42
is ignored. Please look at Action type definition for the code
defining the component action some_component_action
used.
Applying an action asynchronously with synchronization¶
This method will make sure the action is scheduled to run on the target
locality. Applying the action itself does not wait for the function to
start running or to complete, instead this is a fully asynchronous operation
similar to using hpx::apply
as described above. The difference is that this
method will return an instance of a hpx::future<>
encapsulating the result
of the (possibly remote) execution. The future can be used to synchronize with
the asynchronous operation. The following example shows how to apply the action
from above on the local locality:
some_global_action act; // define an instance of some_global_action
hpx::future<void> f = hpx::async(act, hpx::find_here(), 2.0);
//
// ... other code can be executed here
//
f.get(); // this will possibly wait for the asynchronous operation to 'return'
(as before, the function hpx::find_here()
returns the id of the local
locality (the locality this code is executed on).
Note
The use of a hpx::future<void>
allows the current thread to synchronize
with any remote operation not returning any value.
Note
Any std::future<>
returned from std::async()
is required to block in
its destructor if the value has not been set for this future yet. This is not
true for hpx::future<>
which will never block in its destructor, even if
the value has not been returned to the future yet. We believe that
consistency in the behavior of futures is more important than standards
conformance in this case.
Any component member function can be invoked using the same syntactic construct.
Given that id
is the global address for a component instance created
earlier, this invocation looks like:
some_component_action act; // define an instance of some_component_action
hpx::future<int> f = hpx::async(act, id, "42");
//
// ... other code can be executed here
//
cout << f.get(); // this will possibly wait for the asynchronous operation to 'return' 42
Note
The invocation of f.get()
will return the result immediately (without
suspending the calling thread) if the result from the asynchronous operation
has already been returned. Otherwise, the invocation of f.get()
will
suspend the execution of the calling thread until the asynchronous operation
returns its result.
Applying an action synchronously¶
This method will schedule the function wrapped in the specified action on the target locality. While the invocation appears to be synchronous (as we will see), the calling thread will be suspended while waiting for the function to return. Invoking a plain action (e.g. a global function) synchronously is straightforward:
some_global_action act; // define an instance of some_global_action
act(hpx::find_here(), 2.0);
While this call looks just like a normal synchronous function invocation, the function wrapped by the action will be scheduled to run on a new thread and the calling thread will be suspended. After the new thread has executed the wrapped global function, the waiting thread will resume and return from the synchronous call.
Equivalently, any action wrapping a component member function can be invoked synchronously as follows:
some_component_action act; // define an instance of some_component_action
int result = act(id, "42");
The action invocation will either schedule a new thread locally to execute the
wrapped member function (as before, id
is the global address of the
component instance the member function should be invoked on), or it will send a
parcel to the remote locality of the component causing a new thread to
be scheduled there. The calling thread will be suspended until the function
returns its result. This result will be returned from the synchronous action
invocation.
It is very important to understand that this ‘synchronous’ invocation syntax in fact conceals an asynchronous function call. This is beneficial as the calling thread is suspended while waiting for the outcome of a potentially remote operation. The HPX thread scheduler will schedule other work in the mean time, allowing the application to make further progress while the remote result is computed. This helps overlapping computation with communication and hiding communication latencies.
Note
The syntax of applying an action is always the same, regardless whether the target locality is remote to the invocation locality or not. This is a very important feature of HPX as it frees the user from the task of keeping track what actions have to be applied locally and which actions are remote. If the target for applying an action is local, a new thread is automatically created and scheduled. Once this thread is scheduled and run, it will execute the function encapsulated by that action. If the target is remote, HPX will send a parcel to the remote locality which encapsulates the action and its parameters. Once the parcel is received on the remote locality HPX will create and schedule a new thread there. Once this thread runs on the remote locality, it will execute the function encapsulated by the action.
Applying an action with a continuation but without any synchronization¶
This method is very similar to the method described in section Applying an action asynchronously without any synchronization. The
difference is that it allows the user to chain a sequence of asynchronous
operations, while handing the (intermediate) results from one step to the next
step in the chain. Where hpx::apply
invokes a single function using ‘fire
and forget’ semantics, hpx::apply_continue
asynchronously triggers a chain
of functions without the need for the execution flow ‘to come back’ to the
invocation site. Each of the asynchronous functions can be executed on a
different locality.
Applying an action with a continuation and with synchronization¶
This method is very similar to the method described in section Applying an action asynchronously with synchronization. In
addition to what hpx::async
can do, the functions hpx::async_continue
takes an additional function argument. This function will be called as the
continuation of the executed action. It is expected to perform additional
operations and to make sure that a result is returned to the original invocation
site. This method chains operations asynchronously by providing a continuation
operation which is automatically executed once the first action has finished
executing.
As an example we chain two actions, where the result of the first action is forwarded to the second action and the result of the second action is sent back to the original invocation site:
// first action
std::int32_t action1(std::int32_t i)
{
return i+1;
}
HPX_PLAIN_ACTION(action1); // defines action1_type
// second action
std::int32_t action2(std::int32_t i)
{
return i*2;
}
HPX_PLAIN_ACTION(action2); // defines action2_type
// this code invokes 'action1' above and passes along a continuation
// function which will forward the result returned from 'action1' to
// 'action2'.
action1_type act1; // define an instance of 'action1_type'
action2_type act2; // define an instance of 'action2_type'
hpx::future<int> f =
hpx::async_continue(act1, hpx::make_continuation(act2),
hpx::find_here(), 42);
hpx::cout << f.get() << "\n"; // will print: 86 ((42 + 1) * 2)
By default, the continuation is executed on the same locality as
hpx::async_continue
is invoked from. If you want to specify the
locality where the continuation should be executed, the code above has
to be written as:
// this code invokes 'action1' above and passes along a continuation
// function which will forward the result returned from 'action1' to
// 'action2'.
action1_type act1; // define an instance of 'action1_type'
action2_type act2; // define an instance of 'action2_type'
hpx::future<int> f =
hpx::async_continue(act1, hpx::make_continuation(act2, hpx::find_here()),
hpx::find_here(), 42);
hpx::cout << f.get() << "\n"; // will print: 86 ((42 + 1) * 2)
Similarly, it is possible to chain more than 2 operations:
action1_type act1; // define an instance of 'action1_type'
action2_type act2; // define an instance of 'action2_type'
hpx::future<int> f =
hpx::async_continue(act1,
hpx::make_continuation(act2, hpx::make_continuation(act1)),
hpx::find_here(), 42);
hpx::cout << f.get() << "\n"; // will print: 87 ((42 + 1) * 2 + 1)
The function hpx::make_continuation
creates a special function object
which exposes the following prototype:
struct continuation
{
template <typename Result>
void operator()(hpx::id_type id, Result&& result) const
{
...
}
};
where the parameters passed to the overloaded function operator operator()()
are:
- the
id
is the global id where the final result of the asynchronous chain of operations should be sent to (in most cases this is the id of thehpx::future
returned from the initial call tohpx::async_continue
. Any custom continuation function should make sure thisid
is forwarded to the last operation in the chain. - the
result
is the result value of the current operation in the asynchronous execution chain. This value needs to be forwarded to the next operation.
Note
All of those operations are implemented by the predefined continuation
function object which is returned from hpx::make_continuation
. Any (custom)
function object used as a continuation should conform to the same interface.
Action error handling¶
Like in any other asynchronous invocation scheme it is important to be able to handle error conditions occurring while the asynchronous (and possibly remote) operation is executed. In HPX all error handling is based on standard C++ exception handling. Any exception thrown during the execution of an asynchronous operation will be transferred back to the original invocation locality, where it is rethrown during synchronization with the calling thread.
Important
Exceptions thrown during asynchronous execution can be transferred back to
the invoking thread only for the synchronous and the asynchronous case with
synchronization. Like with any other unhandled exception, any exception
thrown during the execution of an asynchronous action without
synchronization will result in calling hpx::terminate
causing the running
application to exit immediately.
Note
Even if error handling internally relies on exceptions, most of the API functions exposed by HPX can be used without throwing an exception. Please see Working with exceptions for more information.
As an example, we will assume that the following remote function will be executed:
namespace app
{
void some_function_with_error(int arg)
{
if (arg < 0) {
HPX_THROW_EXCEPTION(bad_parameter, "some_function_with_error",
"some really bad error happened");
}
// do something else...
}
}
// This will define the action type 'some_error_action' which represents
// the function 'app::some_function_with_error'.
HPX_PLAIN_ACTION(app::some_function_with_error, some_error_action);
The use of HPX_THROW_EXCEPTION
to report the error encapsulates the
creation of a hpx::exception
which is initialized with the error
code hpx::bad_parameter
. Additionally it carries the passed strings, the
information about the file name, line number, and call stack of the point the
exception was thrown from.
We invoke this action using the synchronous syntax as described before:
// note: wrapped function will throw hpx::exception
some_error_action act; // define an instance of some_error_action
try {
act(hpx::find_here(), -3); // exception will be rethrown from here
}
catch (hpx::exception const& e) {
// prints: 'some really bad error happened: HPX(bad parameter)'
cout << e.what();
}
If this action is invoked asynchronously with synchronization, the exception is
propagated to the waiting thread as well and is re-thrown from the future’s
function get()
:
// note: wrapped function will throw hpx::exception
some_error_action act; // define an instance of some_error_action
hpx::future<void> f = hpx::async(act, hpx::find_here(), -3);
try {
f.get(); // exception will be rethrown from here
}
catch (hpx::exception const& e) {
// prints: 'some really bad error happened: HPX(bad parameter)'
cout << e.what();
}
For more information about error handling please refer to the section Working with exceptions. There we also explain how to handle error conditions without having to rely on exception.
Writing components¶
A component in HPX is a C++ class which can be created remotely and for which its member functions can be invoked remotely as well. The following sections highlight how components can be defined, created, and used.
Defining components¶
In order for a C++ class type to be managed remotely in HPX, the type must be
derived from the hpx::components::component_base
template type. We
call such C++ class types ‘components’.
Note that the component type itself is passed as a template argument to the base class:
// header file some_component.hpp
#include <hpx/include/components.hpp>
namespace app
{
// Define a new component type 'some_component'
struct some_component
: hpx::components::component_base<some_component>
{
// This member function is has to be invoked remotely
int some_member_function(std::string const& s)
{
return boost::lexical_cast<int>(s);
}
// This will define the action type 'some_member_action' which
// represents the member function 'some_member_function' of the
// object type 'some_component'.
HPX_DEFINE_COMPONENT_ACTION(some_component, some_member_function, some_member_action);
};
}
// This will generate the necessary boiler-plate code for the action allowing
// it to be invoked remotely. This declaration macro has to be placed in the
// header file defining the component itself.
//
// Note: The second argument to the macro below has to be systemwide-unique
// C++ identifiers
//
HPX_REGISTER_ACTION_DECLARATION(app::some_component::some_member_action, some_component_some_action);
There is more boiler plate code which has to be placed into a source file in order for the component to be usable. Every component type is required to have macros placed into its source file, one for each component type and one macro for each of the actions defined by the component type.
For instance:
// source file some_component.cpp
#include "some_component.hpp"
// The following code generates all necessary boiler plate to enable the
// remote creation of 'app::some_component' instances with 'hpx::new_<>()'
//
using some_component = app::some_component;
using some_component_type = hpx::components::component<some_component>;
// Please note that the second argument to this macro must be a
// (system-wide) unique C++-style identifier (without any namespaces)
//
HPX_REGISTER_COMPONENT(some_component_type, some_component);
// The parameters for this macro have to be the same as used in the corresponding
// HPX_REGISTER_ACTION_DECLARATION() macro invocation in the corresponding
// header file.
//
// Please note that the second argument to this macro must be a
// (system-wide) unique C++-style identifier (without any namespaces)
//
HPX_REGISTER_ACTION(app::some_component::some_member_action, some_component_some_action);
Defining client side representation classes¶
Often it is very convenient to define a separate type for a component which can be used on the client side (from where the component is instantiated and used). This step might seem as unnecessary duplicating code, however it significantly increases the type safety of the code.
A possible implementation of such a client side representation for the component described in the previous section could look like:
#include <hpx/include/components.hpp>
namespace app
{
// Define a client side representation type for the component type
// 'some_component' defined in the previous section.
//
struct some_component_client
: hpx::components::client_base<some_component_client, some_component>
{
using base_type = hpx::components::client_base<
some_component_client, some_component>;
some_component_client(hpx::future<hpx::id_type> && id)
: base_type(std::move(id))
{}
hpx::future<int> some_member_function(std::string const& s)
{
some_component::some_member_action act;
return hpx::async(act, get_id(), s);
}
};
}
A client side object stores the global id of the component instance it
represents. This global id is accessible by calling the function
client_base<>::get_id()
. The special constructor which is provided in the
example allows to create this client side object directly using the API function
hpx::new_
.
Creating component instances¶
Instances of defined component types can be created in two different ways. If the component to create has a defined client side representation type, then this can be used, otherwise use the server type.
The following examples assume that some_component_type
is the type of the
server side implementation of the component to create. All additional arguments
(see , ...
notation below) are passed through to the corresponding
constructor calls of those objects:
// create one instance on the given locality
hpx::id_type here = hpx::find_here();
hpx::future<hpx::id_type> f =
hpx::new_<some_component_type>(here, ...);
// create one instance using the given distribution
// policy (here: hpx::colocating_distribution_policy)
hpx::id_type here = hpx::find_here();
hpx::future<hpx::id_type> f =
hpx::new_<some_component_type>(hpx::colocated(here), ...);
// create multiple instances on the given locality
hpx::id_type here = find_here();
hpx::future<std::vector<hpx::id_type>> f =
hpx::new_<some_component_type[]>(here, num, ...);
// create multiple instances using the given distribution
// policy (here: hpx::binpacking_distribution_policy)
hpx::future<std::vector<hpx::id_type>> f = hpx::new_<some_component_type[]>(
hpx::binpacking(hpx::find_all_localities()), num, ...);
The examples below demonstrate the use of the same API functions for creating
client side representation objects (instead of just plain ids). These examples
assume that client_type
is the type of the client side representation of the
component type to create. As above, all additional arguments
(see , ...
notation below) are passed through to the corresponding constructor
calls of the server side implementation objects corresponding to the
client_type
:
// create one instance on the given locality
hpx::id_type here = hpx::find_here();
client_type c = hpx::new_<client_type>(here, ...);
// create one instance using the given distribution
// policy (here: hpx::colocating_distribution_policy)
hpx::id_type here = hpx::find_here();
client_type c = hpx::new_<client_type>(hpx::colocated(here), ...);
// create multiple instances on the given locality
hpx::id_type here = hpx::find_here();
hpx::future<std::vector<client_type>> f =
hpx::new_<client_type[]>(here, num, ...);
// create multiple instances using the given distribution
// policy (here: hpx::binpacking_distribution_policy)
hpx::future<std::vector<client_type>> f = hpx::new_<client_type[]>(
hpx::binpacking(hpx::find_all_localities()), num, ...);
Using component instances¶
Segmented containers¶
In parallel programming, there is now a plethora of solutions aimed at implementing “partially contiguous” or segmented data structures, whether on shared memory systems or distributed memory systems. HPX implements such structures by drawing inspiration from Standard C++ containers.
Using segmented containers¶
A segmented container is a template class that is described in the namespace
hpx
. All segmented containers are very similar semantically to their
sequential counterpart (defined in namespace std
but with an additional
template parameter named DistPolicy
). The distribution policy is an optional
parameter that is passed last to the segmented container constructor (after the
container size when no default value is given, after the default value if not).
The distribution policy describes the manner in which a container is segmented
and the placement of each segment among the available runtime localities.
However, only a part of the std
container member functions were
reimplemented:
(constructor)
,(destructor)
,operator=
operator[]
begin
,cbegin
,end
,cend
size
An example of how to use the partitioned_vector
container would be:
#include <hpx/include/partitioned_vector.hpp>
// The following code generates all necessary boiler plate to enable the
// remote creation of 'partitioned_vector' segments
//
HPX_REGISTER_PARTITIONED_VECTOR(double);
// By default, the number of segments is equal to the current number of
// localities
//
hpx::partitioned_vector<double> va(50);
hpx::partitioned_vector<double> vb(50, 0.0);
An example of how to use the partitioned_vector
container
with distribution policies would be:
#include <hpx/include/partitioned_vector.hpp>
#include <hpx/runtime/find_localities.hpp>
// The following code generates all necessary boiler plate to enable the
// remote creation of 'partitioned_vector' segments
//
HPX_REGISTER_PARTITIONED_VECTOR(double);
std::size_t num_segments = 10;
std::vector<hpx::id_type> locs = hpx::find_all_localities()
auto layout =
hpx::container_layout( num_segments, locs );
// The number of segments is 10 and those segments are spread across the
// localities collected in the variable locs in a Round-Robin manner
//
hpx::partitioned_vector<double> va(50, layout);
hpx::partitioned_vector<double> vb(50, 0.0, layout);
By definition, a segmented container must be accessible from any thread although its construction is synchronous only for the thread who has called its constructor. To overcome this problem, it is possible to assign a symbolic name to the segmented container:
#include <hpx/include/partitioned_vector.hpp>
// The following code generates all necessary boiler plate to enable the
// remote creation of 'partitioned_vector' segments
//
HPX_REGISTER_PARTITIONED_VECTOR(double);
hpx::future<void> fserver = hpx::async(
[](){
hpx::partitioned_vector<double> v(50);
// Register the 'partitioned_vector' with the name "some_name"
//
v.register_as("some_name");
/* Do some code */
});
hpx::future<void> fclient =
hpx::async(
[](){
// Naked 'partitioned_vector'
//
hpx::partitioned_vector<double> v;
// Now the variable v points to the same 'partitioned_vector' that has
// been registered with the name "some_name"
//
v.connect_to("some_name");
/* Do some code */
});
HPX provides the following segmented containers:
Name | Description | In header | Class page at cppreference.com |
hpx::partitioned_vector |
Dynamic segmented contiguous array. | <hpx/include/partitioned_vector.hpp> |
vector |
Name | Description | In header | Class page at cppreference.com |
hpx::unordered_map |
Segmented collection of key-value pairs, hashed by keys, keys are unique. | <hpx/include/unordered_map.hpp> |
unordered_map |
Segmented iterators and segmented iterator traits¶
The basic iterator used in the STL library is only suitable for one-dimensional
structures. The iterators we use in HPX must adapt to the segmented format of
our containers. Our iterators are then able to know when incrementing themselves
if the next element of type T
is in the same data segment or in another
segment. In this second case, the iterator will automatically point to the
beginning of the next segment.
Note
Note that the dereference operation operator *
does not directly return a
reference of type T&
but an intermediate object wrapping this reference.
When this object is used as an l-value, a remote write operation is
performed; When this object is used as an r-value, implicit conversion to
T
type will take care of performing remote read operation.
It is sometimes useful not only to iterate element by element, but also segment
by segment, or simply get a local iterator in order to avoid additional
construction costs at each deferencing operations. To mitigate this need, the
hpx::traits::segmented_iterator_traits
are used.
With segmented_iterator_traits
users can uniformly get the iterators
which specifically iterates over segments (by providing a segmented iterator
as a parameter), or get the local begin/end iterators of the nearest
local segment (by providing a per-segment iterator as a parameter):
#include <hpx/include/partitioned_vector.hpp>
// The following code generates all necessary boiler plate to enable the
// remote creation of 'partitioned_vector' segments
//
HPX_REGISTER_PARTITIONED_VECTOR(double);
using iterator = hpx::partitioned_vector<T>::iterator;
using traits = hpx::traits::segmented_iterator_traits<iterator>;
hpx::partitioned_vector<T> v;
std::size_t count = 0;
auto seg_begin = traits::segment(v.begin());
auto seg_end = traits::segment(v.end());
// Iterate over segments
for (auto seg_it = seg_begin; seg_it != seg_end; ++seg_it)
{
auto loc_begin = traits::begin(seg_it)
auto loc_end = traits::end(seg_it);
// Iterate over elements inside segments
for (auto lit = loc_begin; lit != loc_end; ++lit, ++count)
{
*lit = count;
}
}
Which is equivalent to:
hpx::partitioned_vector<T> v;
std::size_t count = 0;
auto begin = v.begin();
auto end = v.end();
for (auto it = begin; it != end; ++it, ++count)
{
*it = count;
}
Using views¶
The use of multidimensional arrays is quite common in the numerical field
whether to perform dense matrix operations or to process images. It exist many
libraries which implement such object classes overloading their basic operators
(e.g.``+``, -
, *
, ()
, etc.). However, such operation becomes more
delicate when the underlying data layout is segmented or when it is mandatory to
use optimized linear algebra subroutines (i.e. BLAS subroutines).
Our solution is thus to relax the level of abstraction by allowing the user to work not directly on n-dimensionnal data, but on “n-dimensionnal collections of 1-D arrays”. The use of well-accepted techniques on contiguous data is thus preserved at the segment level, and the composability of the segments is made possible thanks to multidimensional array-inspired access mode.
Although HPX refutes by design this programming model, the locality plays a dominant role when it comes to implement vectorized code. To maximize local computations and avoid unneeded data transfers, a parallel section (or Single Programming Multiple Data section) is required. Because the use of global variables is prohibited, this parallel section is created via the RAII idiom.
To define a parallel section, simply write an action taking a spmd_block
variable as a first parameter:
#include <hpx/lcos/spmd_block.hpp>
void bulk_function(hpx::lcos::spmd_block block /* , arg0, arg1, ... */)
{
// Parallel section
/* Do some code */
}
HPX_PLAIN_ACTION(bulk_function, bulk_action);
Note
In the following paragraphs, we will use the term “image” several times. An image is defined as a lightweight process whose entry point is a function provided by the user. It’s an “image of the function”.
The spmd_block
class contains the following methods:
- [def Team information]
get_num_images
,this_image
,images_per_locality
- [def Control statements]
sync_all
,sync_images
Here is a sample code summarizing the features offered by the spmd_block
class:
#include <hpx/lcos/spmd_block.hpp>
void bulk_function(hpx::lcos::spmd_block block /* , arg0, arg1, ... */)
{
std::size_t num_images = block.get_num_images();
std::size_t this_image = block.this_image();
std::size_t images_per_locality = block.images_per_locality();
/* Do some code */
// Synchronize all images in the team
block.sync_all();
/* Do some code */
// Synchronize image 0 and image 1
block.sync_images(0,1);
/* Do some code */
std::vector<std::size_t> vec_images = {2,3,4};
// Synchronize images 2, 3 and 4
block.sync_images(vec_images);
// Alternative call to synchronize images 2, 3 and 4
block.sync_images(vec_images.begin(), vec_images.end());
/* Do some code */
// Non-blocking version of sync_all()
hpx::future<void> event =
block.sync_all(hpx::launch::async);
// Callback waiting for 'event' to be ready before being scheduled
hpx::future<void> cb =
event.then(
[](hpx::future<void>)
{
/* Do some code */
});
// Finally wait for the execution tree to be finished
cb.get();
}
HPX_PLAIN_ACTION(bulk_test_function, bulk_test_action);
Then, in order to invoke the parallel section, call the function
define_spmd_block
specifying an arbitrary symbolic name and indicating the
number of images per locality to create:
void bulk_function(hpx::lcos::spmd_block block, /* , arg0, arg1, ... */)
{
}
HPX_PLAIN_ACTION(bulk_test_function, bulk_test_action);
int main()
{
/* std::size_t arg0, arg1, ...; */
bulk_action act;
std::size_t images_per_locality = 4;
// Instanciate the parallel section
hpx::lcos::define_spmd_block(
"some_name", images_per_locality, std::move(act) /*, arg0, arg1, ... */);
return 0;
}
Note
In principle, the user should never call the spmd_block
constructor. The
define_spmd_block
function is responsible of instantiating spmd_block
objects and broadcasting them to each created image.
Some classes are defined as “container views” when the purpose is to observe
and/or modify the values of a container using another perspective than the one
that characterizes the container. For example, the values of an std::vector
object can be accessed via the expression [i]
. Container views can be used,
for example, when it is desired for those values to be “viewed” as a 2D matrix
that would have been flattened in a std::vector
. The values would be
possibly accessible via the expression vv(i,j)
which would call internally
the expression v[k]
.
By default, the partitioned_vector
class integrates 1-D views of its segments:
#include <hpx/include/partitioned_vector.hpp>
// The following code generates all necessary boiler plate to enable the
// remote creation of 'partitioned_vector' segments
//
HPX_REGISTER_PARTITIONED_VECTOR(double);
using iterator = hpx::partitioned_vector<double>::iterator;
using traits = hpx::traits::segmented_iterator_traits<iterator>;
hpx::partitioned_vector<double> v;
// Create a 1-D view of the vector of segments
auto vv = traits::segment(v.begin());
// Access segment i
std::vector<double> v = vv[i];
Our views are called “multidimensional” in the sense that they generalize to N
dimensions the purpose of segmented_iterator_traits::segment()
in the 1-D
case. Note that in a parallel section, the 2-D expression a(i,j) = b(i,j)
is
quite confusing because without convention, each of the images invoked will race
to execute the statement. For this reason, our views are not only
multidimensional but also “spmd-aware”.
Note
SPMD-awareness: The convention is simple. If an assignment statement contains a view subscript as an l-value, it is only and only the image holding the r-value who is evaluating the statement. (In MPI sense, it is called a Put operation).
Here are some examples of using subscripts in the 2-D view case:
#include <hpx/components/containers/partitioned_vector/partitioned_vector_view.hpp>
#include <hpx/include/partitioned_vector.hpp>
// The following code generates all necessary boiler plate to enable the
// remote creation of 'partitioned_vector' segments
//
HPX_REGISTER_PARTITIONED_VECTOR(double);
using Vec = hpx::partitioned_vector<double>;
using View_2D = hpx::partitioned_vector_view<double,2>;
/* Do some code */
Vec v;
// Parallel section (suppose 'block' an spmd_block instance)
{
std::size_t height, width;
// Instanciate the view
View_2D vv(block, v.begin(), v.end(), {height,width});
// The l-value is a view subscript, the image that owns vv(1,0)
// evaluates the assignment.
vv(0,1) = vv(1,0);
// The l-value is a view subscript, the image that owns the r-value
// (result of expression 'std::vector<double>(4,1.0)') evaluates the
// assignment : oops! race between all participating images.
vv(2,3) = std::vector<double>(4,1.0);
}
Here are some examples of using iterators in the 3-D view case:
#include <hpx/components/containers/partitioned_vector/partitioned_vector_view.hpp>
#include <hpx/include/partitioned_vector.hpp>
// The following code generates all necessary boiler plate to enable the
// remote creation of 'partitioned_vector' segments
//
HPX_REGISTER_PARTITIONED_VECTOR(int);
using Vec = hpx::partitioned_vector<int>;
using View_3D = hpx::partitioned_vector_view<int,3>;
/* Do some code */
Vec v1, v2;
// Parallel section (suppose 'block' an spmd_block instance)
{
std::size_t sixe_x, size_y, size_z;
// Instanciate the views
View_3D vv1(block, v1.begin(), v1.end(), {sixe_x,size_y,size_z});
View_3D vv2(block, v2.begin(), v2.end(), {sixe_x,size_y,size_z});
// Save previous segments covered by vv1 into segments covered by vv2
auto vv2_it = vv2.begin();
auto vv1_it = vv1.cbegin();
for(; vv2_it != vv2.end(); vv2_it++, vv1_it++)
{
// It's a Put operation
*vv2_it = *vv1_it;
}
// Ensure that all images have performed their Put operations
block.sync_all();
// Ensure that only one image is putting updated data into the different
// segments covered by vv1
if(block.this_image() == 0)
{
int idx = 0;
// Update all the segments covered by vv1
for(auto i = vv1.begin(); i != vv1.end(); i++)
{
// It's a Put operation
*i = std::vector<float>(elt_size,idx++);
}
}
}
Here is an example that shows how to iterate only over segments owned by the current image:
#include <hpx/components/containers/partitioned_vector/partitioned_vector_view.hpp>
#include <hpx/components/containers/partitioned_vector/partitioned_vector_local_view.hpp>
#include <hpx/include/partitioned_vector.hpp>
// The following code generates all necessary boiler plate to enable the
// remote creation of 'partitioned_vector' segments
//
HPX_REGISTER_PARTITIONED_VECTOR(float);
using Vec = hpx::partitioned_vector<float>;
using View_1D = hpx::partitioned_vector_view<float,1>;
/* Do some code */
Vec v;
// Parallel section (suppose 'block' an spmd_block instance)
{
std::size_t num_segments;
// Instanciate the view
View_1D vv(block, v.begin(), v.end(), {num_segments});
// Instanciate the local view from the view
auto local_vv = hpx::local_view(vv);
for ( auto i = localvv.begin(); i != localvv.end(); i++ )
{
std::vector<float> & segment = *i;
/* Do some code */
}
}
It is possible to construct views from other views: we call it sub-views. The constraint nevertheless for the subviews is to retain the dimension and the value type of the input view. Here is an example showing how to create a sub-view:
#include <hpx/components/containers/partitioned_vector/partitioned_vector_view.hpp>
#include <hpx/include/partitioned_vector.hpp>
// The following code generates all necessary boiler plate to enable the
// remote creation of 'partitioned_vector' segments
//
HPX_REGISTER_PARTITIONED_VECTOR(float);
using Vec = hpx::partitioned_vector<float>;
using View_2D = hpx::partitioned_vector_view<float,2>;
/* Do some code */
Vec v;
// Parallel section (suppose 'block' an spmd_block instance)
{
std::size_t N = 20;
std::size_t tilesize = 5;
// Instanciate the view
View_2D vv(block, v.begin(), v.end(), {N,N});
// Instanciate the subview
View_2D svv(
block,&vv(tilesize,0),&vv(2*tilesize-1,tilesize-1),{tilesize,tilesize},{N,N});
if(block.this_image() == 0)
{
// Equivalent to 'vv(tilesize,0) = 2.0f'
svv(0,0) = 2.0f;
// Equivalent to 'vv(2*tilesize-1,tilesize-1) = 3.0f'
svv(tilesize-1,tilesize-1) = 3.0f;
}
}
Note
The last parameter of the subview constructor is the size of the original
view. If one would like to create a subview of the subview and so on, this
parameter should stay unchanged. {N,N}
for the above example).
C++ co-arrays¶
Fortran has extended its scalar element indexing approach to reference each segment of a distributed array. In this extension, a segment is attributed a ?co-index? and lives in a specific locality. A co-index provides the application with enough information to retrieve the corresponding data reference. In C++, containers present themselves as a ?smarter? alternative of Fortran arrays but there are still no corresponding standardized features similar to the Fortran co-indexing approach. We present here an implementation of such features in HPX.
As mentioned before, a co-array is a distributed array whose segments are accessible through an array-inspired access mode. We have previously seen that it is possible to reproduce such access mode using the concept of views. Nevertheless, the user must pre-create a segmented container to instanciate this view. We illustrate below how a single constructor call can perform those two operations:
#include <hpx/components/containers/coarray/coarray.hpp>
#include <hpx/lcos/spmd_block.hpp>
// The following code generates all necessary boiler plate to enable the
// co-creation of 'coarray'
//
HPX_REGISTER_COARRAY(double);
// Parallel section (suppose 'block' an spmd_block instance)
{
using hpx::container::placeholders::_;
std::size_t height=32, width=4, segment_size=10;
hpx::coarray<double,3> a(block, "a", {height,width,_}, segment_size);
/* Do some code */
}
Unlike segmented containers, a co-array object can only be instantiated within a parallel section. Here is the description of the parameters to provide to the coarray constructor:
Parameter | Description |
block |
Reference to a spmd_block object |
"a" |
Symbolic name of type std::string |
{height,width,_} |
Dimensions of the coarray object |
segment_size |
Size of a co-indexed element (i.e. size of the object referenced by the
expression a(i,j,k) ) |
Note that the “last dimension size” cannot be set by the user. It only accepts
the constexpr variable hpx::container::placeholders::_
. This size, which is
considered private, is equal to the number of current images (value returned by
block.get_num_images()
).
Note
An important constraint to remember about coarray objects is that all segments sharing the same “last dimension index” are located in the same image.
The member functions owned by the coarray
objects are exactly the same as
those of spmd multidimensional views. These are:
* Subscript-based operations
* Iterator-based operations
However, one additional functionality is provided. Knowing that the element
a(i,j,k)
is in the memory of the k
th image, the use of local subscripts
is possible.
Note
For spmd multidimensional views, subscripts are only global as it still involves potential remote data transfers.
Here is an example of using local subscripts:
#include <hpx/components/containers/coarray/coarray.hpp>
#include <hpx/lcos/spmd_block.hpp>
// The following code generates all necessary boiler plate to enable the
// co-creation of 'coarray'
//
HPX_REGISTER_COARRAY(double);
// Parallel section (suppose 'block' an spmd_block instance)
{
using hpx::container::placeholders::_;
std::size_t height=32, width=4, segment_size=10;
hpx::coarray<double,3> a(block, "a", {height,width,_}, segment_size);
double idx = block.this_image()*height*width;
for (std::size_t j = 0; j<width; j++)
for (std::size_t i = 0; i<height; i++)
{
// Local write operation performed via the use of local subscript
a(i,j,_) = std::vector<double>(elt_size,idx);
idx++;
}
block.sync_all();
}
Note
When the “last dimension index” of a subscript is equal to
hpx::container::placeholders::_
, local subscript (and not global
subscript) is used. It is equivalent to a global subscript used with a “last
dimension index” equal to the value returned by block.this_image()
.
Running on batch systems¶
This section walks you through launching HPX applications on various batch systems.
How to use HPX applications with PBS¶
Most HPX applications are executed on parallel computers. These platforms typically provide integrated job management services that facilitate the allocation of computing resources for each parallel program. HPX includes out of the box support for one of the most common job management systems, the Portable Batch System (PBS).
All PBS jobs require a script to specify the resource requirements and other
parameters associated with a parallel job. The PBS script is basically a shell
script with PBS directives placed within commented sections at the beginning of
the file. The remaining (not commented-out) portions of the file executes just
like any other regular shell script. While the description of all available PBS
options is outside the scope of this tutorial (the interested reader may refer
to in-depth documentation for
more information), below is a minimal example to illustrate the approach. As a
test application we will use the multithreaded hello_world_distributed
program, explained in the section Remote execution with actions: Hello world.
#!/bin/bash
#
#PBS -l nodes=2:ppn=4
APP_PATH=~/packages/hpx/bin/hello_world_distributed
APP_OPTIONS=
pbsdsh -u $APP_PATH $APP_OPTIONS --hpx:nodes=`cat $PBS_NODEFILE`
Caution
If the first application specific argument (inside $APP_OPTIONS
) is a
non-option (i.e. does not start with a -
or a --
), then those have to
be placed before the option --hpx:nodes
, which in this case should
be the last option on the command line.
Alternatively, use the option --hpx:endnodes
to explicitly mark the
end of the list of node names:
pbsdsh -u $APP_PATH --hpx:nodes`cat $PBS_NODEFILE` --hpx:endnodes $APP_OPTIONS
The #PBS -l nodes=2:ppn=4
directive will cause two compute nodes to be
allocated for the application, as specified in the option nodes
. Each of the
nodes will dedicate four cores to the program, as per the option ppn
, short
for “processors per node” (PBS does not distinguish between processors and
cores). Note that requesting more cores per node than physically available is
pointless and may prevent PBS from accepting the script.
On newer PBS versions the PBS command syntax might be different. For instance, the PBS script above would look like:
#!/bin/bash
#
#PBS -l select=2:ncpus=4
APP_PATH=~/packages/hpx/bin/hello_world_distributed
APP_OPTIONS=
pbsdsh -u $APP_PATH $APP_OPTIONS --hpx:nodes=`cat $PBS_NODEFILE`
APP_PATH
and APP_OPTIONS
are shell variables that respectively specify
the correct path to the executable (hello_world_distributed
in this case)
and the command line options. Since the hello_world_distributed
application
doesn’t need any command line options, APP_OPTIONS
has been left empty.
Unlike in other execution environments, there is no need to use the
--hpx:threads
option to indicate the required number of OS threads per
node; the HPX library will derive this parameter automatically from PBS.
Finally, pbsdsh is a PBS command that starts tasks to the resources allocated to the current job. It is recommended to leave this line as shown and modify only the PBS options and shell variables as needed for a specific application.
Important
A script invoked by pbsdsh starts in a very basic environment: the user’s
$HOME
directory is defined and is the current directory, the LANG
variable is set to C
and the PATH
is set to the basic
/usr/local/bin:/usr/bin:/bin
as defined in a system-wide file
pbs_environment. Nothing that would normally be set up by a system shell
profile or user shell profile is defined, unlike the environment for the main
job script.
Another choice is for the pbsdsh command in your main job script to invoke
your program via a shell, like sh
or bash
so that it gives an initialized
environment for each instance. We create a small script runme.sh
which is used
to invoke the program:
#!/bin/bash
# Small script which invokes the program based on what was passed on its
# command line.
#
# This script is executed by the bash shell which will initialize all
# environment variables as usual.
$@
Now, we invoke this script using the pbsdsh tool:
#!/bin/bash
#
#PBS -l nodes=2:ppn=4
APP_PATH=~/packages/hpx/bin/hello_world_distributed
APP_OPTIONS=
pbsdsh -u runme.sh $APP_PATH $APP_OPTIONS --hpx:nodes=`cat $PBS_NODEFILE`
All that remains now is submitting the job to the queuing system. Assuming that
the contents of the PBS script were saved in file pbs_hello_world.sh
in the
current directory, this is accomplished by typing:
qsub ./pbs_hello_world_pbs.sh
If the job is accepted, qsub will print out the assigned job ID, which may look like:
$ 42.supercomputer.some.university.edu
To check the status of your job, issue the following command:
qstat 42.supercomputer.some.university.edu
and look for a single-letter job status symbol. The common cases include:
- Q - signifies that the job is queued and awaiting its turn to be executed.
- R - indicates that the job is currently running.
- C - means that the job has completed.
The example qstat output below shows a job waiting for execution resources to become available:
Job id Name User Time Use S Queue
------------------------- ---------------- --------------- -------- - -----
42.supercomputer ...ello_world.sh joe_user 0 Q batch
After the job completes, PBS will place two files, pbs_hello_world.sh.o42
and
pbs_hello_world.sh.e42
, in the directory where the job was submitted. The
first contains the standard output and the second contains the standard error
from all the nodes on which the application executed. In our example, the error
output file should be empty and standard output file should contain something
similar to:
hello world from OS-thread 3 on locality 0
hello world from OS-thread 2 on locality 0
hello world from OS-thread 1 on locality 1
hello world from OS-thread 0 on locality 0
hello world from OS-thread 3 on locality 1
hello world from OS-thread 2 on locality 1
hello world from OS-thread 1 on locality 0
hello world from OS-thread 0 on locality 1
Congratulations! You have just run your first distributed HPX application!
How to use HPX applications with SLURM¶
Just like PBS (described in section How to use HPX applications with PBS), SLURM is a job management system which is widely used on large supercomputing systems. Any HPX application can easily be run using SLURM. This section describes how this can be done.
The easiest way to run an HPX application using SLURM is to utilize the command line tool srun which interacts with the SLURM batch scheduling system:
srun -p <partition> -N <number-of-nodes> hpx-application <application-arguments>
Here, <partition>
is one of the node partitions existing on the target
machine (consult the machines documentation to get a list of existing
partitions) and <number-of-nodes>
is the number of compute nodes you want to
use. By default, the HPX application is started with one locality per
node and uses all available cores on a node. You can change the number of
localities started per node (for example to account for NUMA effects) by
specifying the -n
option of srun. The number of cores per locality
can be set by -c
. The <application-arguments>
are any application
specific arguments which need to be passed on to the application.
Note
There is no need to use any of the HPX command line options related to the number of localities, number of threads, or related to networking ports. All of this information is automatically extracted from the SLURM environment by the HPX startup code.
Important
The srun documentation explicitly states: “If -c
is specified without
-n
as many tasks will be allocated per node as possible while satisfying
the -c
restriction. For instance on a cluster with 8 CPUs per node, a job
request for 4 nodes and 3 CPUs per task may be allocated 3 or 6 CPUs per node
(1 or 2 tasks per node) depending upon resource consumption by other jobs.”
For this reason, we suggest to always specify -n <number-of-instances>
,
even if <number-of-instances>
is equal to one (1
).
Interactive shells¶
To get an interactive development shell on one of the nodes you can issue the following command:
srun -p <node-type> -N <number-of-nodes> --pty /bin/bash -l
After the shell has been opened, you can run your HPX application. By default,
it uses all available cores. Note that if you requested one node, you don’t need
to do srun
again. However, if you requested more than one node, and want to
run your distributed application, you can use srun
again to start up the
distributed HPX application. It will use the resources that have been requested
for the interactive shell.
Scheduling batch jobs¶
The above mentioned method of running HPX applications is fine for development
purposes. The disadvantage that comes with srun
is that it only returns once
the application is finished. This might not be appropriate for longer running
applications (for example benchmarks or larger scale simulations). In order to
cope with that limitation you can use the sbatch command.
The sbatch
command expects a script that it can run once the requested
resources are available. In order to request resources you need to add
#SBATCH
comments in your script or provide the necessary parameters to
sbatc
directly. The parameters are the same as with run
. The commands
you need to execute are the same you would need to start your application as if
you were in an interactive shell.
Debugging HPX applications¶
Using a debugger with HPX applications¶
Using a debugger such as gdb
with HPX applications is no problem. However,
there are some things to keep in mind to make the experience somewhat more
productive.
Call stacks in HPX can often be quite unwieldy as the library is heavily
templated and the call stacks can be very deep. For this reason it is sometimes
a good idea compile HPX in RelWithDebInfo
mode which applies some
optimizations but keeps debugging symbols. This can often compress call stacks
significantly. On the other hand, stepping through the code can also be more
difficult because of statements being reordered and variables being optimized
away. Also note that because HPX implements user-space threads and context
switching, call stacks may not always be complete in a debugger.
HPX launches not only worker threads but also a few helper threads. The first thread is the main thread which typically does no work in an HPX application, except at startup and shutdown. If using the default settings, HPX will spawn six additional threads (used for service thread pools). The first worker thread is usually the eighth thread, and most user code will be run on these worker threads. The last thread is a helper thread used for HPX shutdown.
Finally, since HPX is a multi-threaded runtime, the following gdb
options
can be helpful:
set pagination off
set non-stop on
Non-stop mode allows you to have a single thread stop on a breakpoint without stopping all other threads as well.
Using sanitizers with HPX applications¶
Warning
Not all parts of HPX are sanitizer-clean. This means that you may end up with false positives from HPX itself when using sanitizers for your application.
To use sanitizers with HPX you should turn on HPX_WITH_SANITIZERS
and turn
off HPX_WITH_STACK_OVERFLOW_DETECTION
during CMake configuration. It’s
recommended to also build Boost with the same sanitizers that you will be
using for HPX. The appropriate sanitizers can then be enabled using CMake by
appending -fsanitize=address -fno-omit-frame-pointer
to CMAKE_CXX_FLAGS
and -fsanitize=address
to CMAKE_EXE_LINKER_FLAGS
. Replace address
with the sanitizer that you want to use.
Optimizing HPX applications¶
Performance counters¶
Performance Counters in HPX are used to provide information as to how well the runtime system or an application is performing. The counter data can help determine system bottlenecks and fine-tune system and application performance. The HPX runtime system, its networking, and other layers provide counter data that an application can consume to provide users with information of how well the application is performing.
Applications can also use counter data to determine how much system resources to consume. For example, an application that transfers data over the network could consume counter data from a network switch to determine how much data to transfer without competing for network bandwidth with other network traffic. The application could use the counter data to adjust its transfer rate as the bandwidth usage from other network traffic increases or decreases.
Performance Counters are HPX parallel processes which expose a predefined interface. HPX exposes special API functions that allow one to create, manage, read the counter data, and release instances of Performance Counters. Performance Counter instances are accessed by name, and these names have a predefined structure which is described in the section Performance counter names. The advantage of this is that any Performance Counter can be accessed remotely (from a different locality) or locally (from the same locality). Moreover, since all counters expose their data using the same API, any code consuming counter data can be utilized to access arbitrary system information with minimal effort.
Counter data may be accessed in real time. More information about how to consume counter data can be found in the section Consuming performance counter data.
All HPX applications provide command line options related to performance counters, such as the ability to list available counter types, or periodically query specific counters to be printed to the screen or save them in a file. For more information, please refer to the section HPX Command Line Options.
Performance counter names¶
All Performance Counter instances have a name uniquely identifying this instance. This name can be used to access the counter, retrieve all related meta data, and to query the counter data (as described in the section Consuming performance counter data). Counter names are strings with a predefined structure. The general form of a countername is:
/objectname{full_instancename}/countername@parameters
where full_instancename
could be either another (full) counter name or a
string formatted as:
parentinstancename#parentindex/instancename#instanceindex
Each separate part of a countername (e.g. objectname
, countername
parentinstancename
, instancename
, and parameters
) should start with
a letter ('a'
…'z'
, 'A'
…'Z'
) or an underscore
character ('_'
), optionally followed by letters, digits ('0'
…'9'
), hyphen ('-'
), or underscore characters. Whitespace is not allowed
inside a counter name. The characters '/'
, '{'
, '}'
, '#'
and
'@'
have a special meaning and are used to delimit the different parts of
the counter name.
The parts parentinstanceindex
and instanceindex
are integers. If an
index is not specified HPX will assume a default of -1
.
Two simple examples¶
An instance for a well formed (and meaningful) simple counter name would be:
/threads{locality#0/total}/count/cumulative
This counter returns the current cumulative number of executed (retired)
HPX-threads for the locality 0
. The counter type of this counter
is /threads/count/cumulative
and the full instance name is
locality#0/total
. This counter type does not require an instanceindex
or
parameters
to be specified.
In this case, the parentindex
(the '0'
) designates the locality
for which the counter instance is created. The counter will return the number of
HPX-threads retired on that particular locality.
Another example for a well formed (aggregate) counter name is:
/statistics{/threads{locality#0/total}/count/cumulative}/average@500
This counter takes the simple counter from the first example, samples its values
every 500
milliseconds, and returns the average of the value samples
whenever it is queried. The counter type of this counter is
/statistics/average
and the instance name is the full name of the counter
for which the values have to be averaged. In this case, the parameters
(the
'500'
) specify the sampling interval for the averaging to take place (in
milliseconds).
Performance counter types¶
Every Performance Counter belongs to a specific Performance Counter type which
classifies the counters into groups of common semantics. The type of a counter
is identified by the objectname
and the countername
parts of the name.
/objectname/countername
At application start, HPX will register all available counter types on each of the localities. These counter types are held in a special Performance Counter registration database which can be later used to retrieve the meta data related to a counter type and to create counter instances based on a given counter instance name.
Performance counter instances¶
The full_instancename
distinguishes different counter instances of the same
counter type. The formatting of the full_instancename
depends on the counter
type. There are two types of counters: simple counters which usually generate
the counter values based on direct measurements, and aggregate counters which
take another counter and transform its values before generating their own
counter values. An example for a simple counter is given above:
counting retired HPX-threads. An aggregate counter is shown as an example
above as well: calculating the average of the underlying
counter values sampled at constant time intervals.
While simple counters use instance names formatted as
parentinstancename#parentindex/instancename#instanceindex
, most aggregate
counters have the full counter name of the embedded counter as its instance
name.
Not all simple counter types require specifying all 4 elements of a full counter
instance name, some of the parts parentinstancename
, parentindex
,
instancename
, and instanceindex
) are optional for specific counters.
Please refer to the documentation of a particular counter for more information
about the formatting requirements for the name of this counter (see
Existing HPX performance counters).
The parameters
are used to pass additional information to a counter at
creation time. They are optional and they fully depend on the concrete counter.
Even if a specific counter type allows additional parameters to be given, those
usually are not required as sensible defaults will be chosen. Please refer to
the documentation of a particular counter for more information about what
parameters are supported, how to specify them, and what default values are
assumed (see also Existing HPX performance counters).
Every locality of an application exposes its own set of Performance Counter types and Performance Counter instances. The set of exposed counters is determined dynamically at application start based on the execution environment of the application. For instance, this set is influenced by the current hardware environment for the locality (such as whether the locality has access to accelerators), and the software environment of the application (such as the number of OS-threads used to execute HPX-threads).
Using wildcards in performance counter names¶
It is possible to use wildcard characters when specifying performance counter names. Performance counter names can contain 2 types of wildcard characters:
- Wildcard characters in the performance counter type
- Wildcard characters in the performance counter instance name
Wildcard character have a meaning which is very close to usual file name wildcard matching rules implemented by common shells (like bash).
Wildcard | Description |
* |
This wildcard character matches any number (zero or more) of arbitrary characters. |
? |
This wildcard character matches any single arbitrary character. |
[...] |
This wildcard character matches any single character from the list of specified within the square brackets. |
Wildcard | Description |
* |
This wildcard character matches any locality or any thread,
depending on whether it is used for locality#* or
worker-thread#* . No other wildcards are allowed in counter instance
names. |
Consuming performance counter data¶
You can consume performance data using either the command line interface or via the HPX application or the HPX API. The command line interface is easier to use, but it is less flexible and does not allow one to adjust the behaviour of your application at runtime. The command line interface provides a convenience abstraction but simplified abstraction for querying and logging performance counter data for a set of performance counters.
Consuming performance counter data from the command line¶
HPX provides a set of predefined command line options for every application
which uses hpx::init
for its initialization. While there are much more
command line options available (see HPX Command Line Options), the set of options related
to Performance Counters allow one to list existing counters, query existing
counters once at application termination or repeatedly after a constant time
interval.
The following table summarizes the available command line options:
Command line option | Description |
--hpx:print-counter |
print the specified performance counter either repeatedly and/or at the
times specified by --hpx:print-counter-at (see also option
--hpx:print-counter-interval ). |
--hpx:print-counter-reset |
print the specified performance counter either repeatedly and/or at the
times specified by --hpx:print-counter-at reset the counter after the
value is queried. (see also option --hpx:print-counter-interval ). |
--hpx:print-counter-interval |
print the performance counter(s) specified with --hpx:print-counter
repeatedly after the time interval (specified in milliseconds)
(default:0 which means print once at shutdown). |
--hpx:print-counter-destination |
print the performance counter(s) specified with --hpx:print-counter
to the given file (default: console)). |
--hpx:list-counters |
list the names of all registered performance counters. |
--hpx:list-counter-infos |
list the description of all registered performance counters. |
--hpx:print-counter-format |
print the performance counter(s) specified with --hpx:print-counter
possible formats in csv format with header or without any header (see
option --hpx:no-csv-header ), possible values: csv (prints counter
values in CSV format with full names as header) csv-short (prints
counter values in CSV format with shortnames provided with
--hpx:print-counter as --hpx:print-counter
shortname,full-countername ) |
--hpx:no-csv-header |
print the performance counter(s) specified with --hpx:print-counter
and csv or csv-short format specified with
--hpx:print-counter-format without header. |
--hpx:print-counter-at arg |
print the performance counter(s) specified with --hpx:print-counter
(or --hpx:print-counter-reset ) at the given point in time, possible
argument values: startup , shutdown (default), noshutdown . |
--hpx:reset-counters |
reset all performance counter(s) specified with --hpx:print-counter
after they have been evaluated) |
While the options --hpx:list-counters
and --hpx:list-counter-infos
give
a short listing of all available counters, the full documentation for those can
be found in the section Existing HPX performance counters.
A simple example¶
All of the commandline options mentioned above can be for instance tested using
the hello_world_distributed
example.
Listing all available counters hello_world_distributed --hpx:list-counters
yields:
List of available counter instances (replace * below with the appropriate
sequence number)
-------------------------------------------------------------------------
/agas/count/allocate /agas/count/bind /agas/count/bind_gid
/agas/count/bind_name ... /threads{locality#*/allocator#*}/count/objects
/threads{locality#*/total}/count/stack-recycles
/threads{locality#*/total}/idle-rate
/threads{locality#*/worker-thread#*}/idle-rate
Providing more information about all available counters
hello_world_distributed --hpx:list-counter-infos
yields:
Information about available counter instances (replace * below with the
appropriate sequence number)
------------------------------------------------------------------------------
fullname: /agas/count/allocate helptext: returns the number of invocations of
the AGAS service 'allocate' type: counter_raw version: 1.0.0
------------------------------------------------------------------------------
------------------------------------------------------------------------------
fullname: /agas/count/bind helptext: returns the number of invocations of the
AGAS service 'bind' type: counter_raw version: 1.0.0
------------------------------------------------------------------------------
------------------------------------------------------------------------------
fullname: /agas/count/bind_gid helptext: returns the number of invocations of
the AGAS service 'bind_gid' type: counter_raw version: 1.0.0
------------------------------------------------------------------------------
...
This command will not only list the counter names but also a short description of the data exposed by this counter.
Note
The list of available counters may differ depending on the concrete execution environment (hardware or software) of your application.
Requesting the counter data for one or more performance counters can be achieved
by invoking hello_world_distributed
with a list of counter names:
hello_world_distributed \
--hpx:print-counter=/threads{locality#0/total}/count/cumulative \
--hpx:print-counter=/agas{locality#0/total}/count/bind
which yields for instance:
hello world from OS-thread 0 on locality 0
/threads{locality#0/total}/count/cumulative,1,0.212527,[s],33
/agas{locality#0/total}/count/bind,1,0.212790,[s],11
The first line is the normal output generated by hello_world_distributed
and
has no relation to the counter data listed. The last two lines contain the
counter data as gathered at application shutdown. These lines have 6 fields, the
counter name, the sequence number of the counter invocation, the time stamp at
which this information has been sampled, the unit of measure for the time stamp,
the actual counter value, and an optional unit of measure for the counter value.
The actual counter value can be represented by a single number (for counters
returning singular values) or a list of numbers separated by ':'
(for
counters returning an array of values, like for instance a histogram).
Note
The name of the performance counter will be enclosed in double quotes '"'
if it contains one or more commas ','
.
Requesting to query the counter data once after a constant time interval with this command line:
hello_world_distributed \
--hpx:print-counter=/threads{locality#0/total}/count/cumulative \
--hpx:print-counter=/agas{locality#0/total}/count/bind \
--hpx:print-counter-interval=20
yields for instance (leaving off the actual console output of the
hello_world_distributed
example for brevity):
threads{locality#0/total}/count/cumulative,1,0.002409,[s],22
agas{locality#0/total}/count/bind,1,0.002542,[s],9
threads{locality#0/total}/count/cumulative,2,0.023002,[s],41
agas{locality#0/total}/count/bind,2,0.023557,[s],10
threads{locality#0/total}/count/cumulative,3,0.037514,[s],46
agas{locality#0/total}/count/bind,3,0.038679,[s],10
The command --hpx:print-counter-destination=<file>
will redirect all counter
data gathered to the specified file name, which avoids cluttering the console
output of your application.
The command line option --hpx:print-counter
supports using a limited set of
wildcards for a (very limited) set of use cases. In particular, all occurrences
of #*
as in locality#*
and in worker-thread#*
will be automatically
expanded to the proper set of performance counter names representing the actual
environment for the executed program. For instance, if your program is utilizing
4 worker threads for the execution of HPX threads (see command line option
--hpx:threads
) the following command line
hello_world_distributed \
--hpx:threads=4 \
--hpx:print-counter=/threads{locality#0/worker-thread#*}/count/cumulative
will print the value of the performance counters monitoring each of the worker threads:
hello world from OS-thread 1 on locality 0
hello world from OS-thread 0 on locality 0
hello world from OS-thread 3 on locality 0
hello world from OS-thread 2 on locality 0
/threads{locality#0/worker-thread#0}/count/cumulative,1,0.0025214,[s],27
/threads{locality#0/worker-thread#1}/count/cumulative,1,0.0025453,[s],33
/threads{locality#0/worker-thread#2}/count/cumulative,1,0.0025683,[s],29
/threads{locality#0/worker-thread#3}/count/cumulative,1,0.0025904,[s],33
The command --hpx:print-counter-format
takes values csv
and
csv-short
to generate CSV formatted counter values with header.
With format as csv:
hello_world_distributed \
--hpx:threads=2 \
--hpx:print-counter-format csv \
--hpx:print-counter /threads{locality#*/total}/count/cumulative \
--hpx:print-counter /threads{locality#*/total}/count/cumulative-phases
will print the values of performance counters in CSV format with full countername as header:
hello world from OS-thread 1 on locality 0
hello world from OS-thread 0 on locality 0
/threads{locality#*/total}/count/cumulative,/threads{locality#*/total}/count/cumulative-phases
39,93
With format csv-short:
hello_world_distributed \
--hpx:threads 2 \
--hpx:print-counter-format csv-short \
--hpx:print-counter cumulative,/threads{locality#*/total}/count/cumulative \
--hpx:print-counter phases,/threads{locality#*/total}/count/cumulative-phases
will print the values of performance counters in CSV format with short countername as header:
hello world from OS-thread 1 on locality 0
hello world from OS-thread 0 on locality 0
cumulative,phases
39,93
With format csv and csv-short when used with --hpx:print-counter-interval
:
hello_world_distributed \
--hpx:threads 2 \
--hpx:print-counter-format csv-short \
--hpx:print-counter cumulative,/threads{locality#*/total}/count/cumulative \
--hpx:print-counter phases,/threads{locality#*/total}/count/cumulative-phases \
--hpx:print-counter-interval 5
will print the header only once repeating the performance counter value(s) repeatedly:
cum,phases
25,42
hello world from OS-thread 1 on locality 0
hello world from OS-thread 0 on locality 0
44,95
The command --hpx:no-csv-header
to be used with
--hpx:print-counter-format
to print performance counter values in CSV format
without any header:
hello_world_distributed \
--hpx:threads 2 \
--hpx:print-counter-format csv-short \
--hpx:print-counter cumulative,/threads{locality#*/total}/count/cumulative \
--hpx:print-counter phases,/threads{locality#*/total}/count/cumulative-phases \
--hpx:no-csv-header
will print:
hello world from OS-thread 1 on locality 0
hello world from OS-thread 0 on locality 0
37,91
Consuming performance counter data using the HPX API¶
HPX provides an API allowing to discover performance counters and to retrieve the current value of any existing performance counter from any application.
Discover existing performance counters¶
Retrieve the current value of any performance counter¶
Performance counters are specialized HPX components. In order to retrieve a counter value, the performance counter needs to be instantiated. HPX exposes a client component object for this purpose:
hpx::performance_counters::performance_counter counter(std::string const& name);
Instantiating an instance of this type will create the performance counter
identified by the given name
. Only the first invocation for any given counter
name will create a new instance of that counter, all following invocations for a
given counter name will reference the initially created instance. This ensures,
that at any point in time there is always not more than one active instance of
any of the existing performance counters.
In order to access the counter value (or invoking any of the other functionality
related to a performance counter, like start
, stop
or reset
) member
functions of the created client component instance should be called:
// print the current number of threads created on locality 0
hpx::performance_counters::performance_counter count(
"/threads{locality#0/total}/count/cumulative");
hpx::cout << count.get_value<int>().get() << hpx::endl;
For more information about the client component type see [classref hpx::performance_counters::performance_counter].
Note
In the above example count.get_value()
returns a future. In order to print
the result we must append .get()
to retrieve the value. You could write the
above example like this for more clarity:
// print the current number of threads created on locality 0
hpx::performance_counters::performance_counter count(
"/threads{locality#0/total}/count/cumulative");
hpx::future<int> result = count.get_value<int>();
hpx::cout << result.get() << hpx::endl;
Providing performance counter data¶
HPX offers several ways by which you may provide your own data as a performance counter. This has the benefit of exposing additional, possibly application specific information using the existing Performance Counter framework, unifying the process of gathering data about your application.
An application that wants to provide counter data can implement a Performance Counter to provide the data. When a consumer queries performance data, the HPX runtime system calls the provider to collect the data. The runtime system uses an internal registry to determine which provider to call.
Generally, there two ways of exposing your own Performance Counter data: a simple, function based way and a more complex, but more powerful way of implementing a full Performance Counter. Both alternatives are described in the following sections.
Exposing performance counter data using a simple function¶
The simplest way to expose arbitrary numeric data is to write a function which will then be called whenever a consumer queries this counter. Currently, this type of Performance Counter can only be used to expose integer values. The expected signature of this function is:
std::int64_t some_performance_data(bool reset);
The argument bool reset
(which is supplied by the runtime system when the
function is invoked) specifies whether the counter value should be reset after
evaluating the current value (if applicable).
For instance, here is such a function returning how often it was invoked:
// The atomic variable 'counter' ensures the thread safety of the counter.
boost::atomic<std::int64_t> counter(0);
std::int64_t some_performance_data(bool reset)
{
std::int64_t result = ++counter;
if (reset)
counter = 0;
return result;
}
This example function exposes a linearly increasing value as our performance data. The value is incremented on each invocation, e.g. each time a consumer requests the counter data of this Performance Counter.
The next step in exposing this counter to the runtime system is to register the
function as a new raw counter type using the HPX API function
hpx::performance_counters::install_counter_type
. A counter type
represents certain common characteristics of counters, like their counter type
name, and any associated description information. The following snippet shows an
example of how to register the function some_performance_data
which is shown
above for a counter type named "/test/data"
. This registration has to be
executed before any consumer instantiates and queries an instance of this
counter type:
#include <hpx/include/performance_counters.hpp>
void register_counter_type()
{
// Call the HPX API function to register the counter type.
hpx::performance_counters::install_counter_type(
"/test/data", // counter type name
&some_performance_data, // function providing counter data
"returns a linearly increasing counter value" // description text (optional)
"" // unit of measure (optional)
);
}
Now it is possible to instantiate a new counter instance based on the naming
scheme "/test{locality#*/total}/data"
where *
is a zero based integer
index identifying the locality for which the counter instance should be
accessed. The function
hpx::performance_counters::install_counter_type
enables to
instantiate exactly one counter instance for each locality. Repeated
requests to instantiate such a counter will return the same instance, e.g. the
instance created for the first request.
If this counter needs to be accessed using the standard HPX command line
options, the registration has to be performed during application startup, before
hpx_main
is executed. The best way to achieve this is to register an HPX
startup function using the API function
hpx::register_startup_function
before calling hpx::init
to
initialize the runtime system:
int main(int argc, char* argv[])
{
// By registering the counter type we make it available to any consumer
// who creates and queries an instance of the type "/test/data".
//
// This registration should be performed during startup. The
// function 'register_counter_type' should be executed as an HPX thread right
// before hpx_main is executed.
hpx::register_startup_function(®ister_counter_type);
// Initialize and run HPX.
return hpx::init(argc, argv);
}
Please see the code in [hpx_link examples/performance_counters/simplest_performance_counter.cpp..simplest_performance_counter.cpp] for a full example demonstrating this functionality.
Implementing a full performance counter¶
Sometimes, the simple way of exposing a single value as a Performance Counter is not sufficient. For that reason, HPX provides a means of implementing full Performance Counters which support:
- Retrieving the descriptive information about the Performance Counter
- Retrieving the current counter value
- Resetting the Performance Counter (value)
- Starting the Performance Counter
- Stopping the Performance Counter
- Setting the (initial) value of the Performance Counter
Every full Performance Counter will implement a predefined interface:
// Copyright (c) 2007-2018 Hartmut Kaiser
//
// Distributed under the Boost Software License, Version 1.0. (See accompanying
// file LICENSE_1_0.txt or copy at http://www.boost.org/LICENSE_1_0.txt)
#if !defined(HPX_PERFORMANCE_COUNTERS_PERFORMANCE_COUNTER_JAN_18_2013_0939AM)
#define HPX_PERFORMANCE_COUNTERS_PERFORMANCE_COUNTER_JAN_18_2013_0939AM
#include <hpx/config.hpp>
#include <hpx/lcos/future.hpp>
#include <hpx/runtime/components/client_base.hpp>
#include <hpx/runtime/launch_policy.hpp>
#include <hpx/util/bind_front.hpp>
#include <hpx/performance_counters/counters_fwd.hpp>
#include <hpx/performance_counters/stubs/performance_counter.hpp>
#include <string>
#include <utility>
#include <vector>
///////////////////////////////////////////////////////////////////////////////
namespace hpx { namespace performance_counters
{
///////////////////////////////////////////////////////////////////////////
struct HPX_EXPORT performance_counter
: components::client_base<performance_counter, stubs::performance_counter>
{
typedef components::client_base<
performance_counter, stubs::performance_counter
> base_type;
performance_counter() {}
performance_counter(std::string const& name);
performance_counter(std::string const& name, hpx::id_type const& locality);
performance_counter(future<id_type> && id)
: base_type(std::move(id))
{}
performance_counter(hpx::future<performance_counter> && c)
: base_type(std::move(c))
{}
///////////////////////////////////////////////////////////////////////
future<counter_info> get_info() const;
counter_info get_info(launch::sync_policy,
error_code& ec = throws) const;
future<counter_value> get_counter_value(bool reset = false);
counter_value get_counter_value(launch::sync_policy,
bool reset = false, error_code& ec = throws);
future<counter_value> get_counter_value() const;
counter_value get_counter_value(launch::sync_policy,
error_code& ec = throws) const;
future<counter_values_array> get_counter_values_array(bool reset = false);
counter_values_array get_counter_values_array(launch::sync_policy,
bool reset = false, error_code& ec = throws);
future<counter_values_array> get_counter_values_array() const;
counter_values_array get_counter_values_array(launch::sync_policy,
error_code& ec = throws) const;
///////////////////////////////////////////////////////////////////////
future<bool> start();
bool start(launch::sync_policy, error_code& ec = throws);
future<bool> stop();
bool stop(launch::sync_policy, error_code& ec = throws);
future<void> reset();
void reset(launch::sync_policy, error_code& ec = throws);
future<void> reinit(bool reset = true);
void reinit(
launch::sync_policy, bool reset = true, error_code& ec = throws);
///////////////////////////////////////////////////////////////////////
future<std::string> get_name() const;
std::string get_name(launch::sync_policy, error_code& ec = throws) const;
private:
template <typename T>
static T extract_value(future<counter_value> && value)
{
return value.get().get_value<T>();
}
public:
template <typename T>
future<T> get_value(bool reset = false)
{
return get_counter_value(reset).then(
hpx::launch::sync,
util::bind_front(
&performance_counter::extract_value<T>));
}
template <typename T>
T get_value(launch::sync_policy, bool reset = false,
error_code& ec = throws)
{
return get_counter_value(launch::sync, reset).get_value<T>(ec);
}
template <typename T>
future<T> get_value() const
{
return get_counter_value().then(
hpx::launch::sync,
util::bind_front(
&performance_counter::extract_value<T>));
}
template <typename T>
T get_value(launch::sync_policy, error_code& ec = throws) const
{
return get_counter_value(launch::sync).get_value<T>(ec);
}
};
/// Return all counters matching the given name (with optional wildcards).
HPX_API_EXPORT std::vector<performance_counter> discover_counters(
std::string const& name, error_code& ec = throws);
}}
#endif
In order to implement a full Performance Counter you have to create an HPX
component exposing this interface. To simplify this task, HPX provides a ready
made base class which handles all the boiler plate of creating a component for
you. The remainder of this section will explain the process of creating a full
Performance Counter based on the Sine example which you can find in the
directory examples/performance_counters/sine/
.
The base class is defined in the header file [hpx_link hpx/performance_counters/base_performance_counter.hpp..hpx/performance_counters/base_performance_counter.hpp] as:
// Copyright (c) 2007-2018 Hartmut Kaiser
//
// Distributed under the Boost Software License, Version 1.0. (See accompanying
// file LICENSE_1_0.txt or copy at http://www.boost.org/LICENSE_1_0.txt)
#if !defined(HPX_PERFORMANCE_COUNTERS_BASE_PERFORMANCE_COUNTER_JAN_18_2013_1036AM)
#define HPX_PERFORMANCE_COUNTERS_BASE_PERFORMANCE_COUNTER_JAN_18_2013_1036AM
#include <hpx/config.hpp>
#include <hpx/performance_counters/counters.hpp>
#include <hpx/performance_counters/server/base_performance_counter.hpp>
#include <hpx/runtime/actions/component_action.hpp>
#include <hpx/runtime/components/component_type.hpp>
#include <hpx/runtime/components/server/component_base.hpp>
///////////////////////////////////////////////////////////////////////////////
//[performance_counter_base_class
namespace hpx { namespace performance_counters
{
template <typename Derived>
class base_performance_counter;
}}
//]
///////////////////////////////////////////////////////////////////////////////
namespace hpx { namespace performance_counters
{
template <typename Derived>
class base_performance_counter
: public hpx::performance_counters::server::base_performance_counter,
public hpx::components::component_base<Derived>
{
private:
typedef hpx::components::component_base<Derived> base_type;
public:
typedef Derived type_holder;
typedef hpx::performance_counters::server::base_performance_counter
base_type_holder;
base_performance_counter()
{}
base_performance_counter(hpx::performance_counters::counter_info const& info)
: base_type_holder(info)
{}
// Disambiguate finalize() which is implemented in both base classes
void finalize()
{
base_type_holder::finalize();
base_type::finalize();
}
};
}}
#endif
The single template parameter is expected to receive the type of the derived class implementing the Performance Counter. In the Sine example this looks like:
// Copyright (c) 2007-2012 Hartmut Kaiser
//
// Distributed under the Boost Software License, Version 1.0. (See accompanying
// file LICENSE_1_0.txt or copy at http://www.boost.org/LICENSE_1_0.txt)
#if !defined(PERFORMANCE_COUNTERS_SINE_SEP_20_2011_0112PM)
#define PERFORMANCE_COUNTERS_SINE_SEP_20_2011_0112PM
#include <hpx/hpx.hpp>
#include <hpx/util/interval_timer.hpp>
#include <hpx/lcos/local/spinlock.hpp>
#include <hpx/performance_counters/base_performance_counter.hpp>
#include <cstdint>
namespace performance_counters { namespace sine { namespace server
{
///////////////////////////////////////////////////////////////////////////
//[sine_counter_definition
class sine_counter
: public hpx::performance_counters::base_performance_counter<sine_counter>
//]
{
public:
sine_counter() : current_value_(0) {}
sine_counter(hpx::performance_counters::counter_info const& info);
/// This function will be called in order to query the current value of
/// this performance counter
hpx::performance_counters::counter_value get_counter_value(bool reset);
/// The functions below will be called to start and stop collecting
/// counter values from this counter.
bool start();
bool stop();
/// finalize() will be called just before the instance gets destructed
void finalize();
protected:
bool evaluate();
private:
typedef hpx::lcos::local::spinlock mutex_type;
mutable mutex_type mtx_;
double current_value_;
std::uint64_t evaluated_at_;
hpx::util::interval_timer timer_;
};
}}}
#endif
i.e. the type sine_counter
is derived from the base class passing the type
as a template argument (please see [hpx_link
examples/performance_counters/sine/server/sine.hpp..sine.hpp] for the full
source code of the counter definition). For more information about this
technique (called Curiously Recurring Template Pattern - CRTP), please see for
instance the corresponding Wikipedia article. This base
class itself is derived from the performance_counter
interface described
above.
Additionally, a full Performance Counter implementation not only exposes the actual value but also provides information about
- The point in time a particular value was retrieved
- A (sequential) invocation count
- The actual counter value
- An optional scaling coefficient
- Information about the counter status
Existing HPX performance counters¶
The HPX runtime system exposes a wide variety of predefined Performance Counters. These counters expose critical information about different modules of the runtime system. They can help determine system bottlenecks and fine-tune system and application performance.
Counter type | Counter instance formatting | Description | Parameters |
where:
primary namespace services: component namespace services: locality namespace services: symbol namespace services: |
where:
The value for |
None | Returns the total number of invocations of the specified AGAS service since its creation. |
where:
|
where:
|
None | Returns the overall total number of invocations of all AGAS services provided by the given AGAS service category since its creation. |
where:
primary namespace services: component namespace services: locality namespace services: symbol namespace services: |
where:
The value for |
None | Returns the overall execution time of the specified AGAS service since its creation (in nanoseconds). |
where:
|
where:
|
None | Returns the overall execution time of all AGAS services provided by the given AGAS service category since its creation (in nanoseconds). |
/agas/count/entries |
where:
|
None | Returns the number of cache entries resident in the AGAS cache of
the specified locality (see <cache_statistics> ). |
where:
|
where:
|
None | Returns the number of cache events (evictions, hits, inserts, and misses)
in the AGAS cache of the specified locality (see
<cache_statistics> ). |
where:
|
where:
|
None | Returns the number of invocations of the specified cache API function of the AGAS cache. |
where:
|
where:
|
None | Returns the overall time spent executing of the specified API function of the AGAS cache. |
Counter type | Counter instance formatting | Description | Parameters |
where:
|
where:
|
Returns the overall number of raw (uncompressed) bytes sent or received
(see The performance counters for the connection type Please see CMake variables used to configure HPX for more details. |
None |
where:
|
where:
|
Returns the total time (in nanoseconds) between the start of each
asynchronous transmission operation and the end of the corresponding
operation for the specified The performance counters for the connection type Please see CMake variables used to configure HPX for more details. |
None |
where:
|
where:
|
Returns the overall number of bytes transferred (see The performance counters for the connection type Please see CMake variables used to configure HPX for more details. |
If the configure-time option -DHPX_WITH_PARCELPORT_ACTION_COUNTERS=On
was specified, this counter allows to specify an optional action name as
its parameter. In this case the counter will report the number of bytes
transmitted for the given action only. |
where:
|
where:
|
Returns the overall time spent performing outgoing data serialization for
the specified The performance counters for the connection type Please see CMake variables used to configure HPX for more details. |
If the configure-time option -DHPX_WITH_PARCELPORT_ACTION_COUNTERS=On
was specified, this counter allows to specify an optional action name as
its parameter. In this case the counter will report the serialization
time for the given action only. |
/parcels/count/routed |
where:
|
Returns the overall number of routed (outbound) parcels transferred by the given locality. Routed parcels are those which cannot directly be delivered to its destination as the local AGAS is not able to resolve the destination address. In this case a parcel is sent to the AGAS service component which is responsible for creating the destination GID (and is responsible for resolving the destination address). This AGAS service component will deliver the parcel to its final target. |
If the configure-time option -DHPX_WITH_PARCELPORT_ACTION_COUNTERS=On
was specified, this counter allows to specify an optional action name as
its parameter. In this case the counter will report the number of parcels
for the given action only. |
where:
|
where:
|
Returns the overall number of parcels transferred using the specified
The performance counters for the connection type Please see CMake variables used to configure HPX for more details. |
None |
where:
|
where:
|
Returns the overall number of messages [1] transferred using the
specified The performance counters for the connection type Please see CMake variables used to configure HPX for more details. |
None |
where:
<connection_type` is one of the following: |
where:
|
Returns the overall number cache events (evictions, hits, inserts,
misses, and reclaims) for the connection cache of the given connection
type on the given locality (see The performance counters for the connection type Please see CMake variables used to configure HPX for more details. |
None |
where:
|
where:
|
Returns the current number of parcels stored in the parcel queue (see
<operation for which queue to query, e.g. sent or received ). |
None |
Counter type | Counter instance formatting | Description | Parameters |
/threads/count/cumulative |
where:
|
Returns the overall number of executed (retired) HPX-threads on the
given locality since application start. If the instance name is
total the counter returns the accumulated number of retired
HPX-threads for all worker threads (cores) on that locality. If
the instance name is worker-thread#* the counter will return the
overall number of retired HPX-threads for all worker threads
separately. This counter is available only if the configuration time
constant HPX_WITH_THREAD_CUMULATIVE_COUNTS is set to ON (default:
ON ). |
None |
/threads/time/average |
where:
|
Returns the average time spent executing one HPX-thread on the given
locality since application start. If the instance name is total
the counter returns the average time spent executing one HPX-thread for
all worker threads (cores) on that locality. If the instance name
is worker-thread#* the counter will return the average time spent
executing one HPX-thread for all worker threads separately. This counter
is available only if the configuration time constants
HPX_WITH_THREAD_CUMULATIVE_COUNTS (default: ON ) and
HPX_WITH_THREAD_IDLE_RATES are set to ON (default: OFF ). The unit
of measure for this counter is nanosecond [ns]. |
None |
/threads/time/average-overhead |
where:
|
Returns the average time spent on overhead while executing one
HPX-thread on the given locality since application start. If
the instance name is total the counter returns the average time spent
on overhead while executing one HPX-thread for all worker threads
(cores) on that locality. If the instance name is
worker-thread#* the counter will return the average time spent on
overhead executing one HPX-thread for all worker threads separately.
This counter is available only if the configuration time constants
HPX_WITH_THREAD_CUMULATIVE_COUNTS (default: ON ) and
HPX_WITH_THREAD_IDLE_RATES are set to ON (default: OFF ). The
unit of measure for this counter is nanosecond [ns]. |
None |
/threads/count/cumulative-phases |
where:
|
Returns the overall number of executed HPX-thread phases (invocations)
on the given locality since application start. If the instance
name is total the counter returns the accumulated number of executed
HPX-thread phases (invocations) for all worker threads (cores) on that
locality. If the instance name is worker-thread#* the counter
will return the overall number of executed HPX-thread phases for all
worker threads separately. This counter is available only if the
configuration time constant HPX_WITH_THREAD_CUMULATIVE_COUNTS is set
to ON (default: ON ). The unit of measure for this counter is
nanosecond [ns]. |
None |
/threads/time/average-phase |
where:
|
Returns the average time spent executing one HPX-thread phase
(invocation) on the given locality since application start. If
the instance name is total the counter returns the average time spent
executing one HPX-thread phase (invocation) for all worker threads
(cores) on that locality. If the instance name is
worker-thread#* the counter will return the average time spent
executing one HPX-thread phase for all worker threads separately. This
counter is available only if the configuration time constants
HPX_WITH_THREAD_CUMULATIVE_COUNTS (default: ON ) and
HPX_WITH_THREAD_IDLE_RATES are set to ON (default: OFF ). The
unit of measure for this counter is nanosecond [ns]. |
None |
/threads/time/average-phase-overhead |
where:
|
Returns the average time spent on overhead executing one HPX-thread
phase (invocation) on the given locality since application start.
If the instance name is total the counter returns the average time
spent on overhead while executing one HPX-thread phase (invocation) for
all worker threads (cores) on that locality. If the instance name
is worker-thread#* the counter will return the average time spent on
overhead executing one HPX-thread phase for all worker threads
separately. This counter is available only if the configuration time
constants HPX_WITH_THREAD_CUMULATIVE_COUNTS (default: ON ) and
HPX_WITH_THREAD_IDLE_RATES are set to ON (default: OFF ). The
unit of measure for this counter is nanosecond [ns]. |
None |
/threads/time/overall |
where:
|
Returns the overall time spent running the scheduler on the given
locality since application start. If the instance name is total
the counter returns the overall time spent running the scheduler for all
worker threads (cores) on that locality. If the instance name is
worker-thread#* the counter will return the overall time spent running
the scheduler for all worker threads separately. This counter is available
only if the configuration time constant HPX_WITH_THREAD_IDLE_RATES is
set to ON (default: OFF ). The unit of measure for this counter is
nanosecond [ns]. |
None |
/threads/time/cumulative |
where:
|
Returns the overall time spent executing all HPX-threads on the given
locality since application start. If the instance name is total
the counter returns the overall time spent executing all HPX-threads for
all worker threads (cores) on that locality. If the instance name
is worker-thread#* the counter will return the overall time spent
executing all HPX-threads for all worker threads separately. This counter
is available only if the configuration time constants
HPX_THREAD_MAINTAIN_CUMULATIVE_COUNTS (default: ON ) and
HPX_THREAD_MAINTAIN_IDLE_RATES are set to ON (default: OFF ). |
None |
/threads/time/cumulative-overheads |
where:
|
Returns the overall overhead time incurred executing all HPX-threads on
the given locality since application start. If the instance name
is total the counter returns the overall overhead time incurred
executing all HPX-threads for all worker threads (cores) on that
locality. If the instance name is worker-thread#* the counter
will return the overall overhead time incurred executing all
HPX-threads for all worker threads separately. This counter is
available only if the configuration time constants
HPX_THREAD_MAINTAIN_CUMULATIVE_COUNTS (default: ON ) and
HPX_THREAD_MAINTAIN_IDLE_RATES are set to ON (default: OFF ).
The unit of measure for this counter is nanosecond [ns]. |
None |
where:
|
where:
The |
Returns the current number of HPX-threads having the given thread state
on the given locality. If the instance name is total the
counter returns the current number of HPX-threads of the given state
for all worker threads (cores) on that locality. If the instance
name is worker-thread#* the counter will return the current number of
HPX-threads in the given state for all worker threads separately. |
None |
where:
|
where:
The |
Returns the average wait time of HPX-threads (if the thread state is
These counters are available only if the compile time constant
|
None |
/threads/idle-rate |
where:
|
Returns the average idle rate for the given worker thread(s) on the given
locality. The idle rate is defined as the ratio of the time spent
on scheduling and management tasks and the overall time spent executing
work since the application started. This counter is available only if the
configuration time constant HPX_WITH_THREAD_IDLE_RATES is set to ON
(default: OFF ). |
None |
/threads/creation-idle-rate |
where:
|
Returns the average idle rate for the given worker thread(s) on the given
locality which is caused by creating new threads. The creation idle
rate is defined as the ratio of the time spent on creating new threads and
the overall time spent executing work since the application started. This
counter is available only if the configuration time constants
HPX_WITH_THREAD_IDLE_RATES (default: OFF ) and
HPX_WITH_THREAD_CREATION_AND_CLEANUP_RATES are set to ON . |
None |
/threads/cleanup-idle-rate |
where:
|
Returns the average idle rate for the given worker thread(s) on the given
locality which is caused by cleaning up terminated threads. The
cleanup idle rate is defined as the ratio of the time spent on cleaning up
terminated thread objects and the overall time spent executing work since
the application started. This counter is available only if the
configuration time constants HPX_WITH_THREAD_IDLE_RATES (default:
OFF ) and HPX_WITH_THREAD_CREATION_AND_CLEANUP_RATES are set to
ON . |
None |
/threadqueue/length |
where:
|
Returns the overall length of all queues for the given worker thread(s) on the given locality. | None |
/threads/count/stack-unbinds |
where:
|
Returns the total number of HPX-thread unbind (madvise) operations performed for the referenced locality. Note that this counter is not available on Windows based platforms. | None |
/threads/count/stack-recycles |
where:
|
Returns the total number of HPX-thread recycling operations performed. | None |
/threads/count/stolen-from-pending |
|
Returns the total number of HPX-threads ‘stolen’ from the pending
thread queue by a neighboring thread worker thread (these threads are
executed by a different worker thread than they were initially scheduled
on). This counter is available only if the configuration time constant
HPX_WITH_THREAD_STEALING_COUNTS is set to ON (default: ON ). |
None |
/threads/count/pending-misses |
where:
|
Returns the total number of times that the referenced worker-thread on
the referenced locality failed to find pending HPX-threads in
its associated queue. This counter is available only if the configuration
time constant HPX_WITH_THREAD_STEALING_COUNTS is set to ON
(default: ON ). |
None |
/threads/count/pending-accesses |
where:
|
Returns the total number of times that the referenced worker-thread on
the referenced locality looked for pending HPX-threads in its
associated queue. This counter is available only if the configuration
time constant HPX_WITH_THREAD_STEALING_COUNTS is set to ON
(default: ON ). |
None |
/threads/count/stolen-from-staged |
where:
|
Returns the total number of HPX-threads ‘stolen’ from the staged thread
queue by a neighboring worker thread (these threads are executed by a
different worker thread than they were initially scheduled on). This
counter is available only if the configuration time constant
HPX_WITH_THREAD_STEALING_COUNTS is set to ON (default: ON ). |
None |
/threads/count/stolen-to-pending |
where:
|
Returns the total number of HPX-threads ‘stolen’ to the pending thread
queue of the worker thread (these threads are executed by a different
worker thread than they were initially scheduled on). This counter is
available only if the configuration time constant
HPX_WITH_THREAD_STEALING_COUNTS is set to ON (default: ON ). |
None |
/threads/count/stolen-to-staged |
where:
|
Returns the total number of HPX-threads ‘stolen’ to the staged thread
queue of a neighboring worker thread (these threads are executed by a
different worker thread than they were initially scheduled on). This
counter is available only if the configuration time constant
HPX_WITH_THREAD_STEALING_COUNTS is set to ON (default: ON ). |
None |
/threads/count/objects |
where:
|
Returns the total number of HPX-thread objects created. Note that thread objects are reused to improve system performance, thus this number does not reflect the number of actually executed (retired) HPX-threads. | None |
/scheduler/utilization/instantaneous |
where:
|
|
Percent |
/threads/idle-loop-count/instantaneous |
where:
|
Returns the current (instantaneous) idle-loop count for the given HPX- worker thread or the accumulated value for all worker threads. | None |
/threads/busy-loop-count/instantaneous |
where:
|
Returns the current (instantaneous) busy-loop count for the given HPX- worker thread or the accumulated value for all worker threads. | None |
/threads/time/background-work-duration |
where:
|
Returns the overall time spent performing background work on the given
locality since application start. If the instance name is total the
counter returns the overall time spent performing background work for all
worker threads (cores) on that locality. If the instance name is
worker-thread#* the counter will return the overall time spent
performing background work for all worker threads separately. This
counter is available only if the configuration time constants
HPX_WITH_BACKGROUND_THREAD_COUNTERS (default: OFF ) and
HPX_WITH_THREAD_IDLE_RATES are set to ON (default: OFF ). The
unit of measure for this counter is nanosecond [ns]. |
None |
/threads/background-overhead |
where:
|
Returns the background overhead on the given locality since application
start. If the instance name is total the counter returns the
background overhead for all worker threads (cores) on that locality. If
the instance name is worker-thread#* the counter will return
background overhead for all worker threads separately. This counter is
available only if the configuration time constants
HPX_WITH_BACKGROUND_THREAD_COUNTERS (default: OFF ) and
HPX_WITH_THREAD_IDLE_RATES are set to ON (default: OFF ). The
unit of measure displayed for this counter is 0.1%. |
None |
Counter type | Counter instance formatting | Description | Parameters |
/runtime/count/component |
where:
|
Returns the overall number of currently active components of the specified type on the given locality. | The type of the component. This is the string which has been used while
registering the component with HPX, e.g. which has been passed as the
second parameter to the macro HPX_REGISTER_COMPONENT . |
/runtime/count/action-invocation |
|
Returns the overall (local) invocation count of the specified action type on the given locality. | The action type. This is the string which has been used while registering
the action with HPX, e.g. which has been passed as the second parameter
to the macro HPX_REGISTER_ACTION or
HPX_REGISTER_ACTION_ID . |
/runtime/count/remote-action-invocation |
where:
|
Returns the overall (remote) invocation count of the specified action type on the given locality. | The action type. This is the string which has been used while registering
the action with HPX, e.g. which has been passed as the second parameter
to the macro HPX_REGISTER_ACTION or
HPX_REGISTER_ACTION_ID . |
/runtime/uptime |
where:
|
Returns the overall time since application start on the given locality in nanoseconds. | None |
/runtime/memory/virtual |
where:
|
Returns the amount of virtual memory currently allocated by the referenced locality (in bytes). | None |
/runtime/memory/resident |
where:
|
Returns the amount of resident memory currently allocated by the referenced locality (in bytes). | None |
/runtime/memory/total |
where:
|
|
None |
/runtime/io/read_bytes_issued |
where:
|
Returns the number of bytes read by the process (aggregate of count arguments passed to read() call or its analogues). This performance counter is available only on systems which expose the related data through the /proc file system. | None |
/runtime/io/write_bytes_issued |
where:
|
Returns the number of bytes written by the process (aggregate of count arguments passed to write() call or its analogues). This performance counter is available only on systems which expose the related data through the /proc file system. | None |
/runtime/io/read_syscalls |
where:
|
Returns the number of system calls that perform I/O reads. This performance counter is available only on systems which expose the related data through the /proc file system. | None |
/runtime/io/write_syscalls |
where:
|
Returns the number of system calls that perform I/O writes. This performance counter is available only on systems which expose the related data through the /proc file system. | None |
/runtime/io/read_bytes_transferred |
where:
|
Returns the number of bytes retrieved from storage by I/O operations. This performance counter is available only on systems which expose the related data through the /proc file system. | None |
/runtime/io/write_bytes_transferred |
where:
|
Returns the number of bytes retrieved from storage by I/O operations. This performance counter is available only on systems which expose the related data through the /proc file system. | None |
/runtime/io/write_bytes_cancelled |
where:
|
Returns the number of bytes accounted by write_bytes_transferred that has not been ultimately stored due to truncation or deletion. This performance counter is available only on systems which expose the related data through the /proc file system. | None |
Counter type | Counter instance formatting | Description | Parameters |
where:
For a full list of available PAPI events and their (short) description
use the |
where:
|
This counter returns the current count of occurrences of the specified
PAPI event. This counter is available only if the configuration time
constant HPX_WITH_PAPI is set to ON (default: OFF ). |
None |
Counter type | Counter instance formatting | Description | Parameters |
/statistics/average |
Any full performance counter name. The referenced performance counter is queried at fixed time intervals as specified by the first parameter. | Returns the current average (mean) value calculated based on the values queried from the underlying counter (the one specified as the instance name). | Any parameter will be interpreted as a list of up to two comma separated
(integer) values, where the first is the time interval (in milliseconds)
at which the underlying counter should be queried. If no value is
specified, the counter will assume 1000 [ms] as the default. The
second value can be either 0 or 1 and specifies whether the
underlying counter should be reset during evaluation 1 or not 0 .
The default value is 0 . |
/statistics/rolling_average |
Any full performance counter name. The referenced performance counter is queried at fixed time intervals as specified by the first parameter. | Returns the current rolling average (mean) value calculated based on the values queried from the underlying counter (the one specified as the instance name). | Any parameter will be interpreted as a list of up to three comma
separated (integer) values, where the first is the time interval (in
milliseconds) at which the underlying counter should be queried. If no
value is specified, the counter will assume 1000 [ms] as the default.
The second value will be interpreted as the size of the rolling window
(the number of latest values to use to calculate the rolling average).
The default value for this is 10 . The third value can be
either 0 or 1 and specifies whether the underlying counter should
be reset during evaluation 1 or not 0 . The default value is 0 . |
/statistics/stddev |
Any full performance counter name. The referenced performance counter is queried at fixed time intervals as specified by the first parameter. | Returns the current standard deviation (stddev) value calculated based on the values queried from the underlying counter (the one specified as the instance name). | Any parameter will be interpreted as a list of up to two comma separated
(integer) values, where the first is the time interval (in milliseconds)
at which the underlying counter should be queried. If no value is
specified, the counter will assume 1000 [ms] as the default. The
second value can be either 0 or 1 and specifies whether the
underlying counter should be reset during evaluation 1 or not 0 .
The default value is 0 . |
/statistics/rolling_stddev |
Any full performance counter name. The referenced performance counter is queried at fixed time intervals as specified by the first parameter. | Returns the current rolling variance (stddev) value calculated based on the values queried from the underlying counter (the one specified as the instance name). | Any parameter will be interpreted as a list of up to three comma
separated (integer) values, where the first is the time interval (in
milliseconds) at which the underlying counter should be queried. If no
value is specified, the counter will assume 1000 [ms] as the default.
The second value will be interpreted as the size of the rolling window
(the number of latest values to use to calculate the rolling average).
The default value for this is 10 . The third value can be either 0
or 1 and specifies whether the underlying counter should be reset
during evaluation 1 or not 0 . The default value is 0 . |
/statistics/median |
Any full performance counter name. The referenced performance counter is queried at fixed time intervals as specified by the first parameter. | Returns the current (statistically estimated) median value calculated based on the values queried from the underlying counter (the one specified as the instance name). | Any parameter will be interpreted as a list of up to two comma separated
(integer) values, where the first is the time interval (in milliseconds)
at which the underlying counter should be queried. If no value is
specified, the counter will assume 1000 [ms] as the default. The
second value can be either 0 or 1 and specifies whether the
underlying counter should be reset during evaluation 1 or not 0 .
The default value is 0 . |
/statistics/max |
Any full performance counter name. The referenced performance counter is queried at fixed time intervals as specified by the first parameter. | Returns the current maximum value calculated based on the values queried from the underlying counter (the one specified as the instance name). | Any parameter will be interpreted as a list of up to two comma separated
(integer) values, where the first is the time interval (in milliseconds)
at which the underlying counter should be queried. If no value is
specified, the counter will assume 1000 [ms] as the default. The
second value can be either 0 or 1 and specifies whether the
underlying counter should be reset during evaluation 1 or not 0 .
The default value is 0 . |
/statistics/rolling_max |
Any full performance counter name. The referenced performance counter is queried at fixed time intervals as specified by the first parameter. | Returns the current rolling maximum value calculated based on the values queried from the underlying counter (the one specified as the instance name). | Any parameter will be interpreted as a list of up to three comma
separated (integer) values, where the first is the time interval (in
milliseconds) at which the underlying counter should be queried. If no
value is specified, the counter will assume 1000 [ms] as the default.
The second value will be interpreted as the size of the rolling window
(the number of latest values to use to calculate the rolling average).
The default value for this is 10 . The third value can be either 0
or 1 and specifies whether the underlying counter should be reset
during evaluation 1 or not 0 . The default value is 0 . |
/statistics/min |
Any full performance counter name. The referenced performance counter is queried at fixed time intervals as specified by the first parameter. | Returns the current minimum value calculated based on the values queried from the underlying counter (the one specified as the instance name). | Any parameter will be interpreted as a list of up to two comma separated
(integer) values, where the first is the time interval (in milliseconds)
at which the underlying counter should be queried. If no value is
specified, the counter will assume 1000 [ms] as the default. The
second value can be either 0 or 1 and specifies whether the
underlying counter should be reset during evaluation 1 or not 0 .
The default value is 0 . |
/statistics/rolling_min |
Any full performance counter name. The referenced performance counter is queried at fixed time intervals as specified by the first parameter. | Returns the current rolling minimum value calculated based on the values queried from the underlying counter (the one specified as the instance name). | Any parameter will be interpreted as a list of up to three comma
separated (integer) values, where the first is the time interval (in
milliseconds) at which the underlying counter should be queried. If no
value is specified, the counter will assume 1000 [ms] as the default.
The second value will be interpreted as the size of the rolling window
(the number of latest values to use to calculate the rolling average).
The default value for this is 10 . The third value can be either 0
or 1 and specifies whether the underlying counter should be reset
during evaluation 1 or not 0 . The default value is 0 . |
Counter type | Counter instance formatting | Description | Parameters |
/arithmetics/add |
None | Returns the sum calculated based on the values queried from the underlying counters (the ones specified as the parameters). | The parameter will be interpreted as a comma separated list of full performance counter names which are queried whenever this counter is accessed. Any wildcards in the counter names will be expanded. |
/arithmetics/subtract |
None | Returns the difference calculated based on the values queried from the underlying counters (the ones specified as the parameters). | The parameter will be interpreted as a comma separated list of full performance counter names which are queried whenever this counter is accessed. Any wildcards in the counter names will be expanded. |
/arithmetics/multiply |
None | Returns the product calculated based on the values queried from the underlying counters (the ones specified as the parameters). | The parameter will be interpreted as a comma separated list of full performance counter names which are queried whenever this counter is accessed. Any wildcards in the counter names will be expanded. |
/arithmetics/divide |
None | Returns the result of division of the values queried from the underlying counters (the ones specified as the parameters). | The parameter will be interpreted as a comma separated list of full performance counter names which are queried whenever this counter is accessed. Any wildcards in the counter names will be expanded. |
/arithmetics/mean |
None | Returns the average value of all values queried from the underlying counters (the ones specified as the parameters). | The parameter will be interpreted as a comma separated list of full performance counter names which are queried whenever this counter is accessed. Any wildcards in the counter names will be expanded. |
/arithmetics/variance |
None | Returns the standard deviation of all values queried from the underlying counters (the ones specified as the parameters). | The parameter will be interpreted as a comma separated list of full performance counter names which are queried whenever this counter is accessed. Any wildcards in the counter names will be expanded. |
/arithmetics/median |
None | Returns the median value of all values queried from the underlying counters (the ones specified as the parameters). | The parameter will be interpreted as a comma separated list of full performance counter names which are queried whenever this counter is accessed. Any wildcards in the counter names will be expanded. |
/arithmetics/min |
None | Returns the minimum value of all values queried from the underlying counters (the ones specified as the parameters). | The parameter will be interpreted as a comma separated list of full performance counter names which are queried whenever this counter is accessed. Any wildcards in the counter names will be expanded. |
/arithmetics/max |
None | Returns the maximum value of all values queried from the underlying counters (the ones specified as the parameters). | The parameter will be interpreted as a comma separated list of full performance counter names which are queried whenever this counter is accessed. Any wildcards in the counter names will be expanded. |
/arithmetics/count |
None | Returns the count value of all values queried from the underlying counters (the ones specified as the parameters). | The parameter will be interpreted as a comma separated list of full performance counter names which are queried whenever this counter is accessed. Any wildcards in the counter names will be expanded. |
Note
The /arithmetics
counters can consume an arbitrary number of other
counters. For this reason those have to be specified as parameters (a comma
separated list of counters appended after a '@'
. For instance:
./bin/hello_world_distributed -t2 \
--hpx:print-counter=/threads{locality#0/worker-thread#*}/count/cumulative \
--hpx:print-counter=/arithmetics/add@/threads{locality#0/worker-thread#*}/count/cumulative
hello world from OS-thread 0 on locality 0
hello world from OS-thread 1 on locality 0
/threads{locality#0/worker-thread#0}/count/cumulative,1,0.515640,[s],25
/threads{locality#0/worker-thread#1}/count/cumulative,1,0.515520,[s],36
/arithmetics/add@/threads{locality#0/worker-thread#*}/count/cumulative,1,0.516445,[s],64
Since all wildcards in the parameters are expanded, this example is fully
equivalent to specifying both counters separately to /arithmetics/add
:
./bin/hello_world_distributed -t2 \
--hpx:print-counter=/threads{locality#0/worker-thread#*}/count/cumulative \
--hpx:print-counter=/arithmetics/add@\
/threads{locality#0/worker-thread#0}/count/cumulative,\
/threads{locality#0/worker-thread#1}/count/cumulative
Counter type | Counter instance formatting | Description | Parameters |
/coalescing/count/parcels |
where:
|
Returns the number of parcels handled by the message handler associated with the action which is given by the counter parameter. | The action type. This is the string which has been used while registering
the action with HPX, e.g. which has been passed as the second parameter
to the macro HPX_REGISTER_ACTION or
HPX_REGISTER_ACTION_ID . |
/coalescing/count/messages |
where:
|
Returns the number of messages generated by the message handler associated with the action which is given by the counter parameter. | The action type. This is the string which has been used while registering
the action with HPX, e.g. which has been passed as the second parameter
to the macro HPX_REGISTER_ACTION or
HPX_REGISTER_ACTION_ID . |
/coalescing/count/average-parcels-per-message |
where:
|
Returns the average number of parcels sent in a message generated by the message handler associated with the action which is given by the counter parameter. | The action type. This is the string which has been used while registering
the action with HPX, e.g. which has been passed as the second parameter
to the macro HPX_REGISTER_ACTION or
HPX_REGISTER_ACTION_ID |
/coalescing/time/average-parcel-arrival |
where:
|
Returns the average time between arriving parcels for the action which is given by the counter parameter. | The action type. This is the string which has been used while registering
the action with HPX, e.g. which has been passed as the second parameter
to the macro HPX_REGISTER_ACTION or
HPX_REGISTER_ACTION_ID |
/coalescing/time/parcel-arrival-histogram |
where:
|
Returns a histogram representing the times between arriving parcels for the action which is given by the counter parameter. This counter returns an array of values, where the first three values represent the three parameters used for the histogram followed by one value for each of the histogram buckets. The first unit of measure displayed for this counter For each bucket the counter shows a value between |
The action type and optional histogram parameters. The action type is
the string which has been used while registering the action with HPX,
e.g. which has been passed as the second parameter to the macro
The action type may be followed by a comma separated list of up-to three
numbers: the lower and upper boundaries for the collected histogram, and
the number of buckets for the histogram to generate. By default these
three numbers will be assumed to be |
Note
The performance counters related to parcel coalescing are available only if
the configuration time constant HPX_WITH_PARCEL_COALESCING
is set to
ON
(default: ON
). However, even in this case it will be available
only for those actions, which are enabled for parcel coalescing (see the
macros HPX_ACTION_USES_MESSAGE_COALESCING
and
HPX_ACTION_USES_MESSAGE_COALESCING_NOTHROW
).
[1] | A message can potentially consist of more than one parcel. |
APEX integration¶
HPX provides integration with APEX, which is a framework for application
profiling using task timers and various performance counters. It can be added as
a git
submodule by turning on the option HPX_WITH_APEX:BOOL
during
CMake configuration. TAU is an optional dependency when using APEX.
To build HPX with APEX add HPX_WITH_APEX
=ON
, and,
optionally, TAU_ROOT=$PATH_TO_TAU
to your CMake configuration. In
addition, you can override the tag used for APEX with the
HPX_WITH_APEX_TAG
option. Please see the APEX HPX documentation for detailed
instructions on using APEX with HPX.
HPX runtime and resources¶
HPX thread scheduling policies¶
The HPX runtime has five thread scheduling policies: local-priority,
static-priority, local, static and abp-priority. These policies can be specified
from the command line using the command line option --hpx:queuing
. In
order to use a particular scheduling policy, the runtime system must be built
with the appropriate scheduler flag turned on (e.g. cmake
-DHPX_THREAD_SCHEDULERS=local
, see CMake variables used to configure HPX for more
information).
Priority local scheduling policy (default policy)¶
- default or invoke using:
--hpx:queuing
local-priority-fifo
The priority local scheduling policy maintains one queue per operating system
(OS) thread. The OS thread pulls its work from this queue. By default the number
of high priority queues is equal to the number of OS threads; the number of high
priority queues can be specified on the command line using
--hpx:high-priority-threads
. High priority threads are executed by any
of the OS threads before any other work is executed. When a queue is empty work
will be taken from high priority queues first. There is one low priority queue
from which threads will be scheduled only when there is no other work.
For this scheduling policy there is an option to turn on NUMA sensitivity using
the command line option --hpx:numa-sensitive
. When NUMA sensitivity is
turned on work stealing is done from queues associated with the same NUMA domain
first, only after that work is stolen from other NUMA domains.
This scheduler is enabled at build time by default and will be available always.
This scheduler can be used with two underlying queuing policies (FIFO:
first-in-first-out, and LIFO: last-in-first-out). The default is FIFO. In order
to use the LIFO policy use the command line option --hpx:queuing
=local-priority-lifo
.
Static priority scheduling policy¶
- invoke using:
--hpx:queuing
=static-priority
(or-qs
) - flag to turn on for build:
HPX_THREAD_SCHEDULERS=all
orHPX_THREAD_SCHEDULERS=static-priority
The static scheduling policy maintains one queue per OS thread from which each OS thread pulls its tasks (user threads). Threads are distributed in a round robin fashion. There is no thread stealing in this policy.
Local scheduling policy¶
- invoke using:
--hpx:queuing
=local
(or-ql
) - flag to turn on for build:
HPX_THREAD_SCHEDULERS=all
orHPX_THREAD_SCHEDULERS=local
The local scheduling policy maintains one queue per OS thread from which each OS thread pulls its tasks (user threads).
Static scheduling policy¶
- invoke using:
--hpx:queuing
=static
- flag to turn on for build:
HPX_THREAD_SCHEDULERS=all
orHPX_THREAD_SCHEDULERS=static
The static scheduling policy maintains one queue per OS thread from which each OS thread pulls its tasks (user threads). Threads are distributed in a round robin fashion. There is no thread stealing in this policy.
Priority ABP scheduling policy¶
- invoke using:
--hpx:queuing
=abp-priority-fifo
- flag to turn on for build:
HPX_THREAD_SCHEDULERS=all
orHPX_THREAD_SCHEDULERS=abp-priority
Priority ABP policy maintains a double ended lock free queue for each OS thread.
By default the number of high priority queues is equal to the number of OS
threads; the number of high priority queues can be specified on the command line
using --hpx:high-priority-threads
. High priority threads are executed
by the first OS threads before any other work is executed. When a queue is empty
work will be taken from high priority queues first. There is one low priority
queue from which threads will be scheduled only when there is no other work. For
this scheduling policy there is an option to turn on NUMA sensitivity using the
command line option --hpx:numa-sensitive
. When NUMA sensitivity
is turned on work stealing is done from queues associated with the same NUMA
domain first, only after that work is stolen from other NUMA domains.
This scheduler can be used with two underlying queuing policies (FIFO:
first-in-first-out, and LIFO: last-in-first-out). In order to use the LIFO
policy use the command line option --hpx:queuing
=abp-priority-lifo
.
The HPX resource partitioner¶
The HPX resource partitioner lets you take the execution resources available
on a system—processing units, cores, and numa domains—and assign them to
thread pools. By default HPX creates a single thread pool name default
.
While this is good for most use cases, the resource partitioner lets you create
multiple thread pools with custom resources and options.
Creating custom thread pools is useful for cases where you have tasks which absolutely need to run without interference from other tasks. An example of this is when using MPI for distribution instead of the built-in mechanisms in HPX (useful in legacy applications). In this case one can create a thread pool containing a single thread for MPI communication. MPI tasks will then always run on the same thread, instead of potentially being stuck in a queue behind other threads.
Note that HPX thread pools are completely independent from each other in the sense that task stealing will never happen between different thread pools. However, tasks running on a particular thread pool can schedule tasks on another thread pool.
Note
It is simpler in some situations to to schedule important tasks with high priority instead of using a separate thread pool.
Using the resource partitioner¶
In order to create custom thread pools the resource partitioner needs to be set
up before HPX is initialized by creating an instance of
hpx::resource::partitioner
:
#include <hpx/hpx_init.hpp>
#include <hpx/runtime/resource/partitioner.hpp>
int hpx_main(int argc, char* argv[])
{
return hpx::finalize();
}
int main(int argc, char** argv)
{
hpx::resource::partitioner rp(argc, argv);
hpx::init();
}
Note that we have to pass argc
and argv
to the resource partitioner to
be able to parse thread binding options passed on the command line. You should
pass the same arguments to the hpx::resource::partitioner
constructor as you would to hpx::init
or hpx::start
.
Running the above code will have the same effect as not initializing it at all,
i.e. a default thread pool will be created with the type and number of threads
specified on the command line.
The resource partitioner class is the interface to add thread pools to the HPX runtime and to assign resources to the thread pools.
To add a thread pool use the
hpx::resource::partitioner::create_thread_pool
method. If you
simply want to use the default scheduler and scheduler options it is enough to
call rp.create_thread_pool("my-thread-pool")
.
Then, to add resources to the thread pool you can use the
hpx::resource::partitioner::add_resource
method. The resource
partitioner exposes the hardware topology retrieved using Portable Hardware Locality (HWLOC) and lets you
iterate through the topology to add the wanted processing units to the thread
pool. Below is an example of adding all processing units from the first NUMA
domain to a custom thread pool, unless there is only one NUMA domain in which
case we leave the first processing unit for the default thread pool:
#include <hpx/hpx_init.hpp>
#include <hpx/runtime/resource/partitioner.hpp>
#include <iostream>
int hpx_main(int argc, char* argv[])
{
return hpx::finalize();
}
int main(int argc, char* argv[])
{
hpx::resource::partitioner rp(argc, argv);
rp.create_thread_pool("my-thread-pool");
bool one_numa_domain = rp.numa_domains().size() == 1;
bool skipped_first_pu = false;
hpx::resource::numa_domain const& d = rp.numa_domains()[0];
for (const hpx::resource::core& c : d.cores())
{
for (const hpx::resource::pu& p : c.pus())
{
if (one_numa_domain && !skipped_first_pu)
{
skipped_first_pu = true;
continue;
}
rp.add_resource(p, "my-thread-pool");
}
}
hpx::init();
}
Note
Whatever processing units not assigned to a thread pool by the time
hpx::init
is called will be added to the default thread pool. It
is also possible to explicitly add processing units to the default thread
pool, and to create the default thread pool manually (in order to e.g. set
the scheduler type).
Tip
The command line option --hpx:print-bind
is useful for checking
that the thread pools have been set up the way you expect.
Advanced usage¶
It is possible to customize the built in schedulers by passing scheduler options
to hpx::resource::partitioner::create_thread_pool
. It is also possible
to create and use custom schedulers.
Note
It is not recommended to create your own scheduler. The HPX developers use
this to experiment with new scheduler designs before making them available to
users via the standard mechanisms of choosing a scheduler (command line
options). If you would like to experiment with a custom scheduler the
resource partitioner example shared_priority_queue_scheduler.cpp
contains
a fully implemented scheduler with logging etc. to make exploration easier.
To choose a scheduler and custom mode for a thread pool, pass additional options when creating the thread pool like this:
rp.create_thread_pool("my-thread-pool",
hpx::resource::policies::local_priority_lifo,
hpx::policies::scheduler_mode(
hpx::policies::scheduler_mode::default |
hpx::policies::scheduler_mode::enable_elasticity));
The available schedulers are documented here:
hpx::resource::scheduling_policy
, and the available scheduler modes
here: hpx::threads::policies::scheduler_mode
. Also see the examples
folder for examples of advanced resource partitioner usage:
simple_resource_partitioner.cpp
and
oversubscribing_resource_partitioner.cpp
.
Miscellaneous¶
Error handling¶
Like in any other asynchronous invocation scheme it is important to be able to handle error conditions occurring while the asynchronous (and possibly remote) operation is executed. In HPX all error handling is based on standard C++ exception handling. Any exception thrown during the execution of an asynchronous operation will be transferred back to the original invocation locality, where it is rethrown during synchronization with the calling thread.
The source code for this example can be found here:
error_handling.cpp
.
Working with exceptions¶
For the following description we assume that the function raise_exception()
is executed by invoking the plain action raise_exception_type
.
void raise_exception()
{
HPX_THROW_EXCEPTION(hpx::no_success, "raise_exception", "simulated error");
}
HPX_PLAIN_ACTION(raise_exception, raise_exception_action);
The exception is thrown using the macro HPX_THROW_EXCEPTION
. The type
of the thrown exception is hpx::exception
. This associates
additional diagnostic information with the exception, such as file name and line
number, locality id and thread id, and stack backtrace from the point
where the exception was thrown.
Any exception thrown during the execution of an action is transferred back to
the (asynchronous) invocation site. It will be rethrown in this context when the
calling thread tries to wait for the result of the action by invoking either
future<>::get()
or the synchronous action invocation wrapper as shown here:
hpx::cout << "Error reporting using exceptions\n";
try {
// invoke raise_exception() which throws an exception
raise_exception_action do_it;
do_it(hpx::find_here());
}
catch (hpx::exception const& e) {
// Print just the essential error information.
hpx::cout << "caught exception: " << e.what() << "\n\n";
// Print all of the available diagnostic information as stored with
// the exception.
hpx::cout << "diagnostic information:"
<< hpx::diagnostic_information(e) << "\n";
}
hpx::cout << hpx::flush;
Note
The exception is transferred back to the invocation site even if it is executed on a different locality.
Additionally, this example demonstrates how an exception thrown by an (possibly
remote) action can be handled. It shows the use of
hpx::diagnostic_information
which retrieves all available diagnostic
information from the exception as a formatted string. This includes, for
instance, the name of the source file and line number, the sequence number of
the OS-thread and the HPX-thread id, the locality id and the stack
backtrace of the point where the original exception was thrown.
Under certain circumstances it is desirable to output only some of the diagnostics, or to output those using different formatting. For this case, HPX exposes a set of lower level functions as demonstrated in the following code snippet:
hpx::cout << "Detailed error reporting using exceptions\n";
try {
// Invoke raise_exception() which throws an exception.
raise_exception_action do_it;
do_it(hpx::find_here());
}
catch (hpx::exception const& e) {
// Print the elements of the diagnostic information separately.
hpx::cout << "{what}: " << hpx::get_error_what(e) << "\n";
hpx::cout << "{locality-id}: " << hpx::get_error_locality_id(e) << "\n";
hpx::cout << "{hostname}: " << hpx::get_error_host_name(e) << "\n";
hpx::cout << "{pid}: " << hpx::get_error_process_id(e) << "\n";
hpx::cout << "{function}: " << hpx::get_error_function_name(e) << "\n";
hpx::cout << "{file}: " << hpx::get_error_file_name(e) << "\n";
hpx::cout << "{line}: " << hpx::get_error_line_number(e) << "\n";
hpx::cout << "{os-thread}: " << hpx::get_error_os_thread(e) << "\n";
hpx::cout << "{thread-id}: " << std::hex << hpx::get_error_thread_id(e)
<< "\n";
hpx::cout << "{thread-description}: "
<< hpx::get_error_thread_description(e) << "\n";
hpx::cout << "{state}: " << std::hex << hpx::get_error_state(e)
<< "\n";
hpx::cout << "{stack-trace}: " << hpx::get_error_backtrace(e) << "\n";
hpx::cout << "{env}: " << hpx::get_error_env(e) << "\n";
}
hpx::cout << hpx::flush;
Working with error codes¶
Most of the API functions exposed by HPX can be invoked in two different
modes. By default those will throw an exception on error as described above.
However, sometimes it is desirable not to throw an exception in case of an error
condition. In this case an object instance of the hpx::error_code
type can be passed as the last argument to the API function. In case of an error
the error condition will be returned in that hpx::error_code
instance. The following example demonstrates extracting the full diagnostic
information without exception handling:
hpx::cout << "Error reporting using error code\n";
// Create a new error_code instance.
hpx::error_code ec;
// If an instance of an error_code is passed as the last argument while
// invoking the action, the function will not throw in case of an error
// but store the error information in this error_code instance instead.
raise_exception_action do_it;
do_it(hpx::find_here(), ec);
if (ec) {
// Print just the essential error information.
hpx::cout << "returned error: " << ec.get_message() << "\n";
// Print all of the available diagnostic information as stored with
// the exception.
hpx::cout << "diagnostic information:"
<< hpx::diagnostic_information(ec) << "\n";
}
hpx::cout << hpx::flush;
Note
The error information is transferred back to the invocation site even if it is executed on a different locality.
This example show how an error can be handled without having to resolve to
exceptions and that the returned hpx::error_code
instance can be
used in a very similar way as the hpx::exception
type above. Simply
pass it to the hpx::diagnostic_information
which retrieves all
available diagnostic information from the error code instance as a formatted
string.
As for handling exceptions, when working with error codes, under certain circumstances it is desirable to output only some of the diagnostics, or to output those using different formatting. For this case, HPX exposes a set of lower level functions usable with error codes as demonstrated in the following code snippet:
hpx::cout << "Detailed error reporting using error code\n";
// Create a new error_code instance.
hpx::error_code ec;
// If an instance of an error_code is passed as the last argument while
// invoking the action, the function will not throw in case of an error
// but store the error information in this error_code instance instead.
raise_exception_action do_it;
do_it(hpx::find_here(), ec);
if (ec) {
// Print the elements of the diagnostic information separately.
hpx::cout << "{what}: " << hpx::get_error_what(ec) << "\n";
hpx::cout << "{locality-id}: " << hpx::get_error_locality_id(ec) << "\n";
hpx::cout << "{hostname}: " << hpx::get_error_host_name(ec) << "\n";
hpx::cout << "{pid}: " << hpx::get_error_process_id(ec) << "\n";
hpx::cout << "{function}: " << hpx::get_error_function_name(ec)
<< "\n";
hpx::cout << "{file}: " << hpx::get_error_file_name(ec) << "\n";
hpx::cout << "{line}: " << hpx::get_error_line_number(ec) << "\n";
hpx::cout << "{os-thread}: " << hpx::get_error_os_thread(ec) << "\n";
hpx::cout << "{thread-id}: " << std::hex
<< hpx::get_error_thread_id(ec) << "\n";
hpx::cout << "{thread-description}: "
<< hpx::get_error_thread_description(ec) << "\n\n";
hpx::cout << "{state}: " << std::hex << hpx::get_error_state(ec)
<< "\n";
hpx::cout << "{stack-trace}: " << hpx::get_error_backtrace(ec) << "\n";
hpx::cout << "{env}: " << hpx::get_error_env(ec) << "\n";
}
hpx::cout << hpx::flush;
For more information please refer to the documentation of
hpx::get_error_what
, hpx::get_error_locality_id
,
hpx::get_error_host_name
, hpx::get_error_process_id
,
hpx::get_error_function_name
, hpx::get_error_file_name
,
hpx::get_error_line_number
, hpx::get_error_os_thread
,
hpx::get_error_thread_id
,
hpx::get_error_thread_description
,
hpx::get_error_backtrace
, hpx::get_error_env
, and
hpx::get_error_state
.
Lightweight error codes¶
Sometimes it is not desirable to collect all the ambient information about the error at the point where it happened as this might impose too much overhead for simple scenarios. In this case, HPX provides a lightweight error code facility which will hold the error code only. The following snippet demonstrates its use:
hpx::cout << "Error reporting using an lightweight error code\n";
// Create a new error_code instance.
hpx::error_code ec(hpx::lightweight);
// If an instance of an error_code is passed as the last argument while
// invoking the action, the function will not throw in case of an error
// but store the error information in this error_code instance instead.
raise_exception_action do_it;
do_it(hpx::find_here(), ec);
if (ec) {
// Print just the essential error information.
hpx::cout << "returned error: " << ec.get_message() << "\n";
// Print all of the available diagnostic information as stored with
// the exception.
hpx::cout << "error code:" << ec.value() << "\n";
}
hpx::cout << hpx::flush;
All functions which retrieve other diagnostic elements from the
hpx::error_code
will fail if called with a lightweight error_code
instance.
Utilities in HPX¶
In order to ease the burden of programming in HPX we have provided several utilities to users. The following section documents those facilies.
Checkpoint¶
A common need of users is to periodically backup an application. This practice
provides resiliency and potential restart points in code. We have developed the
concept of a checkpoint
to support this use case.
Found in hpx/util/checkpoint.hpp
, checkpoint
s are defined as objects
which hold a serialized version of an object or set of objects at a particular
moment in time. This representation can be stored in memory for later use or it
can be written to disk for storage and/or recovery at a later point. In order to
create and fill this object with data we use a function called
save_checkpoint
. In code the function looks like this:
hpx::future<hpx::util::checkpoint> hpx::util::save_checkpoint(a, b, c, ...);
save_checkpoint
takes arbitrary data containers such as int, double, float,
vector, and future and serializes them into a newly created checkpoint
object. This function returns a future
to a checkpoint
containing the
data. Let us look a simple use case below:
using hpx::util::checkpoint;
using hpx::util::save_checkpoint;
std::vector<int> vec{1,2,3,4,5};
hpx::future<checkpoint> save_checkpoint(vec);
Once the future is ready the checkpoint object will contain the vector
vec
and its five elements.
It is also possible to modify the launch policy used by save_checkpoint
.
This is accomplished by passing a launch policy as the first argument. It is
important to note that passing hpx::launch::sync
will cause
save_checkpoint
to return a checkpoint
instead of a future
to a
checkpoint
. All other policies passed to save_checkpoint
will return a
future
to a checkpoint
.
Sometimes checkpoint
s must be declared before they are used.
save_checkpoint
allows users to move pre-created checkpoint
s into the
function as long as they are the first container passing into the function (In
the case where a launch policy is used, the checkpoint
will immediately
follow the launch policy). An example of these features can be found below:
char character = 'd';
int integer = 10;
float flt = 10.01f;
bool boolean = true;
std::string str = "I am a string of characters";
std::vector<char> vec(str.begin(), str.end());
checkpoint archive;
// Test 1
// test basic functionality
hpx::shared_future<checkpoint> f_archive = save_checkpoint(
std::move(archive), character, integer, flt, boolean, str, vec);
Now that we can create checkpoint
s we now must be able to restore the
objects they contain into memory. This is accomplished by the function
restore_checkpoint
. This function takes a checkpoint
and fills its data
into the containers it is provided. It is important to remember that the
containers must be ordered in the same way they were placed into the
checkpoint
. For clarity see the example below:
char character2;
int integer2;
float flt2;
bool boolean2;
std::string str2;
std::vector<char> vec2;
restore_checkpoint(
f_archive.get(), character2, integer2, flt2, boolean2, str2, vec2);
The core utility of checkpoint
is in its ability to make certain data
persistent. Often this means that the data is needed to be stored in an object,
such as a file, for later use. For these cases we have provided two solutions:
stream operator overloads and access iterators.
We have created the two stream overloads
operator<<
and operator>>
to stream data
out of and into checkpoint
. You can see an
example of the overloads in use below:
double a9 = 1.0, b9 = 1.1, c9 = 1.2;
std::ofstream test_file_9("test_file_9.txt");
hpx::future<checkpoint> f_9 = save_checkpoint(a9, b9, c9);
test_file_9 << f_9.get();
test_file_9.close();
double a9_1, b9_1, c9_1;
std::ifstream test_file_9_1("test_file_9.txt");
checkpoint archive9;
test_file_9_1 >> archive9;
restore_checkpoint(archive9, a9_1, b9_1, c9_1);
This is the primary way to move data into and out of a checkpoint
. It is
important to note, however, that users should be cautious when using a stream
operator to load data an another function to remove it (or vice versa). Both
operator<<
and operator>>
rely on a .write()
and a .read()
function respectively. In order to know how much data to read from the
std::istream
, the operator<<
will write the size of the checkpoint
before writing the checkpoint
data. Correspondingly, the operator>>
will
read the size of the stored data before reading the data into new instance of
checkpoint
. As long as the user employs the operator<<
and
operator>>
to stream the data this detail can be ignored.
Important
Be careful when mixing operator<<
and operator>>
with other
facilities to read and write to a checkpoint
. operator<<
writes and
extra variable and operator>>
reads this variable back separately. Used
together the user will not encounter any issues and can safely ignore this
detail.
Users may also move the data into and out of a checkpoint
using the exposed
.begin()
and .end()
iterators. An example of this use case is
illustrated below.
std::ofstream test_file_7("checkpoint_test_file.txt");
std::vector<float> vec7{1.02f, 1.03f, 1.04f, 1.05f};
hpx::future<checkpoint> fut_7 = save_checkpoint(vec7);
checkpoint archive7 = fut_7.get();
std::copy(archive7.begin() // Write data to ofstream
, archive7.end() // ie. the file
, std::ostream_iterator<char>(test_file_7));
test_file_7.close();
std::vector<float> vec7_1;
std::vector<char> char_vec;
std::ifstream test_file_7_1("checkpoint_test_file.txt");
if (test_file_7_1)
{
test_file_7_1.seekg(0, test_file_7_1.end);
int length = test_file_7_1.tellg();
test_file_7_1.seekg(0, test_file_7_1.beg);
char_vec.resize(length);
test_file_7_1.read(char_vec.data(), length);
}
checkpoint archive7_1(std::move(char_vec)); // Write data to checkpoint
restore_checkpoint(archive7_1, vec7_1);
The HPX I/O-streams component¶
The HPX I/O-streams subsystem extends the standard C++ output streams
std::cout
and std::cerr
to work in the distributed setting of an HPX
application. All of the output streamed to``hpx::cout``will be dispatched to
std::cout
on the console locality. Likewise, all output generated
from hpx::cerr
will be dispatched to std::cerr
on the console
locality.
Note
All existing standard manipulators can be used in conjunction with
hpx::cout
and hpx::cerr
Historically, HPX also defines
hpx::endl
and hpx::flush
but those are just aliases for the
corresponding standard manipulators.
In order to use either hpx::cout
or hpx::cerr
application codes need to
#include <hpx/include/iostreams.hpp>
. For an example, please see the
simplest possible ‘Hello world’ program as included as an example with HPX:
// Copyright (c) 2007-2012 Hartmut Kaiser
//
// Distributed under the Boost Software License, Version 1.0. (See accompanying
// file LICENSE_1_0.txt or copy at http://www.boost.org/LICENSE_1_0.txt)
///////////////////////////////////////////////////////////////////////////////
// The purpose of this example is to execute a HPX-thread printing
// "Hello World!" once. That's all.
//[hello_world_1_getting_started
// Including 'hpx/hpx_main.hpp' instead of the usual 'hpx/hpx_init.hpp' enables
// to use the plain C-main below as the direct main HPX entry point.
#include <hpx/hpx_main.hpp>
#include <hpx/include/iostreams.hpp>
int main()
{
// Say hello to the world!
hpx::cout << "Hello World!\n" << hpx::flush;
return 0;
}
//]
Additionally those applications need to link with the iostreams component. When
using cmake this can be achieved by using the COMPONENT_DEPENDENCIES
parameter, for instance:
include(HPX_AddExecutable)
add_hpx_executable(
hello_world
SOURCES hello_world.cpp
COMPONENT_DEPENDENCIES iostreams
)
Note
The hpx::cout
and hpx::cerr
streams buffer all output locally until a
std::endl
or std::flush
is encountered. That means that no output
will appear on the console as long as either of those is explicitly used.
Additional material¶
- 2-day workshop held at CSCS in 2016
- Tutorials repository
- STE||AR Group blog posts
Overview¶
HPX is organized into different sub-libraries. Those libraries can be seen as
independent modules, with clear dependencies and no cycles. As an end-user, the
use of these modules is completely transparent. If you use e.g.
add_hpx_executable
to create a target in your project you will automatically
get all modules as dependencies. See All modules for a list of the
available modules.
All modules¶
Example module¶
This is an example module used to explain the structure of an HPX module.
The tool create_library_skeleton.py can be used to generate a basic skeleton. The structure of this skeleton is as follows:
<lib_name>/
README.rst
CMakeLists.txt
cmake
docs/
index.rst
examples/
CMakeLists.txt
include/
hpx/
<lib_name>
src/
CMakeLists.txt
tests/
CMakeLists.txt
unit/
CMakeLists.txt
regressions/
CMakeLists.txt
performance/
CMakeLists.txt
A README.rst
should be always included which explains the basic purpose of
the library and a link to the generated documentation.
The include
directory should contain only headers that other libraries need.
For each of those headers, an automatic header test to check for self
containment will be generated. Private headers should be placed under the
src
directory. This allows for clear seperation. The cmake
subdirectory
may include additional CMake scripts needed to generate the respective build
configurations.
Documentation is placed in the docs
folder. A empty skeleton for the index
is created, which is picked up by the main build system and will be part of the
generated documentation. Each header inside the include
directory will
automatically be processed by Doxygen and included into the documentation. If a
header should be excluded from the API reference, a comment //
sphinx:undocumented
needs to be added.
In order to consume any library defined here, all you have to do is use
target_link_libraries
to get the dependencies. This of course requires that
the library to link against specified the appropriate target include directories
and libraries.
preprocessor¶
This library contains useful preprocessor macros:
API reference¶
Main HPX library reference¶
-
template<typename
Action
>
structasync_result
¶ - #include <colocating_distribution_policy.hpp>
- Note
- This function is part of the invocation policy implemented by this class
-
template<typename
Action
>
structasync_result
¶ - #include <default_distribution_policy.hpp>
- Note
- This function is part of the invocation policy implemented by this class
-
struct
auto_chunk_size
¶ - #include <auto_chunk_size.hpp>
Loop iterations are divided into pieces and then assigned to threads. The number of loop iterations combined is determined based on measurements of how long the execution of 1% of the overall number of iterations takes. This executor parameters type makes sure that as many loop iterations are combined as necessary to run for the amount of time specified.
Public Functions
-
auto_chunk_size
()¶ Construct an auto_chunk_size executor parameters object
- Note
- Default constructed auto_chunk_size executor parameter types will use 80 microseconds as the minimal time for which any of the scheduled chunks should run.
-
auto_chunk_size
(hpx::util::steady_duration const &rel_time)¶ Construct an auto_chunk_size executor parameters object
- Parameters
rel_time
: [in] The time duration to use as the minimum to decide how many loop iterations should be combined.
-
-
class
barrier
¶ - #include <barrier.hpp>
The barrier is an implementation performing a barrier over a number of participating threads. The different threads don’t have to be on the same locality. This barrier can be invoked in a distributed application.
For a local only barrier
- See
- hpx::lcos::local::barrier.
Public Functions
-
barrier
(std::string const &base_name)¶ Creates a barrier, rank is locality id, size is number of localities
A barrier
base_name is created. It expects that hpx::get_num_localities() participate and the local rank is hpx::get_locality_id().- Parameters
base_name
: The name of the barrier
-
barrier
(std::string const &base_name, std::size_t num)¶ Creates a barrier with a given size, rank is locality id
A barrier
base_name is created. It expects that num participate and the local rank is hpx::get_locality_id().- Parameters
base_name
: The name of the barriernum
: The number of participating threads
-
barrier
(std::string const &base_name, std::size_t num, std::size_t rank)¶ Creates a barrier with a given size and rank
A barrier
base_name is created. It expects that num participate and the local rank is rank.- Parameters
base_name
: The name of the barriernum
: The number of participating threadsrank
: The rank of the calling site for this invocation
-
barrier
(std::string const &base_name, std::vector<std::size_t> const &ranks, std::size_t rank)¶ Creates a barrier with a vector of ranks
A barrier
base_name is created. It expects that ranks.size() and the local rank is rank (must be contained in ranks).- Parameters
base_name
: The name of the barrierranks
: Gives a list of participating ranks (this could be derived from a list of locality idsrank
: The rank of the calling site for this invocation
-
void
wait
()¶ Wait until each participant entered the barrier. Must be called by all participants
- Return
- This function returns once all participants have entered the barrier (have called wait).
Public Static Functions
-
static void
synchronize
()¶ Perform a global synchronization using the default global barrier The barrier is created once at startup and can be reused throughout the lifetime of an HPX application.
- Note
- This function currently does not support dynamic connection and disconnection of localities.
-
struct
binpacking_distribution_policy
¶ - #include <binpacking_distribution_policy.hpp>
This class specifies the parameters for a binpacking distribution policy to use for creating a given number of items on a given set of localities. The binpacking policy will distribute the new objects in a way such that each of the localities will equalize the number of overall objects of this type based on a given criteria (by default this criteria is the overall number of objects of this type).
Public Functions
-
binpacking_distribution_policy
()¶ Default-construct a new instance of a binpacking_distribution_policy. This policy will represent one locality (the local locality).
-
binpacking_distribution_policy
operator()
(std::vector<id_type> const &locs, char const *perf_counter_name = default_binpacking_counter_name) const¶ Create a new default_distribution policy representing the given set of localities.
- Parameters
locs
: [in] The list of localities the new instance should representperf_counter_name
: [in] The name of the performance counter which should be used as the distribution criteria (by default the overall number of existing instances of the given component type will be used).
-
binpacking_distribution_policy
operator()
(std::vector<id_type> &&locs, char const *perf_counter_name = default_binpacking_counter_name) const¶ Create a new default_distribution policy representing the given set of localities.
- Parameters
locs
: [in] The list of localities the new instance should representperf_counter_name
: [in] The name of the performance counter which should be used as the distribution criteria (by default the overall number of existing instances of the given component type will be used).
-
binpacking_distribution_policy
operator()
(id_type const &loc, char const *perf_counter_name = default_binpacking_counter_name) const¶ Create a new default_distribution policy representing the given locality
- Parameters
loc
: [in] The locality the new instance should representperf_counter_name
: [in] The name of the performance counter which should be used as the distribution criteria (by default the overall number of existing instances of the given component type will be used).
-
template<typename
Component
, typename ...Ts
>
hpx::future<hpx::id_type>create
(Ts&&... vs) const¶ Create one object on one of the localities associated by this policy instance
- Return
- A future holding the global address which represents the newly created object
- Parameters
vs
: [in] The arguments which will be forwarded to the constructor of the new object.
-
template<typename
Component
, typename ...Ts
>
hpx::future<std::vector<bulk_locality_result>>bulk_create
(std::size_t count, Ts&&... vs) const¶ Create multiple objects on the localities associated by this policy instance
- Return
- A future holding the list of global addresses which represent the newly created objects
- Parameters
count
: [in] The number of objects to createvs
: [in] The arguments which will be forwarded to the constructors of the new objects.
-
std::string const &
get_counter_name
() const¶ Returns the name of the performance counter associated with this policy instance.
-
std::size_t
get_num_localities
() const¶ Returns the number of associated localities for this distribution policy
- Note
- This function is part of the creation policy implemented by this class
-
-
class
checkpoint
¶ - #include <checkpoint.hpp>
Checkpoint Object
Checkpoint is the container object which is produced by save_checkpoint and is consumed by a restore_checkpoint. A checkpoint may be moved into the save_checkpoint object to write the byte stream to the pre-created checkpoint object.
Public Types
-
using
const_iterator
= std::vector::const_iterator¶
Public Functions
-
checkpoint
()¶
-
checkpoint
(checkpoint const &c)¶
-
checkpoint
(checkpoint &&c)¶
-
~checkpoint
()¶
-
checkpoint
(std::vector<char> const &vec)¶
-
checkpoint
(std::vector<char> &&vec)¶
-
checkpoint &
operator=
(checkpoint const &c)¶
-
checkpoint &
operator=
(checkpoint &&c)¶
-
bool
operator==
(checkpoint const &c) const¶
-
bool
operator!=
(checkpoint const &c) const¶
-
const_iterator
begin
() const¶
-
const_iterator
end
() const¶
-
size_t
size
() const¶
Private Functions
Private Members
-
std::vector<char>
data
¶
Friends
-
friend
hpx::util::checkpoint::hpx::serialization::access
-
std::ostream &
operator<<
(std::ostream &ost, checkpoint const &ckp)¶ Operator<< Overload
This overload is the main way to write data from a checkpoint to an object such as a file. Inside the function, the size of the checkpoint will be written to the stream before the checkpoint’s data. The operator>> overload uses this to read the correct number of bytes. Be mindful of this additional write and read when you use different facilities to write out or read in data to a checkpoint!
- Parameters
ost
: Output stream to write to.ckp
: Checkpoint to copy from.
- Return
- Operator<< returns the ostream object.
-
std::istream &
operator>>
(std::istream &ist, checkpoint &ckp)¶ Operator>> Overload
This overload is the main way to read in data from an object such as a file to a checkpoint. It is important to note that inside the function, the first variable to be read is the size of the checkpoint. This size variable is written to the stream before the checkpoint’s data in the operator<< overload. Be mindful of this additional read and write when you use different facilities to read in or write out data from a checkpoint!
- Parameters
ist
: Input stream to write from.ckp
: Checkpoint to write to.
- Return
- Operator>> returns the ostream object.
-
template<typename
T
, typename ...Ts
>
voidrestore_checkpoint
(checkpoint const &c, T &t, Ts&... ts)¶ Resurrect
Restore_checkpoint takes a checkpoint object as a first argument and the containers which will be filled from the byte stream (in the same order as they were placed in save_checkpoint).
- Return
- Restore_checkpoint returns void.
- Template Parameters
T
: A container to restore.Ts
: Other containers to restore. Containers must be in the same order that they were inserted into the checkpoint.
- Parameters
c
: The checkpoint to restore.t
: A container to restore.ts
: Other containers to restore Containers must be in the same order that they were inserted into the checkpoint.
-
using
-
struct
colocating_distribution_policy
¶ - #include <colocating_distribution_policy.hpp>
This class specifies the parameters for a distribution policy to use for creating a given number of items on the locality where a given object is currently placed.
Public Functions
-
colocating_distribution_policy
()¶ Default-construct a new instance of a colocating_distribution_policy. This policy will represent the local locality.
-
colocating_distribution_policy
operator()
(id_type const &id) const¶ Create a new colocating_distribution_policy representing the locality where the given object os current located
- Parameters
id
: [in] The global address of the object with which the new instances should be colocated on
-
template<typename
Client
, typenameStub
>
colocating_distribution_policyoperator()
(client_base<Client, Stub> const &client) const¶ Create a new colocating_distribution_policy representing the locality where the given object os current located
- Parameters
client
: [in] The client side representation of the object with which the new instances should be colocated on
-
template<typename
Component
, typename ...Ts
>
hpx::future<hpx::id_type>create
(Ts&&... vs) const¶ Create one object on the locality of the object this distribution policy instance is associated with
- Note
- This function is part of the placement policy implemented by this class
- Return
- A future holding the global address which represents the newly created object
- Parameters
vs
: [in] The arguments which will be forwarded to the constructor of the new object.
-
template<typename
Component
, typename ...Ts
>
hpx::future<std::vector<bulk_locality_result>>bulk_create
(std::size_t count, Ts&&... vs) const¶ Create multiple objects colocated with the object represented by this policy instance
- Note
- This function is part of the placement policy implemented by this class
- Return
- A future holding the list of global addresses which represent the newly created objects
- Parameters
count
: [in] The number of objects to createvs
: [in] The arguments which will be forwarded to the constructors of the new objects.
-
template<typename
Action
, typename ...Ts
>
async_result<Action>::typeasync
(launch policy, Ts&&... vs) const¶
-
template<typename
Action
, typenameCallback
, typename ...Ts
>
async_result<Action>::typeasync_cb
(launch policy, Callback &&cb, Ts&&... vs) const¶ - Note
- This function is part of the invocation policy implemented by this class
-
template<typename
Action
, typenameContinuation
, typename ...Ts
>
boolapply
(Continuation &&c, threads::thread_priority priority, Ts&&... vs) const¶ - Note
- This function is part of the invocation policy implemented by this class
-
template<typename
Action
, typename ...Ts
>
boolapply
(threads::thread_priority priority, Ts&&... vs) const¶
-
template<typename
Action
, typenameContinuation
, typenameCallback
, typename ...Ts
>
boolapply_cb
(Continuation &&c, threads::thread_priority priority, Callback &&cb, Ts&&... vs) const¶ - Note
- This function is part of the invocation policy implemented by this class
-
template<typename
Action
, typenameCallback
, typename ...Ts
>
boolapply_cb
(threads::thread_priority priority, Callback &&cb, Ts&&... vs) const¶
-
std::size_t
get_num_localities
() const¶ Returns the number of associated localities for this distribution policy
- Note
- This function is part of the creation policy implemented by this class
-
-
class
core
¶ - #include <partitioner.hpp>
Public Functions
-
core
(std::size_t id = invalid_core_id, numa_domain *domain = nullptr)¶
-
std::size_t
id
() const¶
Private Static Attributes
-
const std::size_t
invalid_core_id
= std::size_t(-1)¶
Friends
-
friend
hpx::resource::core::pu
-
friend
hpx::resource::core::numa_domain
-
-
struct
default_distribution_policy
¶ - #include <default_distribution_policy.hpp>
This class specifies the parameters for a simple distribution policy to use for creating (and evenly distributing) a given number of items on a given set of localities.
Public Functions
-
default_distribution_policy
()¶ Default-construct a new instance of a default_distribution_policy. This policy will represent one locality (the local locality).
-
default_distribution_policy
operator()
(std::vector<id_type> const &locs) const¶ Create a new default_distribution policy representing the given set of localities.
- Parameters
locs
: [in] The list of localities the new instance should represent
-
default_distribution_policy
operator()
(std::vector<id_type> &&locs) const¶ Create a new default_distribution policy representing the given set of localities.
- Parameters
locs
: [in] The list of localities the new instance should represent
-
default_distribution_policy
operator()
(id_type const &loc) const¶ Create a new default_distribution policy representing the given locality
- Parameters
loc
: [in] The locality the new instance should represent
-
template<typename
Component
, typename ...Ts
>
hpx::future<hpx::id_type>create
(Ts&&... vs) const¶ Create one object on one of the localities associated by this policy instance
- Note
- This function is part of the placement policy implemented by this class
- Return
- A future holding the global address which represents the newly created object
- Parameters
vs
: [in] The arguments which will be forwarded to the constructor of the new object.
-
template<typename
Component
, typename ...Ts
>
hpx::future<std::vector<bulk_locality_result>>bulk_create
(std::size_t count, Ts&&... vs) const¶ Create multiple objects on the localities associated by this policy instance
- Note
- This function is part of the placement policy implemented by this class
- Return
- A future holding the list of global addresses which represent the newly created objects
- Parameters
count
: [in] The number of objects to createvs
: [in] The arguments which will be forwarded to the constructors of the new objects.
-
template<typename
Action
, typename ...Ts
>
async_result<Action>::typeasync
(launch policy, Ts&&... vs) const¶
-
template<typename
Action
, typenameCallback
, typename ...Ts
>
async_result<Action>::typeasync_cb
(launch policy, Callback &&cb, Ts&&... vs) const¶ - Note
- This function is part of the invocation policy implemented by this class
-
template<typename
Action
, typenameContinuation
, typename ...Ts
>
boolapply
(Continuation &&c, threads::thread_priority priority, Ts&&... vs) const¶ - Note
- This function is part of the invocation policy implemented by this class
-
template<typename
Action
, typename ...Ts
>
boolapply
(threads::thread_priority priority, Ts&&... vs) const¶
-
template<typename
Action
, typenameContinuation
, typenameCallback
, typename ...Ts
>
boolapply_cb
(Continuation &&c, threads::thread_priority priority, Callback &&cb, Ts&&... vs) const¶ - Note
- This function is part of the invocation policy implemented by this class
-
template<typename
Action
, typenameCallback
, typename ...Ts
>
boolapply_cb
(threads::thread_priority priority, Callback &&cb, Ts&&... vs) const¶
-
std::size_t
get_num_localities
() const¶ Returns the number of associated localities for this distribution policy
- Note
- This function is part of the creation policy implemented by this class
-
-
struct
dynamic_chunk_size
¶ - #include <dynamic_chunk_size.hpp>
Loop iterations are divided into pieces of size chunk_size and then dynamically scheduled among the threads; when a thread finishes one chunk, it is dynamically assigned another If chunk_size is not specified, the default chunk size is 1.
- Note
- This executor parameters type is equivalent to OpenMP’s DYNAMIC scheduling directive.
Public Functions
-
dynamic_chunk_size
(std::size_t chunk_size = 1)¶ Construct a dynamic_chunk_size executor parameters object
- Parameters
chunk_size
: [in] The optional chunk size to use as the number of loop iterations to schedule together. The default chunk size is 1.
-
class
error_code
: public error_code¶ - #include <error_code.hpp>
A hpx::error_code represents an arbitrary error condition.
The class hpx::error_code describes an object used to hold error code values, such as those originating from the operating system or other low-level application program interfaces.
- Note
- Class hpx::error_code is an adjunct to error reporting by exception
Public Functions
-
error_code
(throwmode mode = plain)¶ Construct an object of type error_code.
- Parameters
mode
: The parametermode
specifies whether the constructed hpx::error_code belongs to the error category hpx_category (if mode is plain, this is the default) or to the category hpx_category_rethrow (if mode is rethrow).
- Exceptions
nothing
:
-
error_code
(error e, throwmode mode = plain)¶ Construct an object of type error_code.
- Parameters
e
: The parametere
holds the hpx::error code the new exception should encapsulate.mode
: The parametermode
specifies whether the constructed hpx::error_code belongs to the error category hpx_category (if mode is plain, this is the default) or to the category hpx_category_rethrow (if mode is rethrow).
- Exceptions
nothing
:
-
error_code
(error e, char const *func, char const *file, long line, throwmode mode = plain)¶ Construct an object of type error_code.
- Parameters
e
: The parametere
holds the hpx::error code the new exception should encapsulate.func
: The name of the function where the error was raised.file
: The file name of the code where the error was raised.line
: The line number of the code line where the error was raised.mode
: The parametermode
specifies whether the constructed hpx::error_code belongs to the error category hpx_category (if mode is plain, this is the default) or to the category hpx_category_rethrow (if mode is rethrow).
- Exceptions
nothing
:
-
error_code
(error e, char const *msg, throwmode mode = plain)¶ Construct an object of type error_code.
- Parameters
e
: The parametere
holds the hpx::error code the new exception should encapsulate.msg
: The parametermsg
holds the error message the new exception should encapsulate.mode
: The parametermode
specifies whether the constructed hpx::error_code belongs to the error category hpx_category (if mode is plain, this is the default) or to the category hpx_category_rethrow (if mode is rethrow).
- Exceptions
std::bad_alloc
: (if allocation of a copy of the passed string fails).
-
error_code
(error e, char const *msg, char const *func, char const *file, long line, throwmode mode = plain)¶ Construct an object of type error_code.
- Parameters
e
: The parametere
holds the hpx::error code the new exception should encapsulate.msg
: The parametermsg
holds the error message the new exception should encapsulate.func
: The name of the function where the error was raised.file
: The file name of the code where the error was raised.line
: The line number of the code line where the error was raised.mode
: The parametermode
specifies whether the constructed hpx::error_code belongs to the error category hpx_category (if mode is plain, this is the default) or to the category hpx_category_rethrow (if mode is rethrow).
- Exceptions
std::bad_alloc
: (if allocation of a copy of the passed string fails).
-
error_code
(error e, std::string const &msg, throwmode mode = plain)¶ Construct an object of type error_code.
- Parameters
e
: The parametere
holds the hpx::error code the new exception should encapsulate.msg
: The parametermsg
holds the error message the new exception should encapsulate.mode
: The parametermode
specifies whether the constructed hpx::error_code belongs to the error category hpx_category (if mode is plain, this is the default) or to the category hpx_category_rethrow (if mode is rethrow).
- Exceptions
std::bad_alloc
: (if allocation of a copy of the passed string fails).
-
error_code
(error e, std::string const &msg, char const *func, char const *file, long line, throwmode mode = plain)¶ Construct an object of type error_code.
- Parameters
e
: The parametere
holds the hpx::error code the new exception should encapsulate.msg
: The parametermsg
holds the error message the new exception should encapsulate.func
: The name of the function where the error was raised.file
: The file name of the code where the error was raised.line
: The line number of the code line where the error was raised.mode
: The parametermode
specifies whether the constructed hpx::error_code belongs to the error category hpx_category (if mode is plain, this is the default) or to the category hpx_category_rethrow (if mode is rethrow).
- Exceptions
std::bad_alloc
: (if allocation of a copy of the passed string fails).
-
std::string
get_message
() const¶ Return a reference to the error message stored in the hpx::error_code.
- Exceptions
nothing
:
-
void
clear
()¶ Clear this error_code object. The postconditions of invoking this method are.
- value() == hpx::success and category() == hpx::get_hpx_category()
-
error_code
(error_code const &rhs)¶ Copy constructor for error_code
- Note
- This function maintains the error category of the left hand side if the right hand side is a success code.
-
error_code &
operator=
(error_code const &rhs)¶ Assignment operator for error_code
- Note
- This function maintains the error category of the left hand side if the right hand side is a success code.
Private Functions
-
error_code
(std::exception_ptr const &e)¶
Private Members
-
std::exception_ptr
exception_
¶
-
class
exception
: public system_error¶ - #include <exception.hpp>
A hpx::exception is the main exception type used by HPX to report errors.
The hpx::exception type is the main exception type used by HPX to report errors. Any exceptions thrown by functions in the HPX library are either of this type or of a type derived from it. This implies that it is always safe to use this type only in catch statements guarding HPX library calls.
Subclassed by hpx::exception_list, hpx::parallel::v2::task_canceled_exception
Public Functions
-
exception
(error e = success)¶ Construct a hpx::exception from a hpx::error.
- Parameters
e
: The parametere
holds the hpx::error code the new exception should encapsulate.
-
exception
(boost::system::system_error const &e)¶ Construct a hpx::exception from a boost::system_error.
-
exception
(boost::system::error_code const &e)¶ Construct a hpx::exception from a boost::system::error_code (this is new for Boost V1.69). This constructor is required to compensate for the changes introduced as a resolution to LWG3162 (https://cplusplus.github.io/LWG/issue3162).
-
exception
(error e, char const *msg, throwmode mode = plain)¶ Construct a hpx::exception from a hpx::error and an error message.
- Parameters
e
: The parametere
holds the hpx::error code the new exception should encapsulate.msg
: The parametermsg
holds the error message the new exception should encapsulate.mode
: The parametermode
specifies whether the returned hpx::error_code belongs to the error category hpx_category (if mode is plain, this is the default) or to the category hpx_category_rethrow (if mode is rethrow).
-
exception
(error e, std::string const &msg, throwmode mode = plain)¶ Construct a hpx::exception from a hpx::error and an error message.
- Parameters
e
: The parametere
holds the hpx::error code the new exception should encapsulate.msg
: The parametermsg
holds the error message the new exception should encapsulate.mode
: The parametermode
specifies whether the returned hpx::error_code belongs to the error category hpx_category (if mode is plain, this is the default) or to the category hpx_category_rethrow (if mode is rethrow).
-
~exception
()¶ Destruct a hpx::exception
- Exceptions
nothing
:
-
error
get_error
() const¶ The function get_error() returns the hpx::error code stored in the referenced instance of a hpx::exception. It returns the hpx::error code this exception instance was constructed from.
- Exceptions
nothing
:
-
error_code
get_error_code
(throwmode mode = plain) const¶ The function get_error_code() returns a hpx::error_code which represents the same error condition as this hpx::exception instance.
- Parameters
mode
: The parametermode
specifies whether the returned hpx::error_code belongs to the error category hpx_category (if mode is plain, this is the default) or to the category hpx_category_rethrow (if mode is rethrow).
-
-
class
exception_list
: public hpx::exception¶ - #include <exception_list.hpp>
The class exception_list is a container of exception_ptr objects parallel algorithms may use to communicate uncaught exceptions encountered during parallel execution to the caller of the algorithm
The type exception_list::const_iterator fulfills the requirements of a forward iterator.
Public Types
-
typedef exception_list_type::const_iterator
iterator
¶ bidirectional iterator
Public Functions
-
std::size_t
size
() const¶ The number of exception_ptr objects contained within the exception_list.
- Note
- Complexity: Constant time.
-
exception_list_type::const_iterator
begin
() const¶ An iterator referring to the first exception_ptr object contained within the exception_list.
-
exception_list_type::const_iterator
end
() const¶ An iterator which is the past-the-end value for the exception_list.
Private Types
-
typedef std::list<std::exception_ptr>
exception_list_type
¶
-
typedef exception_list_type::const_iterator
-
struct
guided_chunk_size
¶ - #include <guided_chunk_size.hpp>
Iterations are dynamically assigned to threads in blocks as threads request them until no blocks remain to be assigned. Similar to dynamic_chunk_size except that the block size decreases each time a number of loop iterations is given to a thread. The size of the initial block is proportional to number_of_iterations / number_of_cores. Subsequent blocks are proportional to number_of_iterations_remaining / number_of_cores. The optional chunk size parameter defines the minimum block size. The default chunk size is 1.
- Note
- This executor parameters type is equivalent to OpenMP’s GUIDED scheduling directive.
Public Functions
-
guided_chunk_size
(std::size_t min_chunk_size = 1)¶ Construct a guided_chunk_size executor parameters object
- Parameters
min_chunk_size
: [in] The optional minimal chunk size to use as the minimal number of loop iterations to schedule together. The default minimal chunk size is 1.
-
struct
invoke
¶ - #include <invoke.hpp>
Public Functions
-
template<typename F, typename... Ts>HPX_HOST_DEVICE util::invoke_result<F, Ts...>::type hpx::util::functional::invoke::operator()(F && f, Ts &&... vs) const
-
-
template<typename
R
>
structinvoke_r
¶ - #include <invoke.hpp>
Public Functions
-
template<typename F, typename... Ts>HPX_HOST_DEVICE R hpx::util::functional::invoke_r::operator()(F && f, Ts &&... vs) const
-
-
template<typename
T
>
structis_async_execution_policy
: public execution::detail::is_async_execution_policy<hpx::util::decay<T>::type>¶ - #include <is_execution_policy.hpp>
Extension: Detect whether given execution policy makes algorithms asynchronous
- The type is_async_execution_policy can be used to detect asynchronous execution policies for the purpose of excluding function signatures from otherwise ambiguous overload resolution participation.
- If T is the type of a standard or implementation-defined execution policy, is_async_execution_policy<T> shall be publicly derived from integral_constant<bool, true>, otherwise from integral_constant<bool, false>.
- The behavior of a program that adds specializations for is_async_execution_policy is undefined.
-
template<typename
T
>
structis_execution_policy
: public execution::detail::is_execution_policy<hpx::util::decay<T>::type>¶ - #include <is_execution_policy.hpp>
- The type is_execution_policy can be used to detect execution policies for the purpose of excluding function signatures from otherwise ambiguous overload resolution participation.
- If T is the type of a standard or implementation-defined execution policy, is_execution_policy<T> shall be publicly derived from integral_constant<bool, true>, otherwise from integral_constant<bool, false>.
- The behavior of a program that adds specializations for is_execution_policy is undefined.
-
template<typename
T
>
structis_parallel_execution_policy
: public execution::detail::is_parallel_execution_policy<hpx::util::decay<T>::type>¶ - #include <is_execution_policy.hpp>
Extension: Detect whether given execution policy enables parallelization
- The type is_parallel_execution_policy can be used to detect parallel execution policies for the purpose of excluding function signatures from otherwise ambiguous overload resolution participation.
- If T is the type of a standard or implementation-defined execution policy, is_parallel_execution_policy<T> shall be publicly derived from integral_constant<bool, true>, otherwise from integral_constant<bool, false>.
- The behavior of a program that adds specializations for is_parallel_execution_policy is undefined.
-
template<typename
T
>
structis_sequenced_execution_policy
: public execution::detail::is_sequenced_execution_policy<hpx::util::decay<T>::type>¶ - #include <is_execution_policy.hpp>
Extension: Detect whether given execution policy does not enable parallelization
- The type is_sequenced_execution_policy can be used to detect non-parallel execution policies for the purpose of excluding function signatures from otherwise ambiguous overload resolution participation.
- If T is the type of a standard or implementation-defined execution policy, is_sequenced_execution_policy<T> shall be publicly derived from integral_constant<bool, true>, otherwise from integral_constant<bool, false>.
- The behavior of a program that adds specializations for is_sequenced_execution_policy is undefined.
-
struct
launch
: public detail::policy_holder<>¶ - #include <launch_policy.hpp>
Launch policies for hpx::async etc.
Public Functions
-
launch
()¶ Default constructor. This creates a launch policy representing all possible launch modes
Public Static Attributes
-
const detail::fork_policy
fork
¶ Predefined launch policy representing asynchronous execution.The new thread is executed in a preferred way
-
const detail::sync_policy
sync
¶ Predefined launch policy representing synchronous execution.
-
const detail::deferred_policy
deferred
¶ Predefined launch policy representing deferred execution.
-
const detail::apply_policy
apply
¶ Predefined launch policy representing fire and forget execution.
-
const detail::select_policy_generator
select
¶ Predefined launch policy representing delayed policy selection.
-
-
class
numa_domain
¶ - #include <partitioner.hpp>
Private Static Attributes
-
const std::size_t
invalid_numa_domain_id
= std::size_t(-1)¶
Friends
-
friend
hpx::resource::numa_domain::pu
-
friend
hpx::resource::numa_domain::core
-
const std::size_t
-
struct
parallel_execution_tag
¶ - #include <execution_fwd.hpp>
Function invocations executed by a group of parallel execution agents execute in unordered fashion. Any such invocations executing in the same thread are indeterminately sequenced with respect to each other.
- Note
- parallel_execution_tag is weaker than sequenced_execution_tag.
-
struct
parallel_policy
¶ - #include <execution_policy.hpp>
The class parallel_policy is an execution policy type used as a unique type to disambiguate parallel algorithm overloading and indicate that a parallel algorithm’s execution may be parallelized.
Subclassed by hpx::parallel::execution::parallel_policy_shim< Executor, Parameters >
Public Types
-
typedef parallel_executor
executor_type
¶ The type of the executor associated with this execution policy.
-
typedef execution::extract_executor_parameters<executor_type>::type
executor_parameters_type
¶ The type of the associated executor parameters object which is associated with this execution policy
-
typedef parallel_execution_tag
execution_category
¶ The category of the execution agents created by this execution policy.
Public Functions
-
parallel_task_policy
operator()
(task_policy_tag) const¶ Create a new parallel_policy referencing a chunk size.
- Return
- The new parallel_policy
- Parameters
tag
: [in] Specify that the corresponding asynchronous execution policy should be used
-
template<typename
Executor
>
rebind_executor<parallel_policy, Executor, executor_parameters_type>::typeon
(Executor &&exec) const¶ Create a new parallel_policy referencing an executor and a chunk size.
- Return
- The new parallel_policy
- Parameters
exec
: [in] The executor to use for the execution of the parallel algorithm the returned execution policy is used with
-
template<typename ...
Parameters
, typenameParametersType
= typename executor_parameters_join<Parameters...>::type>
rebind_executor<parallel_policy, executor_type, ParametersType>::typewith
(Parameters&&... params) const¶ Create a new parallel_policy from the given execution parameters
- Note
- Requires: is_executor_parameters<Parameters>::value is true
- Return
- The new parallel_policy
- Template Parameters
Parameters
: The type of the executor parameters to associate with this execution policy.
- Parameters
params
: [in] The executor parameters to use for the execution of the parallel algorithm the returned execution policy is used with.
-
executor_type &
executor
()¶ Return the associated executor object.
-
executor_type const &
executor
() const¶ Return the associated executor object.
-
executor_parameters_type &
parameters
()¶ Return the associated executor parameters object.
-
executor_parameters_type const &
parameters
() const¶ Return the associated executor parameters object.
Private Functions
Friends
-
friend
hpx::parallel::execution::parallel_policy::hpx::serialization::access
-
typedef parallel_executor
-
template<typename
Policy
>
structparallel_policy_executor
¶ - #include <parallel_executor.hpp>
A parallel_executor creates groups of parallel execution agents which execute in threads implicitly created by the executor. This executor prefers continuing with the creating thread first before executing newly created threads.
This executor conforms to the concepts of a TwoWayExecutor, and a BulkTwoWayExecutor
Public Types
-
typedef parallel_execution_tag
execution_category
¶ Associate the parallel_execution_tag executor tag type as a default with this executor.
-
typedef static_chunk_size
executor_parameters_type
¶ Associate the static_chunk_size executor parameters type as a default with this executor.
Public Functions
-
parallel_policy_executor
(Policy l = detail::get_default_policy<Policy>::call(), std::size_t spread = 4, std::size_t tasks = std::size_t(-1))¶ Create a new parallel executor.
-
typedef parallel_execution_tag
-
template<typename
Executor
, typenameParameters
>
structparallel_policy_shim
: public hpx::parallel::execution::parallel_policy¶ - #include <execution_policy.hpp>
The class parallel_policy_shim is an execution policy type used as a unique type to disambiguate parallel algorithm overloading and indicate that a parallel algorithm’s execution may be parallelized.
Public Types
-
typedef Executor
executor_type
¶ The type of the executor associated with this execution policy.
-
typedef Parameters
executor_parameters_type
¶ The type of the associated executor parameters object which is associated with this execution policy
-
typedef hpx::traits::executor_execution_category<executor_type>::type
execution_category
¶ The category of the execution agents created by this execution policy.
Public Functions
-
parallel_task_policy_shim<Executor, Parameters>
operator()
(task_policy_tag tag) const¶ Create a new parallel_policy referencing a chunk size.
- Return
- The new parallel_policy
- Parameters
tag
: [in] Specify that the corresponding asynchronous execution policy should be used
-
template<typename
Executor_
>
rebind_executor<parallel_policy_shim, Executor_, executor_parameters_type>::typeon
(Executor_ &&exec) const¶ Create a new parallel_policy from the given executor
- Note
- Requires: is_executor<Executor>::value is true
- Return
- The new parallel_policy
- Template Parameters
Executor
: The type of the executor to associate with this execution policy.
- Parameters
exec
: [in] The executor to use for the execution of the parallel algorithm the returned execution policy is used with.
-
template<typename ...
Parameters_
, typenameParametersType
= typename executor_parameters_join<Parameters_...>::type>
rebind_executor<parallel_policy_shim, executor_type, ParametersType>::typewith
(Parameters_&&... params) const¶ Create a new parallel_policy_shim from the given execution parameters
- Note
- Requires: is_executor_parameters<Parameters>::value is true
- Return
- The new parallel_policy_shim
- Template Parameters
Parameters
: The type of the executor parameters to associate with this execution policy.
- Parameters
params
: [in] The executor parameters to use for the execution of the parallel algorithm the returned execution policy is used with.
-
Executor &
executor
()¶ Return the associated executor object.
-
Executor const &
executor
() const¶ Return the associated executor object.
-
Parameters &
parameters
()¶ Return the associated executor parameters object.
-
Parameters const &
parameters
() const¶ Return the associated executor parameters object.
-
typedef Executor
-
struct
parallel_task_policy
¶ - #include <execution_policy.hpp>
Extension: The class parallel_task_policy is an execution policy type used as a unique type to disambiguate parallel algorithm overloading and indicate that a parallel algorithm’s execution may be parallelized.
The algorithm returns a future representing the result of the corresponding algorithm when invoked with the parallel_policy.
Subclassed by hpx::parallel::execution::parallel_task_policy_shim< Executor, Parameters >
Public Types
-
typedef parallel_executor
executor_type
¶ The type of the executor associated with this execution policy.
-
typedef execution::extract_executor_parameters<executor_type>::type
executor_parameters_type
¶ The type of the associated executor parameters object which is associated with this execution policy
-
typedef parallel_execution_tag
execution_category
¶ The category of the execution agents created by this execution policy.
Public Functions
-
parallel_task_policy
operator()
(task_policy_tag) const¶ Create a new parallel_task_policy from itself
- Return
- The new parallel_task_policy
- Parameters
tag
: [in] Specify that the corresponding asynchronous execution policy should be used
-
template<typename
Executor
>
rebind_executor<parallel_task_policy, Executor, executor_parameters_type>::typeon
(Executor &&exec) const¶ Create a new parallel_task_policy from given executor
- Note
- Requires: is_executor<Executor>::value is true
- Return
- The new parallel_task_policy
- Template Parameters
Executor
: The type of the executor to associate with this execution policy.
- Parameters
exec
: [in] The executor to use for the execution of the parallel algorithm the returned execution policy is used with.
-
template<typename ...
Parameters
, typenameParametersType
= typename executor_parameters_join<Parameters...>::type>
rebind_executor<parallel_task_policy, executor_type, ParametersType>::typewith
(Parameters&&... params) const¶ Create a new parallel_policy_shim from the given execution parameters
- Note
- Requires: all parameters are executor_parameters, different parameter types can’t be duplicated
- Return
- The new parallel_policy_shim
- Template Parameters
Parameters
: The type of the executor parameters to associate with this execution policy.
- Parameters
params
: [in] The executor parameters to use for the execution of the parallel algorithm the returned execution policy is used with.
-
executor_type &
executor
()¶ Return the associated executor object.
-
executor_type const &
executor
() const¶ Return the associated executor object.
-
executor_parameters_type &
parameters
()¶ Return the associated executor parameters object.
-
executor_parameters_type const &
parameters
() const¶ Return the associated executor parameters object.
Private Functions
Friends
-
friend
hpx::parallel::execution::parallel_task_policy::hpx::serialization::access
-
typedef parallel_executor
-
template<typename
Executor
, typenameParameters
>
structparallel_task_policy_shim
: public hpx::parallel::execution::parallel_task_policy¶ - #include <execution_policy.hpp>
Extension: The class parallel_task_policy_shim is an execution policy type used as a unique type to disambiguate parallel algorithm overloading based on combining a underlying parallel_task_policy and an executor and indicate that a parallel algorithm’s execution may be parallelized.
Public Types
-
typedef Executor
executor_type
¶ The type of the executor associated with this execution policy.
-
typedef Parameters
executor_parameters_type
¶ The type of the associated executor parameters object which is associated with this execution policy
-
typedef hpx::traits::executor_execution_category<executor_type>::type
execution_category
¶ The category of the execution agents created by this execution policy.
Public Functions
-
parallel_task_policy_shim
operator()
(task_policy_tag tag) const¶ Create a new parallel_task_policy_shim from itself
- Return
- The new sequenced_task_policy
- Parameters
tag
: [in] Specify that the corresponding asynchronous execution policy should be used
-
template<typename
Executor_
>
rebind_executor<parallel_task_policy_shim, Executor_, executor_parameters_type>::typeon
(Executor_ &&exec) const¶ Create a new parallel_task_policy from the given executor
- Note
- Requires: is_executor<Executor>::value is true
- Return
- The new parallel_task_policy
- Template Parameters
Executor
: The type of the executor to associate with this execution policy.
- Parameters
exec
: [in] The executor to use for the execution of the parallel algorithm the returned execution policy is used with.
-
template<typename ...
Parameters_
, typenameParametersType
= typename executor_parameters_join<Parameters_...>::type>
rebind_executor<parallel_task_policy_shim, executor_type, ParametersType>::typewith
(Parameters_&&... params) const¶ Create a new parallel_policy_shim from the given execution parameters
- Note
- Requires: all parameters are executor_parameters, different parameter types can’t be duplicated
- Return
- The new parallel_policy_shim
- Template Parameters
Parameters
: The type of the executor parameters to associate with this execution policy.
- Parameters
params
: [in] The executor parameters to use for the execution of the parallel algorithm the returned execution policy is used with.
-
Executor &
executor
()¶ Return the associated executor object.
-
Executor const &
executor
() const¶ Return the associated executor object.
-
Parameters &
parameters
()¶ Return the associated executor parameters object.
-
Parameters const &
parameters
() const¶ Return the associated executor parameters object.
-
typedef Executor
-
struct
parallel_unsequenced_policy
¶ - #include <execution_policy.hpp>
The class parallel_unsequenced_policy is an execution policy type used as a unique type to disambiguate parallel algorithm overloading and indicate that a parallel algorithm’s execution may be vectorized.
Public Types
-
typedef parallel_executor
executor_type
¶ The type of the executor associated with this execution policy.
-
typedef execution::extract_executor_parameters<executor_type>::type
executor_parameters_type
¶ The type of the associated executor parameters object which is associated with this execution policy
-
typedef parallel_execution_tag
execution_category
¶ The category of the execution agents created by this execution policy.
Public Functions
-
parallel_unsequenced_policy
operator()
(task_policy_tag) const¶ Create a new parallel_unsequenced_policy from itself
- Return
- The new parallel_unsequenced_policy
- Parameters
tag
: [in] Specify that the corresponding asynchronous execution policy should be used
-
executor_type &
executor
()¶ Return the associated executor object.
-
executor_type const &
executor
() const¶ Return the associated executor object.
-
executor_parameters_type &
parameters
()¶ Return the associated executor parameters object.
-
executor_parameters_type const &
parameters
() const¶ Return the associated executor parameters object.
Private Functions
Friends
-
friend
hpx::parallel::execution::parallel_unsequenced_policy::hpx::serialization::access
-
typedef parallel_executor
-
class
partitioner
¶ - #include <partitioner.hpp>
Public Functions
-
partitioner
(util::function_nonser<int(boost::program_options::variables_map &vm)> const &f, boost::program_options::options_description const &desc_cmdline, int argc, char **argv, std::vector<std::string> ini_config, resource::partitioner_mode rpmode = resource::mode_default, runtime_mode mode = runtime_mode_default, )¶
-
partitioner
(util::function_nonser<int(int, char **)> const &f, int argc, char **argv, resource::partitioner_mode rpmode = resource::mode_default, hpx::runtime_mode mode = hpx::runtime_mode_default, )¶
-
partitioner
(util::function_nonser<int(int, char **)> const &f, int argc, char **argv, std::vector<std::string> const &cfg, resource::partitioner_mode rpmode = resource::mode_default, hpx::runtime_mode mode = hpx::runtime_mode_default, )¶
-
partitioner
(int argc, char **argv, resource::partitioner_mode rpmode = resource::mode_default, runtime_mode mode = runtime_mode_default)¶
-
partitioner
(int argc, char **argv, std::vector<std::string> ini_config, resource::partitioner_mode rpmode = resource::mode_default, runtime_mode mode = runtime_mode_default)¶
-
partitioner
(boost::program_options::options_description const &desc_cmdline, int argc, char **argv, resource::partitioner_mode rpmode = resource::mode_default, runtime_mode mode = runtime_mode_default)¶
-
partitioner
(boost::program_options::options_description const &desc_cmdline, int argc, char **argv, std::vector<std::string> ini_config, resource::partitioner_mode rpmode = resource::mode_default, runtime_mode mode = runtime_mode_default)¶
-
partitioner
(std::nullptr_t f, int argc, char **argv, resource::partitioner_mode rpmode = resource::mode_default, hpx::runtime_mode mode = hpx::runtime_mode_default)¶
-
partitioner
(std::nullptr_t f, int argc, char **argv, std::vector<std::string> const &cfg, resource::partitioner_mode rpmode = resource::mode_default, hpx::runtime_mode mode = hpx::runtime_mode_default)¶
-
partitioner
(std::nullptr_t f, boost::program_options::options_description const &desc_cmdline, int argc, char **argv, std::vector<std::string> ini_config, resource::partitioner_mode rpmode = resource::mode_default, runtime_mode mode = runtime_mode_default)¶
-
void
create_thread_pool
(std::string const &name, scheduling_policy sched = scheduling_policy::unspecified, hpx::threads::policies::scheduler_mode = hpx::threads::policies::scheduler_mode::default_mode)¶
-
void
create_thread_pool
(std::string const &name, scheduler_function scheduler_creation)¶
-
void
set_default_pool_name
(std::string const &name)¶
-
const std::string &
get_default_pool_name
() const¶
-
void
add_resource
(hpx::resource::pu const &p, std::string const &pool_name, std::size_t num_threads = 1)¶
-
void
add_resource
(hpx::resource::pu const &p, std::string const &pool_name, bool exclusive, std::size_t num_threads = 1)¶
-
void
add_resource
(std::vector<hpx::resource::pu> const &pv, std::string const &pool_name, bool exclusive = true)¶
-
void
add_resource
(hpx::resource::core const &c, std::string const &pool_name, bool exclusive = true)¶
-
void
add_resource
(std::vector<hpx::resource::core> &cv, std::string const &pool_name, bool exclusive = true)¶
-
void
add_resource
(hpx::resource::numa_domain const &nd, std::string const &pool_name, bool exclusive = true)¶
-
void
add_resource
(std::vector<hpx::resource::numa_domain> const &ndv, std::string const &pool_name, bool exclusive = true)¶
-
std::vector<numa_domain> const &
numa_domains
() const¶
-
std::size_t
get_number_requested_threads
()¶
Private Members
-
detail::partitioner &
partitioner_
¶
-
-
struct
persistent_auto_chunk_size
¶ - #include <persistent_auto_chunk_size.hpp>
Loop iterations are divided into pieces and then assigned to threads. The number of loop iterations combined is determined based on measurements of how long the execution of 1% of the overall number of iterations takes. This executor parameters type makes sure that as many loop iterations are combined as necessary to run for the amount of time specified.
Public Functions
-
persistent_auto_chunk_size
()¶ Construct an persistent_auto_chunk_size executor parameters object
- Note
- Default constructed persistent_auto_chunk_size executor parameter types will use 0 microseconds as the execution time for each chunk and 80 microseconds as the minimal time for which any of the scheduled chunks should run.
-
persistent_auto_chunk_size
(hpx::util::steady_duration const &time_cs)¶ Construct an persistent_auto_chunk_size executor parameters object
- Parameters
time_cs
: The execution time for each chunk.
-
persistent_auto_chunk_size
(hpx::util::steady_duration const &time_cs, hpx::util::steady_duration const &rel_time)¶ Construct an persistent_auto_chunk_size executor parameters object
- Parameters
rel_time
: [in] The time duration to use as the minimum to decide how many loop iterations should be combined.time_cs
: The execution time for each chunk.
-
-
class
pu
¶ - #include <partitioner.hpp>
Public Functions
-
pu
(std::size_t id = invalid_pu_id, core *core = nullptr, std::size_t thread_occupancy = 0)¶
-
std::size_t
id
() const¶
Private Members
-
std::size_t
id_
¶
-
std::size_t
thread_occupancy_
¶
-
std::size_t
thread_occupancy_count_
¶
Private Static Attributes
-
const std::size_t
invalid_pu_id
= std::size_t(-1)¶
Friends
-
friend
hpx::resource::pu::core
-
friend
hpx::resource::pu::numa_domain
-
-
template<typename
Executor_
, typenameParameters_
>
structrebind
¶ - #include <execution_policy.hpp>
Rebind the type of executor used by this execution policy. The execution category of Executor shall not be weaker than that of this execution policy
Public Types
-
typedef parallel_task_policy_shim<Executor_, Parameters_>
type
¶ The type of the rebound execution policy.
-
typedef parallel_task_policy_shim<Executor_, Parameters_>
-
template<typename
Executor_
, typenameParameters_
>
structrebind
¶ - #include <execution_policy.hpp>
Rebind the type of executor used by this execution policy. The execution category of Executor shall not be weaker than that of this execution policy
Public Types
-
typedef sequenced_policy_shim<Executor_, Parameters_>
type
¶ The type of the rebound execution policy.
-
typedef sequenced_policy_shim<Executor_, Parameters_>
-
template<typename
Executor_
, typenameParameters_
>
structrebind
¶ - #include <execution_policy.hpp>
Rebind the type of executor used by this execution policy. The execution category of Executor shall not be weaker than that of this execution policy
Public Types
-
template<>
typedef sequenced_task_policy_shim<Executor_, Parameters_>type
¶ The type of the rebound execution policy.
-
template<>
-
template<typename
Executor_
, typenameParameters_
>
structrebind
¶ - #include <execution_policy.hpp>
Rebind the type of executor used by this execution policy. The execution category of Executor shall not be weaker than that of this execution policy
Public Types
-
template<>
typedef parallel_task_policy_shim<Executor_, Parameters_>type
¶ The type of the rebound execution policy.
-
template<>
-
template<typename
Executor_
, typenameParameters_
>
structrebind
¶ - #include <execution_policy.hpp>
Rebind the type of executor used by this execution policy. The execution category of Executor shall not be weaker than that of this execution policy
Public Types
-
template<>
typedef parallel_policy_shim<Executor_, Parameters_>type
¶ The type of the rebound execution policy.
-
template<>
-
template<typename
Executor_
, typenameParameters_
>
structrebind
¶ - #include <execution_policy.hpp>
Rebind the type of executor used by this execution policy. The execution category of Executor shall not be weaker than that of this execution policy
Public Types
-
typedef parallel_policy_shim<Executor_, Parameters_>
type
¶ The type of the rebound execution policy.
-
typedef parallel_policy_shim<Executor_, Parameters_>
-
template<typename
Executor_
, typenameParameters_
>
structrebind
¶ - #include <execution_policy.hpp>
Rebind the type of executor used by this execution policy. The execution category of Executor shall not be weaker than that of this execution policy
Public Types
-
typedef sequenced_task_policy_shim<Executor_, Parameters_>
type
¶ The type of the rebound execution policy.
-
typedef sequenced_task_policy_shim<Executor_, Parameters_>
-
template<typename
Executor_
, typenameParameters_
>
structrebind
¶ - #include <execution_policy.hpp>
Rebind the type of executor used by this execution policy. The execution category of Executor shall not be weaker than that of this execution policy
Public Types
-
template<>
typedef sequenced_policy_shim<Executor_, Parameters_>type
¶ The type of the rebound execution policy.
-
template<>
-
struct
sequenced_execution_tag
¶ - #include <execution_fwd.hpp>
Function invocations executed by a group of sequential execution agents execute in sequential order.
-
struct
sequenced_executor
¶ - #include <sequenced_executor.hpp>
A sequential_executor creates groups of sequential execution agents which execute in the calling thread. The sequential order is given by the lexicographical order of indices in the index space.
-
struct
sequenced_policy
¶ - #include <execution_policy.hpp>
The class sequenced_policy is an execution policy type used as a unique type to disambiguate parallel algorithm overloading and require that a parallel algorithm’s execution may not be parallelized.
Subclassed by hpx::parallel::execution::sequenced_policy_shim< Executor, Parameters >
Public Types
-
typedef sequenced_executor
executor_type
¶ The type of the executor associated with this execution policy.
-
typedef execution::extract_executor_parameters<executor_type>::type
executor_parameters_type
¶ The type of the associated executor parameters object which is associated with this execution policy
-
typedef sequenced_execution_tag
execution_category
¶ The category of the execution agents created by this execution policy.
Public Functions
-
sequenced_task_policy
operator()
(task_policy_tag) const¶ Create a new sequenced_task_policy.
- Return
- The new sequenced_task_policy
- Parameters
tag
: [in] Specify that the corresponding asynchronous execution policy should be used
-
template<typename
Executor
>
rebind_executor<sequenced_policy, Executor, executor_parameters_type>::typeon
(Executor &&exec) const¶ Create a new sequenced_policy from the given executor
- Note
- Requires: is_executor<Executor>::value is true
- Return
- The new sequenced_policy
- Template Parameters
Executor
: The type of the executor to associate with this execution policy.
- Parameters
exec
: [in] The executor to use for the execution of the parallel algorithm the returned execution policy is used with.
-
template<typename ...
Parameters
, typenameParametersType
= typename executor_parameters_join<Parameters...>::type>
rebind_executor<sequenced_policy, executor_type, ParametersType>::typewith
(Parameters&&... params) const¶ Create a new sequenced_policy from the given execution parameters
- Note
- Requires: all parameters are executor_parameters, different parameter types can’t be duplicated
- Return
- The new sequenced_policy
- Template Parameters
Parameters
: The type of the executor parameters to associate with this execution policy.
- Parameters
params
: [in] The executor parameters to use for the execution of the parallel algorithm the returned execution policy is used with.
-
executor_type &
executor
()¶ Return the associated executor object. Return the associated executor object.
-
executor_type const &
executor
() const¶ Return the associated executor object.
-
executor_parameters_type &
parameters
()¶ Return the associated executor parameters object.
-
executor_parameters_type const &
parameters
() const¶ Return the associated executor parameters object.
Private Functions
Friends
-
friend
hpx::parallel::execution::sequenced_policy::hpx::serialization::access
-
typedef sequenced_executor
-
template<typename
Executor
, typenameParameters
>
structsequenced_policy_shim
: public hpx::parallel::execution::sequenced_policy¶ - #include <execution_policy.hpp>
The class sequenced_policy is an execution policy type used as a unique type to disambiguate parallel algorithm overloading and require that a parallel algorithm’s execution may not be parallelized.
Public Types
-
typedef Executor
executor_type
¶ The type of the executor associated with this execution policy.
-
typedef Parameters
executor_parameters_type
¶ The type of the associated executor parameters object which is associated with this execution policy
-
typedef hpx::traits::executor_execution_category<executor_type>::type
execution_category
¶ The category of the execution agents created by this execution policy.
Public Functions
-
sequenced_task_policy_shim<Executor, Parameters>
operator()
(task_policy_tag tag) const¶ Create a new sequenced_task_policy.
- Return
- The new sequenced_task_policy_shim
- Parameters
tag
: [in] Specify that the corresponding asynchronous execution policy should be used
-
template<typename
Executor_
>
rebind_executor<sequenced_policy_shim, Executor_, executor_parameters_type>::typeon
(Executor_ &&exec) const¶ Create a new sequenced_policy from the given executor
- Note
- Requires: is_executor<Executor>::value is true
- Return
- The new sequenced_policy
- Template Parameters
Executor
: The type of the executor to associate with this execution policy.
- Parameters
exec
: [in] The executor to use for the execution of the parallel algorithm the returned execution policy is used with.
-
template<typename ...
Parameters_
, typenameParametersType
= typename executor_parameters_join<Parameters_...>::type>
rebind_executor<sequenced_policy_shim, executor_type, ParametersType>::typewith
(Parameters_&&... params) const¶ Create a new sequenced_policy_shim from the given execution parameters
- Note
- Requires: all parameters are executor_parameters, different parameter types can’t be duplicated
- Return
- The new sequenced_policy_shim
- Template Parameters
Parameters
: The type of the executor parameters to associate with this execution policy.
- Parameters
params
: [in] The executor parameters to use for the execution of the parallel algorithm the returned execution policy is used with.
-
Executor &
executor
()¶ Return the associated executor object.
-
Executor const &
executor
() const¶ Return the associated executor object.
-
Parameters &
parameters
()¶ Return the associated executor parameters object.
-
Parameters const &
parameters
() const¶ Return the associated executor parameters object.
-
typedef Executor
-
struct
sequenced_task_policy
¶ - #include <execution_policy.hpp>
Extension: The class sequenced_task_policy is an execution policy type used as a unique type to disambiguate parallel algorithm overloading and indicate that a parallel algorithm’s execution may not be parallelized (has to run sequentially).
The algorithm returns a future representing the result of the corresponding algorithm when invoked with the sequenced_policy.
Subclassed by hpx::parallel::execution::sequenced_task_policy_shim< Executor, Parameters >
Public Types
-
typedef sequenced_executor
executor_type
¶ The type of the executor associated with this execution policy.
-
typedef execution::extract_executor_parameters<executor_type>::type
executor_parameters_type
¶ The type of the associated executor parameters object which is associated with this execution policy
-
typedef sequenced_execution_tag
execution_category
¶ The category of the execution agents created by this execution policy.
Public Functions
-
sequenced_task_policy
operator()
(task_policy_tag) const¶ Create a new sequenced_task_policy from itself
- Return
- The new sequenced_task_policy
- Parameters
tag
: [in] Specify that the corresponding asynchronous execution policy should be used
-
template<typename
Executor
>
rebind_executor<sequenced_task_policy, Executor, executor_parameters_type>::typeon
(Executor &&exec) const¶ Create a new sequenced_task_policy from the given executor
- Note
- Requires: is_executor<Executor>::value is true
- Return
- The new sequenced_task_policy
- Template Parameters
Executor
: The type of the executor to associate with this execution policy.
- Parameters
exec
: [in] The executor to use for the execution of the parallel algorithm the returned execution policy is used with.
-
template<typename ...
Parameters
, typenameParametersType
= typename executor_parameters_join<Parameters...>::type>
rebind_executor<sequenced_task_policy, executor_type, ParametersType>::typewith
(Parameters&&... params) const¶ Create a new sequenced_task_policy from the given execution parameters
- Note
- Requires: all parameters are executor_parameters, different parameter types can’t be duplicated
- Return
- The new sequenced_task_policy
- Template Parameters
Parameters
: The type of the executor parameters to associate with this execution policy.
- Parameters
params
: [in] The executor parameters to use for the execution of the parallel algorithm the returned execution policy is used with.
-
executor_type &
executor
()¶ Return the associated executor object.
-
executor_type const &
executor
() const¶ Return the associated executor object.
-
executor_parameters_type &
parameters
()¶ Return the associated executor parameters object.
-
executor_parameters_type const &
parameters
() const¶ Return the associated executor parameters object.
Private Functions
Friends
-
friend
hpx::parallel::execution::sequenced_task_policy::hpx::serialization::access
-
typedef sequenced_executor
-
template<typename
Executor
, typenameParameters
>
structsequenced_task_policy_shim
: public hpx::parallel::execution::sequenced_task_policy¶ - #include <execution_policy.hpp>
Extension: The class sequenced_task_policy_shim is an execution policy type used as a unique type to disambiguate parallel algorithm overloading based on combining a underlying sequenced_task_policy and an executor and indicate that a parallel algorithm’s execution may not be parallelized (has to run sequentially).
The algorithm returns a future representing the result of the corresponding algorithm when invoked with the sequenced_policy.
Public Types
-
typedef Executor
executor_type
¶ The type of the executor associated with this execution policy.
-
typedef Parameters
executor_parameters_type
¶ The type of the associated executor parameters object which is associated with this execution policy
-
typedef hpx::traits::executor_execution_category<executor_type>::type
execution_category
¶ The category of the execution agents created by this execution policy.
Public Functions
-
sequenced_task_policy_shim const &
operator()
(task_policy_tag tag) const¶ Create a new sequenced_task_policy from itself
- Return
- The new sequenced_task_policy
- Parameters
tag
: [in] Specify that the corresponding asynchronous execution policy should be used
-
template<typename
Executor_
>
rebind_executor<sequenced_task_policy_shim, Executor_, executor_parameters_type>::typeon
(Executor_ &&exec) const¶ Create a new sequenced_task_policy from the given executor
- Note
- Requires: is_executor<Executor>::value is true
- Return
- The new sequenced_task_policy
- Template Parameters
Executor
: The type of the executor to associate with this execution policy.
- Parameters
exec
: [in] The executor to use for the execution of the parallel algorithm the returned execution policy is used with.
-
template<typename ...
Parameters_
, typenameParametersType
= typename executor_parameters_join<Parameters_...>::type>
rebind_executor<sequenced_task_policy_shim, executor_type, ParametersType>::typewith
(Parameters_&&... params) const¶ Create a new sequenced_task_policy_shim from the given execution parameters
- Note
- Requires: all parameters are executor_parameters, different parameter types can’t be duplicated
- Return
- The new sequenced_task_policy_shim
- Template Parameters
Parameters
: The type of the executor parameters to associate with this execution policy.
- Parameters
params
: [in] The executor parameters to use for the execution of the parallel algorithm the returned execution policy is used with.
-
Executor &
executor
()¶ Return the associated executor object.
-
Executor const &
executor
() const¶ Return the associated executor object.
-
Parameters &
parameters
()¶ Return the associated executor parameters object.
-
Parameters const &
parameters
() const¶ Return the associated executor parameters object.
-
typedef Executor
-
struct
static_chunk_size
¶ - #include <static_chunk_size.hpp>
Loop iterations are divided into pieces of size chunk_size and then assigned to threads. If chunk_size is not specified, the iterations are evenly (if possible) divided contiguously among the threads.
- Note
- This executor parameters type is equivalent to OpenMP’s STATIC scheduling directive.
Public Functions
-
static_chunk_size
()¶ Construct a static_chunk_size executor parameters object
- Note
- By default the number of loop iterations is determined from the number of available cores and the overall number of loop iterations to schedule.
-
static_chunk_size
(std::size_t chunk_size)¶ Construct a static_chunk_size executor parameters object
- Parameters
chunk_size
: [in] The optional chunk size to use as the number of loop iterations to run on a single thread.
-
template<typename
ExPolicy
= parallel::execution::parallel_policy>
classtask_block
¶ - #include <task_block.hpp>
The class task_block defines an interface for forking and joining parallel tasks. The define_task_block and define_task_block_restore_thread function templates create an object of type task_block and pass a reference to that object to a user-provided callable object.
An object of class task_block cannot be constructed, destroyed, copied, or moved except by the implementation of the task region library. Taking the address of a task_block object via operator& or addressof is ill formed. The result of obtaining its address by any other means is unspecified.
is active if it was created by the nearest enclosing task block, where “task block” refers to an invocation of define_task_block or define_task_block_restore_thread and “nearest
enclosing” means the most recent invocation that has not yet completed. Code designated for execution in another thread by means other than the facilities in this section (e.g., using thread or async) are not enclosed in the task region and a
task_block passed to (or captured by) such code is not active within that code. Performing any operation on a task_block that is not active results in undefined behavior.The task_block that is active before a specific call to the run member function is not active within the asynchronous function that invoked run. (The invoked function should not, therefore, capture the task_block from the surrounding block.)
Example: define_task_block([&](auto& tr) { tr.run([&] { tr.run([] { f(); }); // Error: tr is not active define_task_block([&](auto& tr) { // Nested task block tr.run(f); // OK: inner tr is active /// ... }); }); /// ... });
- Template Parameters
ExPolicy
: The execution policy an instance of a task_block was created with. This defaults to parallel_policy.
Public Types
-
typedef ExPolicy
execution_policy
¶ Refers to the type of the execution policy used to create the task_block.
Public Functions
-
execution_policy const &
get_execution_policy
() const¶ Return the execution policy instance used to create this task_block
-
template<typename
F
, typename ...Ts
>
voidrun
(F &&f, Ts&&... ts)¶ Causes the expression f() to be invoked asynchronously. The invocation of f is permitted to run on an unspecified thread in an unordered fashion relative to the sequence of operations following the call to run(f) (the continuation), or indeterminately sequenced within the same thread as the continuation.
The call to run synchronizes with the invocation of f. The completion of f() synchronizes with the next invocation of wait on the same task_block or completion of the nearest enclosing task block (i.e., the define_task_block or define_task_block_restore_thread that created this task block).
Requires: F shall be MoveConstructible. The expression, (void)f(), shall be well-formed.
Precondition: this shall be the active task_block.
Postconditions: A call to run may return on a different thread than that on which it was called.
- Note
- The call to run is sequenced before the continuation as if run returns on the same thread. The invocation of the user-supplied callable object f may be immediate or may be delayed until compute resources are available. run might or might not return before invocation of f completes.
- Exceptions
This
: function may throw task_canceled_exception, as described in Exception Handling.
-
template<typename
Executor
, typenameF
, typename ...Ts
>
voidrun
(Executor &exec, F &&f, Ts&&... ts)¶ Causes the expression f() to be invoked asynchronously using the given executor. The invocation of f is permitted to run on an unspecified thread associated with the given executor and in an unordered fashion relative to the sequence of operations following the call to run(exec, f) (the continuation), or indeterminately sequenced within the same thread as the continuation.
The call to run synchronizes with the invocation of f. The completion of f() synchronizes with the next invocation of wait on the same task_block or completion of the nearest enclosing task block (i.e., the define_task_block or define_task_block_restore_thread that created this task block).
Requires: Executor shall be a type modeling the Executor concept. F shall be MoveConstructible. The expression, (void)f(), shall be well-formed.
Precondition: this shall be the active task_block.
Postconditions: A call to run may return on a different thread than that on which it was called.
- Note
- The call to run is sequenced before the continuation as if run returns on the same thread. The invocation of the user-supplied callable object f may be immediate or may be delayed until compute resources are available. run might or might not return before invocation of f completes.
- Exceptions
This
: function may throw task_canceled_exception, as described in Exception Handling.
-
void
wait
()¶ Blocks until the tasks spawned using this task_block have finished.
Precondition: this shall be the active task_block.
Postcondition: All tasks spawned by the nearest enclosing task region have finished. A call to wait may return on a different thread than that on which it was called.
Example: define_task_block([&](auto& tr) { tr.run([&]{ process(a, w, x); }); // Process a[w] through a[x] if (y < x) tr.wait(); // Wait if overlap between [w, x) and [y, z) process(a, y, z); // Process a[y] through a[z] });
- Note
- The call to wait is sequenced before the continuation as if wait returns on the same thread.
- Exceptions
This
: function may throw task_canceled_exception, as described in Exception Handling.
-
ExPolicy &
policy
()¶ Returns a reference to the execution policy used to construct this object.
Precondition: this shall be the active task_block.
-
ExPolicy const &
policy
() const¶ Returns a reference to the execution policy used to construct this object.
Precondition: this shall be the active task_block.
-
class
task_canceled_exception
: public hpx::exception¶ - #include <task_block.hpp>
The class task_canceled_exception defines the type of objects thrown by task_block::run or task_block::wait if they detect that an exception is pending within the current parallel region.
Public Functions
-
task_canceled_exception
()¶
-
-
struct
thread_interrupted
: public exception¶ - #include <exception.hpp>
A hpx::thread_interrupted is the exception type used by HPX to interrupt a running HPX thread.
The hpx::thread_interrupted type is the exception type used by HPX to interrupt a running thread.
A running thread can be interrupted by invoking the interrupt() member function of the corresponding hpx::thread object. When the interrupted thread next executes one of the specified interruption points (or if it is currently blocked whilst executing one) with interruption enabled, then a hpx::thread_interrupted exception will be thrown in the interrupted thread. If not caught, this will cause the execution of the interrupted thread to terminate. As with any other exception, the stack will be unwound, and destructors for objects of automatic storage duration will be executed.
If a thread wishes to avoid being interrupted, it can create an instance of hpx::this_thread::disable_interruption. Objects of this class disable interruption for the thread that created them on construction, and restore the interruption state to whatever it was before on destruction.
void f() { // interruption enabled here { hpx::this_thread::disable_interruption di; // interruption disabled { hpx::this_thread::disable_interruption di2; // interruption still disabled } // di2 destroyed, interruption state restored // interruption still disabled } // di destroyed, interruption state restored // interruption now enabled }
The effects of an instance of hpx::this_thread::disable_interruption can be temporarily reversed by constructing an instance of hpx::this_thread::restore_interruption, passing in the hpx::this_thread::disable_interruption object in question. This will restore the interruption state to what it was when the hpx::this_thread::disable_interruption object was constructed, and then disable interruption again when the hpx::this_thread::restore_interruption object is destroyed.
void g() { // interruption enabled here { hpx::this_thread::disable_interruption di; // interruption disabled { hpx::this_thread::restore_interruption ri(di); // interruption now enabled } // ri destroyed, interruption disable again } // di destroyed, interruption state restored // interruption now enabled }
At any point, the interruption state for the current thread can be queried by calling hpx::this_thread::interruption_enabled().
-
class
thread_pool_base
: public manage_executor¶ - #include <thread_pool_base.hpp>
The base class used to manage a pool of OS threads.
Public Functions
-
virtual hpx::future<void>
resume
() = 0¶ Resumes the thread pool. When the all OS threads on the thread pool have been resumed the returned future will be ready.
- Note
- Can only be called from an HPX thread. Use resume_cb or resume_direct to suspend the pool from outside HPX.
- Return
- A
future<void>
which is ready when the thread pool has been resumed. - Exceptions
hpx::exception
: if called from outside the HPX runtime.
-
virtual void
resume_cb
(std::function<void(void)> callback, error_code &ec = throws, ) = 0¶ Resumes the thread pool. Takes a callback as a parameter which will be called when all OS threads on the thread pool have been resumed.
- Parameters
callback
: [in] called when the thread pool has been resumed.ec
: [in,out] this represents the error status on exit, if this is pre-initialized to hpx::throws the function will throw on error instead.
-
virtual void
resume_direct
(error_code &ec = throws) = 0¶ Resumes the thread pool. Blocks until all OS threads on the thread pool have been resumed.
- Parameters
ec
: [in,out] this represents the error status on exit, if this is pre-initialized to hpx::throws the function will throw on error instead.
-
virtual hpx::future<void>
suspend
() = 0¶ Suspends the thread pool. When the all OS threads on the thread pool have been suspended the returned future will be ready.
- Note
- Can only be called from an HPX thread. Use suspend_cb or suspend_direct to suspend the pool from outside HPX. A thread pool cannot be suspended from an HPX thread running on the pool itself.
- Return
- A
future<void>
which is ready when the thread pool has been suspended. - Exceptions
hpx::exception
: if called from outside the HPX runtime.
-
virtual void
suspend_cb
(std::function<void(void)> callback, error_code &ec = throws, ) = 0¶ Suspends the thread pool. Takes a callback as a parameter which will be called when all OS threads on the thread pool have been suspended.
- Note
- A thread pool cannot be suspended from an HPX thread running on the pool itself.
- Parameters
callback
: [in] called when the thread pool has been suspended.ec
: [in,out] this represents the error status on exit, if this is pre-initialized to hpx::throws the function will throw on error instead.
- Exceptions
hpx::exception
: if called from an HPX thread which is running on the pool itself.
-
virtual void
suspend_direct
(error_code &ec = throws) = 0¶ Suspends the thread pool. Blocks until all OS threads on the thread pool have been suspended.
- Note
- A thread pool cannot be suspended from an HPX thread running on the pool itself.
- Parameters
ec
: [in,out] this represents the error status on exit, if this is pre-initialized to hpx::throws the function will throw on error instead.
- Exceptions
hpx::exception
: if called from an HPX thread which is running on the pool itself.
-
virtual hpx::future<void>
suspend_processing_unit
(std::size_t virt_core) = 0¶ Suspends the given processing unit. When the processing unit has been suspended the returned future will be ready.
- Note
- Can only be called from an HPX thread. Use suspend_processing_unit_cb or to suspend the processing unit from outside HPX. Requires that the pool has threads::policies::enable_elasticity set.
- Return
- A
future<void>
which is ready when the given processing unit has been suspended. - Parameters
virt_core
: [in] The processing unit on the the pool to be suspended. The processing units are indexed starting from 0.
- Exceptions
hpx::exception
: if called from outside the HPX runtime.
-
virtual void
suspend_processing_unit_cb
(std::function<void(void)> callback, std::size_t virt_core, error_code &ec = throws, ) = 0¶ Suspends the given processing unit. Takes a callback as a parameter which will be called when the processing unit has been suspended.
- Note
- Requires that the pool has threads::policies::enable_elasticity set.
- Parameters
callback
: [in] Callback which is called when the processing unit has been suspended.virt_core
: [in] The processing unit to suspend.ec
: [in,out] this represents the error status on exit, if this is pre-initialized to hpx::throws the function will throw on error instead.
-
virtual hpx::future<void>
resume_processing_unit
(std::size_t virt_core) = 0¶ Resumes the given processing unit. When the processing unit has been resumed the returned future will be ready.
- Note
- Can only be called from an HPX thread. Use resume_processing_unit_cb or to resume the processing unit from outside HPX. Requires that the pool has threads::policies::enable_elasticity set.
- Return
- A
future<void>
which is ready when the given processing unit has been resumed. - Parameters
virt_core
: [in] The processing unit on the the pool to be resumed. The processing units are indexed starting from 0.
-
virtual void
resume_processing_unit_cb
(std::function<void(void)> callback, std::size_t virt_core, error_code &ec = throws, ) = 0¶ Resumes the given processing unit. Takes a callback as a parameter which will be called when the processing unit has been resumed.
- Note
- Requires that the pool has threads::policies::enable_elasticity set.
- Parameters
callback
: [in] Callback which is called when the processing unit has been suspended.virt_core
: [in] The processing unit to resume.ec
: [in,out] this represents the error status on exit, if this is pre-initialized to hpx::throws the function will throw on error instead.
-
virtual hpx::future<void>
-
struct
thread_schedule_hint
¶ - #include <thread_enums.hpp>
Public Functions
-
thread_schedule_hint
()¶
-
thread_schedule_hint
(std::int16_t thread_hint)¶
-
thread_schedule_hint
(thread_schedule_hint_mode mode, std::int16_t hint)¶
-
-
struct
unsequenced_execution_tag
¶ - #include <execution_fwd.hpp>
Function invocations executed by a group of vector execution agents are permitted to execute in unordered fashion when executed in different threads, and un-sequenced with respect to one another when executed in the same thread.
- Note
- unsequenced_execution_tag is weaker than parallel_execution_tag.
-
struct
unwrap
¶ - #include <unwrap.hpp>
A helper function object for functionally invoking
hpx::util::unwrap
. For more information please refer to its documentation.
-
struct
unwrap_all
¶ - #include <unwrap.hpp>
A helper function object for functionally invoking
hpx::util::unwrap_all
. For more information please refer to its documentation.
-
template<std::size_t
Depth
>
structunwrap_n
¶ - #include <unwrap.hpp>
A helper function object for functionally invoking
hpx::util::unwrap_n
. For more information please refer to its documentation.
-
template<typename
Sequence
>
structwhen_any_result
¶ - #include <when_any.hpp>
Result type for when_any, contains a sequence of futures and an index pointing to a ready future.
Public Members
-
std::size_t
index
¶ The index of a future which has become ready.
-
Sequence
futures
¶ The sequence of futures as passed to hpx::when_any.
-
std::size_t
-
template<typename
Sequence
>
structwhen_some_result
¶ - #include <when_some.hpp>
Result type for when_some, contains a sequence of futures and indices pointing to ready futures.
Public Members
-
std::vector<std::size_t>
indices
¶ List of indices of futures which became ready.
-
Sequence
futures
¶ The sequence of futures as passed to hpx::when_some.
-
std::vector<std::size_t>
-
namespace
applier
¶ The namespace applier contains all definitions needed for the class hpx::applier::applier and its related functionality. This namespace is part of the HPX core module.
-
namespace
hpx
¶ Unnamed Group
-
error_code
make_error_code
(error e, throwmode mode = plain)¶ Returns a new error_code constructed from the given parameters.
-
error_code
make_error_code
(error e, char const *func, char const *file, long line, throwmode mode = plain)¶
-
error_code
make_error_code
(error e, char const *msg, throwmode mode = plain)¶ Returns error_code(e, msg, mode).
-
error_code
make_error_code
(error e, char const *msg, char const *func, char const *file, long line, throwmode mode = plain)¶
-
error_code
make_error_code
(error e, std::string const &msg, throwmode mode = plain)¶ Returns error_code(e, msg, mode).
-
error_code
make_error_code
(error e, std::string const &msg, char const *func, char const *file, long line, throwmode mode = plain)¶
-
error_code
make_error_code
(std::exception_ptr const &e)¶
Typedefs
-
typedef util::function_nonser<void(boost::system::error_code const&, parcelset::parcel const&)>
parcel_write_handler_type
¶ The type of a function which can be registered as a parcel write handler using the function hpx::set_parcel_write_handler.
- Note
- A parcel write handler is a function which is called by the parcel layer whenever a parcel has been sent by the underlying networking library and if no explicit parcel handler function was specified for the parcel.
Enums
-
enum
error
¶ Possible error conditions.
This enumeration lists all possible error conditions which can be reported from any of the API functions.
Values:
-
success
= 0¶ The operation was successful.
-
no_success
= 1¶ The operation did failed, but not in an unexpected manner.
-
not_implemented
= 2¶ The operation is not implemented.
-
out_of_memory
= 3¶ The operation caused an out of memory condition.
-
bad_action_code
= 4¶
-
bad_component_type
= 5¶ The specified component type is not known or otherwise invalid.
-
network_error
= 6¶ A generic network error occurred.
-
version_too_new
= 7¶ The version of the network representation for this object is too new.
-
version_too_old
= 8¶ The version of the network representation for this object is too old.
-
version_unknown
= 9¶ The version of the network representation for this object is unknown.
-
unknown_component_address
= 10¶
-
duplicate_component_address
= 11¶ The given global id has already been registered.
-
invalid_status
= 12¶ The operation was executed in an invalid status.
-
bad_parameter
= 13¶ One of the supplied parameters is invalid.
-
internal_server_error
= 14¶
-
bad_request
= 16¶
-
repeated_request
= 17¶
-
lock_error
= 18¶
-
duplicate_console
= 19¶ There is more than one console locality.
-
no_registered_console
= 20¶ There is no registered console locality available.
-
startup_timed_out
= 21¶
-
uninitialized_value
= 22¶
-
bad_response_type
= 23¶
-
deadlock
= 24¶
-
assertion_failure
= 25¶
-
null_thread_id
= 26¶ Attempt to invoke a API function from a non-HPX thread.
-
invalid_data
= 27¶
-
yield_aborted
= 28¶ The yield operation was aborted.
-
dynamic_link_failure
= 29¶
-
commandline_option_error
= 30¶ One of the options given on the command line is erroneous.
-
serialization_error
= 31¶ There was an error during serialization of this object.
-
unhandled_exception
= 32¶ An unhandled exception has been caught.
-
kernel_error
= 33¶ The OS kernel reported an error.
-
broken_task
= 34¶ The task associated with this future object is not available anymore.
-
task_moved
= 35¶ The task associated with this future object has been moved.
-
task_already_started
= 36¶ The task associated with this future object has already been started.
-
future_already_retrieved
= 37¶ The future object has already been retrieved.
-
promise_already_satisfied
= 38¶ The value for this future object has already been set.
-
future_does_not_support_cancellation
= 39¶ The future object does not support cancellation.
-
future_can_not_be_cancelled
= 40¶ The future can’t be canceled at this time.
-
no_state
= 41¶ The future object has no valid shared state.
-
broken_promise
= 42¶ The promise has been deleted.
-
thread_resource_error
= 43¶
-
future_cancelled
= 44¶
-
thread_cancelled
= 45¶
-
thread_not_interruptable
= 46¶
-
duplicate_component_id
= 47¶ The component type has already been registered.
-
unknown_error
= 48¶ An unknown error occurred.
-
bad_plugin_type
= 49¶ The specified plugin type is not known or otherwise invalid.
-
filesystem_error
= 50¶ The specified file does not exist or other filesystem related error.
-
bad_function_call
= 51¶ equivalent of std::bad_function_call
-
task_canceled_exception
= 52¶
-
task_block_not_active
= 53¶ task_region is not active
-
out_of_range
= 54¶ Equivalent to std::out_of_range.
-
length_error
= 55¶ Equivalent to std::length_error.
-
migration_needs_retry
= 56¶ migration failed because of global race, retry
-
-
enum
throwmode
¶ Encode error category for new error_code.
Values:
-
plain
= 0¶
-
rethrow
= 1¶
-
-
enum
runtime_mode
¶ A HPX runtime can be executed in two different modes: console mode and worker mode.
Values:
-
runtime_mode_invalid
= -1¶
-
runtime_mode_console
= 0¶ The runtime is the console locality.
-
runtime_mode_worker
= 1¶ The runtime is a worker locality.
-
runtime_mode_connect
= 2¶ The runtime is a worker locality connecting late
-
runtime_mode_default
= 3¶ The runtime mode will be determined based on the command line arguments
-
runtime_mode_last
¶
-
Functions
-
int
init
(util::function_nonser<int(boost::program_options::variables_map &vm)> const &f, boost::program_options::options_description const &desc_cmdline, int argc, char **argv, std::vector<std::string> const &cfg, startup_function_type startup = startup_function_type(), shutdown_function_type shutdown = shutdown_function_type(), hpx::runtime_mode mode = hpx::runtime_mode_default, )¶ Main entry point for launching the HPX runtime system.
This is the main entry point for any HPX application. This function (or one of its overloads below) should be called from the users
main()
function. It will set up the HPX runtime environment and schedule the function given byf
as a HPX thread. This overload will not callhpx_main
.- Return
- The function returns the value, which has been returned from the user supplied
f
. - Note
- If the parameter
mode
is not given (defaulted), the created runtime system instance will be executed in console or worker mode depending on the command line arguments passed inargc
/argv
. Otherwise it will be executed as specified by the parametermode
. - Parameters
f
: [in] The function to be scheduled as an HPX thread. Usually this function represents the main entry point of any HPX application. Iff
isnullptr
the HPX runtime environment will be started without invokingf
.desc_cmdline
: [in] This parameter may hold the description of additional command line arguments understood by the application. These options will be prepended to the default command line options understood by hpx::init (see description below).argc
: [in] The number of command line arguments passed inargv
. This is usually the unchanged value as passed by the operating system (tomain()
).argv
: [in] The command line arguments for this application, usually that is the value as passed by the operating system (tomain()
).cfg
: A list of configuration settings which will be added to the system configuration before the runtime instance is run. Each of the entries in this list must have the format of a fully defined key/value pair from an ini-file (for instance ‘hpx.component.enabled=1’)startup
: [in] A function to be executed inside a HPX thread beforef
is called. If this parameter is not given no function will be executed.shutdown
: [in] A function to be executed inside an HPX thread while hpx::finalize is executed. If this parameter is not given no function will be executed.mode
: [in] The mode the created runtime environment should be initialized in. There has to be exactly one locality in each HPX application which is executed in console mode (hpx::runtime_mode_console), all other localities have to be run in worker mode (hpx::runtime_mode_worker). Normally this is set up automatically, but sometimes it is necessary to explicitly specify the mode.
-
int
init
(int (*f)(boost::program_options::variables_map &vm), boost::program_options::options_description const &desc_cmdline, int argc, char **argv, startup_function_type startup = startup_function_type(), shutdown_function_type shutdown = shutdown_function_type(), hpx::runtime_mode mode = hpx::runtime_mode_default, )¶ Main entry point for launching the HPX runtime system.
This is the main entry point for any HPX application. This function (or one of its overloads below) should be called from the users
main()
function. It will set up the HPX runtime environment and schedule the function given byf
as a HPX thread. This overload will not callhpx_main
.- Return
- The function returns the value, which has been returned from the user supplied
f
. - Note
- If the parameter
mode
is not given (defaulted), the created runtime system instance will be executed in console or worker mode depending on the command line arguments passed inargc
/argv
. Otherwise it will be executed as specified by the parametermode
. - Parameters
f
: [in] The function to be scheduled as an HPX thread. Usually this function represents the main entry point of any HPX application. Iff
isnullptr
the HPX runtime environment will be started without invokingf
.desc_cmdline
: [in] This parameter may hold the description of additional command line arguments understood by the application. These options will be prepended to the default command line options understood by hpx::init (see description below).argc
: [in] The number of command line arguments passed inargv
. This is usually the unchanged value as passed by the operating system (tomain()
).argv
: [in] The command line arguments for this application, usually that is the value as passed by the operating system (tomain()
).startup
: [in] A function to be executed inside a HPX thread beforef
is called. If this parameter is not given no function will be executed.shutdown
: [in] A function to be executed inside an HPX thread while hpx::finalize is executed. If this parameter is not given no function will be executed.mode
: [in] The mode the created runtime environment should be initialized in. There has to be exactly one locality in each HPX application which is executed in console mode (hpx::runtime_mode_console), all other localities have to be run in worker mode (hpx::runtime_mode_worker). Normally this is set up automatically, but sometimes it is necessary to explicitly specify the mode.
-
int
init
(boost::program_options::options_description const &desc_cmdline, int argc, char **argv, startup_function_type startup = startup_function_type(), shutdown_function_type shutdown = shutdown_function_type(), hpx::runtime_mode mode = hpx::runtime_mode_default)¶ Main entry point for launching the HPX runtime system.
This is a simplified main entry point, which can be used to set up the runtime for an HPX application (the runtime system will be set up in console mode or worker mode depending on the command line settings).
In console mode it will execute the user supplied function
hpx_main
, in worker mode it will execute an emptyhpx_main
.- Return
- The function returns the value, which has been returned from
hpx_main
(or 0 when executed in worker mode). - Note
- If the parameter
mode
is not given (defaulted), the created runtime system instance will be executed in console or worker mode depending on the command line arguments passed inargc
/argv
. Otherwise it will be executed as specified by the parametermode
. - Parameters
desc_cmdline
: [in] This parameter may hold the description of additional command line arguments understood by the application. These options will be prepended to the default command line options understood by hpx::init (see description below).argc
: [in] The number of command line arguments passed inargv
. This is usually the unchanged value as passed by the operating system (tomain()
).argv
: [in] The command line arguments for this application, usually that is the value as passed by the operating system (tomain()
).startup
: [in] A function to be executed inside a HPX thread beforef
is called. If this parameter is not given no function will be executed.shutdown
: [in] A function to be executed inside an HPX thread while hpx::finalize is executed. If this parameter is not given no function will be executed.mode
: [in] The mode the created runtime environment should be initialized in. There has to be exactly one locality in each HPX application which is executed in console mode (hpx::runtime_mode_console), all other localities have to be run in worker mode (hpx::runtime_mode_worker). Normally this is set up automatically, but sometimes it is necessary to explicitly specify the mode.
-
int
init
(boost::program_options::options_description const &desc_cmdline, int argc, char **argv, std::vector<std::string> const &cfg, startup_function_type startup = startup_function_type(), shutdown_function_type shutdown = shutdown_function_type(), hpx::runtime_mode mode = hpx::runtime_mode_default)¶ Main entry point for launching the HPX runtime system.
This is a simplified main entry point, which can be used to set up the runtime for an HPX application (the runtime system will be set up in console mode or worker mode depending on the command line settings).
In console mode it will execute the user supplied function
hpx_main
, in worker mode it will execute an emptyhpx_main
.- Return
- The function returns the value, which has been returned from
hpx_main
(or 0 when executed in worker mode). - Note
- If the parameter
mode
is not given (defaulted), the created runtime system instance will be executed in console or worker mode depending on the command line arguments passed inargc
/argv
. Otherwise it will be executed as specified by the parametermode
. - Parameters
desc_cmdline
: [in] This parameter may hold the description of additional command line arguments understood by the application. These options will be prepended to the default command line options understood by hpx::init (see description below).argc
: [in] The number of command line arguments passed inargv
. This is usually the unchanged value as passed by the operating system (tomain()
).argv
: [in] The command line arguments for this application, usually that is the value as passed by the operating system (tomain()
).cfg
: A list of configuration settings which will be added to the system configuration before the runtime instance is run. Each of the entries in this list must have the format of a fully defined key/value pair from an ini-file (for instance ‘hpx.component.enabled=1’)startup
: [in] A function to be executed inside a HPX thread beforef
is called. If this parameter is not given no function will be executed.shutdown
: [in] A function to be executed inside an HPX thread while hpx::finalize is executed. If this parameter is not given no function will be executed.mode
: [in] The mode the created runtime environment should be initialized in. There has to be exactly one locality in each HPX application which is executed in console mode (hpx::runtime_mode_console), all other localities have to be run in worker mode (hpx::runtime_mode_worker). Normally this is set up automatically, but sometimes it is necessary to explicitly specify the mode.
-
int
init
(int argc, char **argv, std::vector<std::string> const &cfg, hpx::runtime_mode mode = hpx::runtime_mode_default)¶ Main entry point for launching the HPX runtime system.
This is a simplified main entry point, which can be used to set up the runtime for an HPX application (the runtime system will be set up in console mode or worker mode depending on the command line settings).
In console mode it will execute the user supplied function
hpx_main
, in worker mode it will execute an emptyhpx_main
.- Return
- The function returns the value, which has been returned from
hpx_main
(or 0 when executed in worker mode). - Note
- The created runtime system instance will be executed in console or worker mode depending on the command line arguments passed in
argc
/argv
. - Parameters
argc
: [in] The number of command line arguments passed inargv
. This is usually the unchanged value as passed by the operating system (tomain()
).argv
: [in] The command line arguments for this application, usually that is the value as passed by the operating system (tomain()
).cfg
: A list of configuration settings which will be added to the system configuration before the runtime instance is run. Each of the entries in this list must have the format of a fully defined key/value pair from an ini-file (for instance ‘hpx.component.enabled=1’)mode
: [in] The mode the created runtime environment should be initialized in. There has to be exactly one locality in each HPX application which is executed in console mode (hpx::runtime_mode_console), all other localities have to be run in worker mode (hpx::runtime_mode_worker). Normally this is set up automatically, but sometimes it is necessary to explicitly specify the mode.
-
int
init
(boost::program_options::options_description const &desc_cmdline, int argc, char **argv, hpx::runtime_mode mode)¶ Main entry point for launching the HPX runtime system.
This is a simplified main entry point, which can be used to set up the runtime for an HPX application (the runtime system will be set up in console mode or worker mode depending on the command line settings).
In console mode it will execute the user supplied function
hpx_main
, in worker mode it will execute an emptyhpx_main
.- Return
- The function returns the value, which has been returned from
hpx_main
(or 0 when executed in worker mode). - Note
- If the parameter
mode
is runtime_mode_default, the created runtime system instance will be executed in console or worker mode depending on the command line arguments passed inargc
/argv
. Otherwise it will be executed as specified by the parametermode
. - Parameters
desc_cmdline
: [in] This parameter may hold the description of additional command line arguments understood by the application. These options will be prepended to the default command line options understood by hpx::init (see description below).argc
: [in] The number of command line arguments passed inargv
. This is usually the unchanged value as passed by the operating system (tomain()
).argv
: [in] The command line arguments for this application, usually that is the value as passed by the operating system (tomain()
).mode
: [in] The mode the created runtime environment should be initialized in. There has to be exactly one locality in each HPX application which is executed in console mode (hpx::runtime_mode_console), all other localities have to be run in worker mode (hpx::runtime_mode_worker). Normally this is set up automatically, but sometimes it is necessary to explicitly specify the mode.
-
int
init
(boost::program_options::options_description const &desc_cmdline, int argc, char **argv, std::vector<std::string> const &cfg, hpx::runtime_mode mode)¶ Main entry point for launching the HPX runtime system.
This is a simplified main entry point, which can be used to set up the runtime for an HPX application (the runtime system will be set up in console mode or worker mode depending on the command line settings).
In console mode it will execute the user supplied function
hpx_main
, in worker mode it will execute an emptyhpx_main
.- Return
- The function returns the value, which has been returned from
hpx_main
(or 0 when executed in worker mode). - Note
- If the parameter
mode
is runtime_mode_default, the created runtime system instance will be executed in console or worker mode depending on the command line arguments passed inargc
/argv
. Otherwise it will be executed as specified by the parametermode
. - Parameters
desc_cmdline
: [in] This parameter may hold the description of additional command line arguments understood by the application. These options will be prepended to the default command line options understood by hpx::init (see description below).argc
: [in] The number of command line arguments passed inargv
. This is usually the unchanged value as passed by the operating system (tomain()
).argv
: [in] The command line arguments for this application, usually that is the value as passed by the operating system (tomain()
).cfg
: A list of configuration settings which will be added to the system configuration before the runtime instance is run. Each of the entries in this list must have the format of a fully defined key/value pair from an ini-file (for instance ‘hpx.component.enabled=1’)mode
: [in] The mode the created runtime environment should be initialized in. There has to be exactly one locality in each HPX application which is executed in console mode (hpx::runtime_mode_console), all other localities have to be run in worker mode (hpx::runtime_mode_worker). Normally this is set up automatically, but sometimes it is necessary to explicitly specify the mode.
-
int
init
(std::string const &app_name, int argc = 0, char **argv = nullptr, hpx::runtime_mode mode = hpx::runtime_mode_default)¶ Main entry point for launching the HPX runtime system.
This is a simplified main entry point, which can be used to set up the runtime for an HPX application (the runtime system will be set up in console mode or worker mode depending on the command line settings).
- Return
- The function returns the value, which has been returned from
hpx_main
(or 0 when executed in worker mode). - Note
- The created runtime system instance will be executed in console or worker mode depending on the command line arguments passed in
argc
/argv
. - Parameters
app_name
: [in] The name of the application.argc
: [in] The number of command line arguments passed inargv
. This is usually the unchanged value as passed by the operating system (tomain()
).argv
: [in] The command line arguments for this application, usually that is the value as passed by the operating system (tomain()
).mode
: [in] The mode the created runtime environment should be initialized in. There has to be exactly one locality in each HPX application which is executed in console mode (hpx::runtime_mode_console), all other localities have to be run in worker mode (hpx::runtime_mode_worker). Normally this is set up automatically, but sometimes it is necessary to explicitly specify the mode.
-
int
init
(int argc = 0, char **argv = nullptr, hpx::runtime_mode mode = hpx::runtime_mode_default)¶ Main entry point for launching the HPX runtime system.
This is a simplified main entry point, which can be used to set up the runtime for an HPX application (the runtime system will be set up in console mode or worker mode depending on the command line settings).
- Return
- The function returns the value, which has been returned from
hpx_main
(or 0 when executed in worker mode). - Note
- The created runtime system instance will be executed in console or worker mode depending on the command line arguments passed in
argc
/argv
. If not command line arguments are passed, console mode is assumed. - Note
- If no command line arguments are passed the HPX runtime system will not support any of the default command line options as described in the section ‘HPX Command Line Options’.
- Parameters
argc
: [in] The number of command line arguments passed inargv
. This is usually the unchanged value as passed by the operating system (tomain()
).argv
: [in] The command line arguments for this application, usually that is the value as passed by the operating system (tomain()
).mode
: [in] The mode the created runtime environment should be initialized in. There has to be exactly one locality in each HPX application which is executed in console mode (hpx::runtime_mode_console), all other localities have to be run in worker mode (hpx::runtime_mode_worker). Normally this is set up automatically, but sometimes it is necessary to explicitly specify the mode.
-
int
init
(std::vector<std::string> const &cfg, hpx::runtime_mode mode = hpx::runtime_mode_default)¶ Main entry point for launching the HPX runtime system.
This is a simplified main entry point, which can be used to set up the runtime for an HPX application (the runtime system will be set up in console mode or worker mode depending on the command line settings).
- Return
- The function returns the value, which has been returned from
hpx_main
(or 0 when executed in worker mode). - Note
- The created runtime system instance will be executed in console or worker mode depending on the command line arguments passed in
argc
/argv
. If not command line arguments are passed, console mode is assumed. - Note
- If no command line arguments are passed the HPX runtime system will not support any of the default command line options as described in the section ‘HPX Command Line Options’.
- Parameters
cfg
: A list of configuration settings which will be added to the system configuration before the runtime instance is run. Each of the entries in this list must have the format of a fully defined key/value pair from an ini-file (for instance ‘hpx.component.enabled=1’)mode
: [in] The mode the created runtime environment should be initialized in. There has to be exactly one locality in each HPX application which is executed in console mode (hpx::runtime_mode_console), all other localities have to be run in worker mode (hpx::runtime_mode_worker). Normally this is set up automatically, but sometimes it is necessary to explicitly specify the mode.
-
int
init
(int (*f)(boost::program_options::variables_map &vm), std::string const &app_name, int argc, char **argv, hpx::runtime_mode mode = hpx::runtime_mode_default, )¶ Main entry point for launching the HPX runtime system.
This is a simplified main entry point, which can be used to set up the runtime for an HPX application (the runtime system will be set up in console mode or worker mode depending on the command line settings). It will schedule the function given by
f
as a HPX thread. This overload will not callhpx_main
.- Return
- The function returns the value, which has been returned from the user supplied function
f
. - Note
- The created runtime system instance will be executed in console or worker mode depending on the command line arguments passed in
argc
/argv
. - Parameters
f
: [in] The function to be scheduled as an HPX thread. Usually this function represents the main entry point of any HPX application. Iff
isnullptr
the HPX runtime environment will be started without invokingf
.app_name
: [in] The name of the application.argc
: [in] The number of command line arguments passed inargv
. This is usually the unchanged value as passed by the operating system (tomain()
).argv
: [in] The command line arguments for this application, usually that is the value as passed by the operating system (tomain()
).mode
: [in] The mode the created runtime environment should be initialized in. There has to be exactly one locality in each HPX application which is executed in console mode (hpx::runtime_mode_console), all other localities have to be run in worker mode (hpx::runtime_mode_worker). Normally this is set up automatically, but sometimes it is necessary to explicitly specify the mode.
-
int
init
(int (*f)(boost::program_options::variables_map &vm), int argc, char **argv, hpx::runtime_mode mode = hpx::runtime_mode_default, )¶ Main entry point for launching the HPX runtime system.
This is a simplified main entry point, which can be used to set up the runtime for an HPX application (the runtime system will be set up in console mode or worker mode depending on the command line settings). It will schedule the function given by
f
as a HPX thread. This overload will not callhpx_main
.- Return
- The function returns the value, which has been returned from the user supplied function
f
. - Note
- The created runtime system instance will be executed in console or worker mode depending on the command line arguments passed in
argc
/argv
. - Parameters
f
: [in] The function to be scheduled as an HPX thread. Usually this function represents the main entry point of any HPX application. Iff
isnullptr
the HPX runtime environment will be started without invokingf
.argc
: [in] The number of command line arguments passed inargv
. This is usually the unchanged value as passed by the operating system (tomain()
).argv
: [in] The command line arguments for this application, usually that is the value as passed by the operating system (tomain()
).mode
: [in] The mode the created runtime environment should be initialized in. There has to be exactly one locality in each HPX application which is executed in console mode (hpx::runtime_mode_console), all other localities have to be run in worker mode (hpx::runtime_mode_worker). Normally this is set up automatically, but sometimes it is necessary to explicitly specify the mode.
-
int
init
(util::function_nonser<int(int, char **)> const &f, std::string const &app_name, int argc, char **argv, hpx::runtime_mode mode = hpx::runtime_mode_default, )¶ Main entry point for launching the HPX runtime system.
This is a simplified main entry point, which can be used to set up the runtime for an HPX application (the runtime system will be set up in console mode or worker mode depending on the command line settings). It will schedule the function given by
f
as a HPX thread. This overload will not callhpx_main
.- Return
- The function returns the value, which has been returned from the user supplied function
f
. - Note
- The created runtime system instance will be executed in console or worker mode depending on the command line arguments passed in
argc
/argv
. - Parameters
f
: [in] The function to be scheduled as an HPX thread. Usually this function represents the main entry point of any HPX application. Iff
isnullptr
the HPX runtime environment will be started without invokingf
.app_name
: [in] The name of the application.argc
: [in] The number of command line arguments passed inargv
. This is usually the unchanged value as passed by the operating system (tomain()
).argv
: [in] The command line arguments for this application, usually that is the value as passed by the operating system (tomain()
).mode
: [in] The mode the created runtime environment should be initialized in. There has to be exactly one locality in each HPX application which is executed in console mode (hpx::runtime_mode_console), all other localities have to be run in worker mode (hpx::runtime_mode_worker). Normally this is set up automatically, but sometimes it is necessary to explicitly specify the mode.
-
int
init
(util::function_nonser<int(int, char **)> const &f, int argc, char **argv, hpx::runtime_mode mode = hpx::runtime_mode_default, )¶ Main entry point for launching the HPX runtime system.
This is a simplified main entry point, which can be used to set up the runtime for an HPX application (the runtime system will be set up in console mode or worker mode depending on the command line settings). It will schedule the function given by
f
as a HPX thread. This overload will not callhpx_main
.- Return
- The function returns the value, which has been returned from the user supplied function
f
. - Note
- The created runtime system instance will be executed in console or worker mode depending on the command line arguments passed in
argc
/argv
. - Parameters
f
: [in] The function to be scheduled as an HPX thread. Usually this function represents the main entry point of any HPX application. Iff
isnullptr
the HPX runtime environment will be started without invokingf
.argc
: [in] The number of command line arguments passed inargv
. This is usually the unchanged value as passed by the operating system (tomain()
).argv
: [in] The command line arguments for this application, usually that is the value as passed by the operating system (tomain()
).mode
: [in] The mode the created runtime environment should be initialized in. There has to be exactly one locality in each HPX application which is executed in console mode (hpx::runtime_mode_console), all other localities have to be run in worker mode (hpx::runtime_mode_worker). Normally this is set up automatically, but sometimes it is necessary to explicitly specify the mode.
-
int
init
(util::function_nonser<int(int, char **)> const &f, int argc, char **argv, std::vector<std::string> const &cfg, hpx::runtime_mode mode = hpx::runtime_mode_default, )¶ Main entry point for launching the HPX runtime system.
This is a simplified main entry point, which can be used to set up the runtime for an HPX application (the runtime system will be set up in console mode or worker mode depending on the command line settings). It will schedule the function given by
f
as a HPX thread. This overload will not callhpx_main
.- Return
- The function returns the value, which has been returned from the user supplied function
f
. - Note
- The created runtime system instance will be executed in console or worker mode depending on the command line arguments passed in
argc
/argv
. - Parameters
f
: [in] The function to be scheduled as an HPX thread. Usually this function represents the main entry point of any HPX application. Iff
isnullptr
the HPX runtime environment will be started without invokingf
.argc
: [in] The number of command line arguments passed inargv
. This is usually the unchanged value as passed by the operating system (tomain()
).argv
: [in] The command line arguments for this application, usually that is the value as passed by the operating system (tomain()
).cfg
: A list of configuration settings which will be added to the system configuration before the runtime instance is run. Each of the entries in this list must have the format of a fully defined key/value pair from an ini-file (for instance ‘hpx.component.enabled=1’)mode
: [in] The mode the created runtime environment should be initialized in. There has to be exactly one locality in each HPX application which is executed in console mode (hpx::runtime_mode_console), all other localities have to be run in worker mode (hpx::runtime_mode_worker). Normally this is set up automatically, but sometimes it is necessary to explicitly specify the mode.
-
bool
start
(util::function_nonser<int(boost::program_options::variables_map &vm)> const &f, boost::program_options::options_description const &desc_cmdline, int argc, char **argv, std::vector<std::string> const &cfg, startup_function_type startup = startup_function_type(), shutdown_function_type shutdown = shutdown_function_type(), hpx::runtime_mode mode = hpx::runtime_mode_default, )¶ Main non-blocking entry point for launching the HPX runtime system.
This is the main, non-blocking entry point for any HPX application. This function (or one of its overloads below) should be called from the users
main()
function. It will set up the HPX runtime environment and schedule the function given byf
as a HPX thread. It will return immediately after that. Usehpx::wait
andhpx::stop
to synchronize with the runtime system’s execution. This overload will not callhpx_main
.- Return
- The function returns true if command line processing succeeded and the runtime system was started successfully. It will return false otherwise.
- Note
- If the parameter
mode
is not given (defaulted), the created runtime system instance will be executed in console or worker mode depending on the command line arguments passed inargc
/argv
. Otherwise it will be executed as specified by the parametermode
. - Parameters
f
: [in] The function to be scheduled as an HPX thread. Usually this function represents the main entry point of any HPX application. Iff
isnullptr
the HPX runtime environment will be started without invokingf
.desc_cmdline
: [in] This parameter may hold the description of additional command line arguments understood by the application. These options will be prepended to the default command line options understood by hpx::init (see description below).argc
: [in] The number of command line arguments passed inargv
. This is usually the unchanged value as passed by the operating system (tomain()
).argv
: [in] The command line arguments for this application, usually that is the value as passed by the operating system (tomain()
).cfg
: A list of configuration settings which will be added to the system configuration before the runtime instance is run. Each of the entries in this list must have the format of a fully defined key/value pair from an ini-file (for instance ‘hpx.component.enabled=1’)startup
: [in] A function to be executed inside a HPX thread beforef
is called. If this parameter is not given no function will be executed.shutdown
: [in] A function to be executed inside an HPX thread while hpx::finalize is executed. If this parameter is not given no function will be executed.mode
: [in] The mode the created runtime environment should be initialized in. There has to be exactly one locality in each HPX application which is executed in console mode (hpx::runtime_mode_console), all other localities have to be run in worker mode (hpx::runtime_mode_worker). Normally this is set up automatically, but sometimes it is necessary to explicitly specify the mode.
-
bool
start
(int (*f)(boost::program_options::variables_map &vm), boost::program_options::options_description const &desc_cmdline, int argc, char **argv, startup_function_type startup = startup_function_type(), shutdown_function_type shutdown = shutdown_function_type(), hpx::runtime_mode mode = hpx::runtime_mode_default, )¶ Main non-blocking entry point for launching the HPX runtime system.
This is the main, non-blocking entry point for any HPX application. This function (or one of its overloads below) should be called from the users
main()
function. It will set up the HPX runtime environment and schedule the function given byf
as a HPX thread. It will return immediately after that. Usehpx::wait
andhpx::stop
to synchronize with the runtime system’s execution. This overload will not callhpx_main
.- Return
- The function returns true if command line processing succeeded and the runtime system was started successfully. It will return false otherwise.
- Note
- If the parameter
mode
is not given (defaulted), the created runtime system instance will be executed in console or worker mode depending on the command line arguments passed inargc
/argv
. Otherwise it will be executed as specified by the parametermode
. - Parameters
f
: [in] The function to be scheduled as an HPX thread. Usually this function represents the main entry point of any HPX application. Iff
isnullptr
the HPX runtime environment will be started without invokingf
.desc_cmdline
: [in] This parameter may hold the description of additional command line arguments understood by the application. These options will be prepended to the default command line options understood by hpx::init (see description below).argc
: [in] The number of command line arguments passed inargv
. This is usually the unchanged value as passed by the operating system (tomain()
).argv
: [in] The command line arguments for this application, usually that is the value as passed by the operating system (tomain()
).startup
: [in] A function to be executed inside a HPX thread beforef
is called. If this parameter is not given no function will be executed.shutdown
: [in] A function to be executed inside an HPX thread while hpx::finalize is executed. If this parameter is not given no function will be executed.mode
: [in] The mode the created runtime environment should be initialized in. There has to be exactly one locality in each HPX application which is executed in console mode (hpx::runtime_mode_console), all other localities have to be run in worker mode (hpx::runtime_mode_worker). Normally this is set up automatically, but sometimes it is necessary to explicitly specify the mode.
-
bool
start
(boost::program_options::options_description const &desc_cmdline, int argc, char **argv, startup_function_type startup = startup_function_type(), shutdown_function_type shutdown = shutdown_function_type(), hpx::runtime_mode mode = hpx::runtime_mode_default)¶ Main non-blocking entry point for launching the HPX runtime system.
This is a simplified main, non-blocking entry point, which can be used to set up the runtime for an HPX application (the runtime system will be set up in console mode or worker mode depending on the command line settings). It will return immediately after that. Use
hpx::wait
andhpx::stop
to synchronize with the runtime system’s execution.In console mode it will execute the user supplied function
hpx_main
, in worker mode it will execute an emptyhpx_main
.- Return
- The function returns true if command line processing succeeded and the runtime system was started successfully. It will return false otherwise.
- Note
- If the parameter
mode
is not given (defaulted), the created runtime system instance will be executed in console or worker mode depending on the command line arguments passed inargc
/argv
. Otherwise it will be executed as specified by the parametermode
. - Parameters
desc_cmdline
: [in] This parameter may hold the description of additional command line arguments understood by the application. These options will be prepended to the default command line options understood by hpx::init (see description below).argc
: [in] The number of command line arguments passed inargv
. This is usually the unchanged value as passed by the operating system (tomain()
).argv
: [in] The command line arguments for this application, usually that is the value as passed by the operating system (tomain()
).startup
: [in] A function to be executed inside a HPX thread beforef
is called. If this parameter is not given no function will be executed.shutdown
: [in] A function to be executed inside an HPX thread while hpx::finalize is executed. If this parameter is not given no function will be executed.mode
: [in] The mode the created runtime environment should be initialized in. There has to be exactly one locality in each HPX application which is executed in console mode (hpx::runtime_mode_console), all other localities have to be run in worker mode (hpx::runtime_mode_worker). Normally this is set up automatically, but sometimes it is necessary to explicitly specify the mode.
-
bool
start
(boost::program_options::options_description const &desc_cmdline, int argc, char **argv, std::vector<std::string> const &cfg, startup_function_type startup = startup_function_type(), shutdown_function_type shutdown = shutdown_function_type(), hpx::runtime_mode mode = hpx::runtime_mode_default)¶ Main non-blocking entry point for launching the HPX runtime system.
This is a simplified main, non-blocking entry point, which can be used to set up the runtime for an HPX application (the runtime system will be set up in console mode or worker mode depending on the command line settings). It will return immediately after that. Use
hpx::wait
andhpx::stop
to synchronize with the runtime system’s execution.In console mode it will execute the user supplied function
hpx_main
, in worker mode it will execute an emptyhpx_main
.- Return
- The function returns true if command line processing succeeded and the runtime system was started successfully. It will return false otherwise.
- Note
- If the parameter
mode
is not given (defaulted), the created runtime system instance will be executed in console or worker mode depending on the command line arguments passed inargc
/argv
. Otherwise it will be executed as specified by the parametermode
. - Parameters
desc_cmdline
: [in] This parameter may hold the description of additional command line arguments understood by the application. These options will be prepended to the default command line options understood by hpx::init (see description below).argc
: [in] The number of command line arguments passed inargv
. This is usually the unchanged value as passed by the operating system (tomain()
).argv
: [in] The command line arguments for this application, usually that is the value as passed by the operating system (tomain()
).cfg
: A list of configuration settings which will be added to the system configuration before the runtime instance is run. Each of the entries in this list must have the format of a fully defined key/value pair from an ini-file (for instance ‘hpx.component.enabled=1’)startup
: [in] A function to be executed inside a HPX thread beforef
is called. If this parameter is not given no function will be executed.shutdown
: [in] A function to be executed inside an HPX thread while hpx::finalize is executed. If this parameter is not given no function will be executed.mode
: [in] The mode the created runtime environment should be initialized in. There has to be exactly one locality in each HPX application which is executed in console mode (hpx::runtime_mode_console), all other localities have to be run in worker mode (hpx::runtime_mode_worker). Normally this is set up automatically, but sometimes it is necessary to explicitly specify the mode.
-
bool
start
(int argc, char **argv, std::vector<std::string> const &cfg, hpx::runtime_mode mode = hpx::runtime_mode_default)¶ Main non-blocking entry point for launching the HPX runtime system.
This is a simplified main, non-blocking entry point, which can be used to set up the runtime for an HPX application (the runtime system will be set up in console mode or worker mode depending on the command line settings). It will return immediately after that. Use
hpx::wait
andhpx::stop
to synchronize with the runtime system’s execution.In console mode it will execute the user supplied function
hpx_main
, in worker mode it will execute an emptyhpx_main
.- Return
- The function returns true if command line processing succeeded and the runtime system was started successfully. It will return false otherwise.
- Note
- If the parameter
mode
is runtime_mode_default, the created runtime system instance will be executed in console or worker mode depending on the command line arguments passed inargc
/argv
. Otherwise it will be executed as specified by the parametermode
. - Parameters
argc
: [in] The number of command line arguments passed inargv
. This is usually the unchanged value as passed by the operating system (tomain()
).argv
: [in] The command line arguments for this application, usually that is the value as passed by the operating system (tomain()
).cfg
: A list of configuration settings which will be added to the system configuration before the runtime instance is run. Each of the entries in this list must have the format of a fully defined key/value pair from an ini-file (for instance ‘hpx.component.enabled=1’)mode
: [in] The mode the created runtime environment should be initialized in. There has to be exactly one locality in each HPX application which is executed in console mode (hpx::runtime_mode_console), all other localities have to be run in worker mode (hpx::runtime_mode_worker). Normally this is set up automatically, but sometimes it is necessary to explicitly specify the mode.
-
bool
start
(boost::program_options::options_description const &desc_cmdline, int argc, char **argv, hpx::runtime_mode mode)¶ Main non-blocking entry point for launching the HPX runtime system.
This is a simplified main, non-blocking entry point, which can be used to set up the runtime for an HPX application (the runtime system will be set up in console mode or worker mode depending on the command line settings). It will return immediately after that. Use
hpx::wait
andhpx::stop
to synchronize with the runtime system’s execution.In console mode it will execute the user supplied function
hpx_main
, in worker mode it will execute an emptyhpx_main
.- Return
- The function returns true if command line processing succeeded and the runtime system was started successfully. It will return false otherwise.
- Note
- If the parameter
mode
is runtime_mode_default, the created runtime system instance will be executed in console or worker mode depending on the command line arguments passed inargc
/argv
. Otherwise it will be executed as specified by the parametermode
. - Parameters
desc_cmdline
: [in] This parameter may hold the description of additional command line arguments understood by the application. These options will be prepended to the default command line options understood by hpx::init (see description below).argc
: [in] The number of command line arguments passed inargv
. This is usually the unchanged value as passed by the operating system (tomain()
).argv
: [in] The command line arguments for this application, usually that is the value as passed by the operating system (tomain()
).mode
: [in] The mode the created runtime environment should be initialized in. There has to be exactly one locality in each HPX application which is executed in console mode (hpx::runtime_mode_console), all other localities have to be run in worker mode (hpx::runtime_mode_worker). Normally this is set up automatically, but sometimes it is necessary to explicitly specify the mode.
-
bool
start
(boost::program_options::options_description const &desc_cmdline, int argc, char **argv, std::vector<std::string> const &cfg, hpx::runtime_mode mode)¶ Main non-blocking entry point for launching the HPX runtime system.
This is a simplified main, non-blocking entry point, which can be used to set up the runtime for an HPX application (the runtime system will be set up in console mode or worker mode depending on the command line settings). It will return immediately after that. Use
hpx::wait
andhpx::stop
to synchronize with the runtime system’s execution.In console mode it will execute the user supplied function
hpx_main
, in worker mode it will execute an emptyhpx_main
.- Return
- The function returns true if command line processing succeeded and the runtime system was started successfully. It will return false otherwise.
- Note
- If the parameter
mode
is runtime_mode_default, the created runtime system instance will be executed in console or worker mode depending on the command line arguments passed inargc
/argv
. Otherwise it will be executed as specified by the parametermode
. - Parameters
desc_cmdline
: [in] This parameter may hold the description of additional command line arguments understood by the application. These options will be prepended to the default command line options understood by hpx::init (see description below).argc
: [in] The number of command line arguments passed inargv
. This is usually the unchanged value as passed by the operating system (tomain()
).argv
: [in] The command line arguments for this application, usually that is the value as passed by the operating system (tomain()
).cfg
: A list of configuration settings which will be added to the system configuration before the runtime instance is run. Each of the entries in this list must have the format of a fully defined key/value pair from an ini-file (for instance ‘hpx.component.enabled=1’)mode
: [in] The mode the created runtime environment should be initialized in. There has to be exactly one locality in each HPX application which is executed in console mode (hpx::runtime_mode_console), all other localities have to be run in worker mode (hpx::runtime_mode_worker). Normally this is set up automatically, but sometimes it is necessary to explicitly specify the mode.
-
bool
start
(std::string const &app_name, int argc = 0, char **argv = nullptr, hpx::runtime_mode mode = hpx::runtime_mode_default)¶ Main non-blocking entry point for launching the HPX runtime system.
This is a simplified main, non-blocking entry point, which can be used to set up the runtime for an HPX application (the runtime system will be set up in console mode or worker mode depending on the command line settings). It will return immediately after that. Use
hpx::wait
andhpx::stop
to synchronize with the runtime system’s execution.- Return
- The function returns true if command line processing succeeded and the runtime system was started successfully. It will return false otherwise.
- Note
- The created runtime system instance will be executed in console or worker mode depending on the command line arguments passed in
argc
/argv
. - Parameters
app_name
: [in] The name of the application.argc
: [in] The number of command line arguments passed inargv
. This is usually the unchanged value as passed by the operating system (tomain()
).argv
: [in] The command line arguments for this application, usually that is the value as passed by the operating system (tomain()
).mode
: [in] The mode the created runtime environment should be initialized in. There has to be exactly one locality in each HPX application which is executed in console mode (hpx::runtime_mode_console), all other localities have to be run in worker mode (hpx::runtime_mode_worker). Normally this is set up automatically, but sometimes it is necessary to explicitly specify the mode.
-
bool
start
(int argc = 0, char **argv = nullptr, hpx::runtime_mode mode = hpx::runtime_mode_default)¶ Main non-blocking entry point for launching the HPX runtime system.
This is a simplified main, non-blocking entry point, which can be used to set up the runtime for an HPX application (the runtime system will be set up in console mode or worker mode depending on the command line settings). It will return immediately after that. Use
hpx::wait
andhpx::stop
to synchronize with the runtime system’s execution.- Return
- The function returns true if command line processing succeeded and the runtime system was started successfully. It will return false otherwise.
- Note
- The created runtime system instance will be executed in console or worker mode depending on the command line arguments passed in
argc
/argv
. If not command line arguments are passed, console mode is assumed. - Note
- If no command line arguments are passed the HPX runtime system will not support any of the default command line options as described in the section ‘HPX Command Line Options’.
- Parameters
argc
: [in] The number of command line arguments passed inargv
. This is usually the unchanged value as passed by the operating system (tomain()
).argv
: [in] The command line arguments for this application, usually that is the value as passed by the operating system (tomain()
).mode
: [in] The mode the created runtime environment should be initialized in. There has to be exactly one locality in each HPX application which is executed in console mode (hpx::runtime_mode_console), all other localities have to be run in worker mode (hpx::runtime_mode_worker). Normally this is set up automatically, but sometimes it is necessary to explicitly specify the mode.
-
bool
start
(std::vector<std::string> const &cfg, hpx::runtime_mode mode = hpx::runtime_mode_default)¶ Main non-blocking entry point for launching the HPX runtime system.
This is a simplified main, non-blocking entry point, which can be used to set up the runtime for an HPX application (the runtime system will be set up in console mode or worker mode depending on the command line settings). It will return immediately after that. Use
hpx::wait
andhpx::stop
to synchronize with the runtime system’s execution.- Return
- The function returns true if command line processing succeeded and the runtime system was started successfully. It will return false otherwise.
- Note
- The created runtime system instance will be executed in console or worker mode depending on the command line arguments passed in
argc
/argv
. If not command line arguments are passed, console mode is assumed. - Note
- If no command line arguments are passed the HPX runtime system will not support any of the default command line options as described in the section ‘HPX Command Line Options’.
- Parameters
cfg
: A list of configuration settings which will be added to the system configuration before the runtime instance is run. Each of the entries in this list must have the format of a fully defined key/value pair from an ini-file (for instance ‘hpx.component.enabled=1’)mode
: [in] The mode the created runtime environment should be initialized in. There has to be exactly one locality in each HPX application which is executed in console mode (hpx::runtime_mode_console), all other localities have to be run in worker mode (hpx::runtime_mode_worker). Normally this is set up automatically, but sometimes it is necessary to explicitly specify the mode.
-
bool
start
(int (*f)(boost::program_options::variables_map &vm), std::string const &app_name, int argc, char **argv, hpx::runtime_mode mode = hpx::runtime_mode_default, )¶ Main non-blocking entry point for launching the HPX runtime system.
This is a simplified main, non-blocking entry point, which can be used to set up the runtime for an HPX application (the runtime system will be set up in console mode or worker mode depending on the command line settings). It will return immediately after that. Use
hpx::wait
andhpx::stop
to synchronize with the runtime system’s execution. This overload will schedule the function given byf
as a HPX thread. It will not callhpx_main
.- Return
- The function returns true if command line processing succeeded and the runtime system was started successfully. It will return false otherwise.
- Note
- The created runtime system instance will be executed in console or worker mode depending on the command line arguments passed in
argc
/argv
. - Parameters
f
: [in] The function to be scheduled as an HPX thread. Usually this function represents the main entry point of any HPX application.app_name
: [in] The name of the application.argc
: [in] The number of command line arguments passed inargv
. This is usually the unchanged value as passed by the operating system (tomain()
).argv
: [in] The command line arguments for this application, usually that is the value as passed by the operating system (tomain()
).mode
: [in] The mode the created runtime environment should be initialized in. There has to be exactly one locality in each HPX application which is executed in console mode (hpx::runtime_mode_console), all other localities have to be run in worker mode (hpx::runtime_mode_worker). Normally this is set up automatically, but sometimes it is necessary to explicitly specify the mode.
-
bool
start
(util::function_nonser<int(int, char **)> const &f, std::string const &app_name, int argc, char **argv, hpx::runtime_mode mode = hpx::runtime_mode_default, )¶ Main non-blocking entry point for launching the HPX runtime system.
This is a simplified main, non-blocking entry point, which can be used to set up the runtime for an HPX application (the runtime system will be set up in console mode or worker mode depending on the command line settings). It will return immediately after that. Use
hpx::wait
andhpx::stop
to synchronize with the runtime system’s execution. This overload will schedule the function given byf
as a HPX thread. It will not callhpx_main
.- Return
- The function returns true if command line processing succeeded and the runtime system was started successfully. It will return false otherwise.
- Note
- The created runtime system instance will be executed in console or worker mode depending on the command line arguments passed in
argc
/argv
. - Parameters
f
: [in] The function to be scheduled as an HPX thread. Usually this function represents the main entry point of any HPX application. Iff
isnullptr
the HPX runtime environment will be started without invokingf
.app_name
: [in] The name of the application.argc
: [in] The number of command line arguments passed inargv
. This is usually the unchanged value as passed by the operating system (tomain()
).argv
: [in] The command line arguments for this application, usually that is the value as passed by the operating system (tomain()
).mode
: [in] The mode the created runtime environment should be initialized in. There has to be exactly one locality in each HPX application which is executed in console mode (hpx::runtime_mode_console), all other localities have to be run in worker mode (hpx::runtime_mode_worker). Normally this is set up automatically, but sometimes it is necessary to explicitly specify the mode.
-
bool
start
(int (*f)(boost::program_options::variables_map &vm), int argc, char **argv, hpx::runtime_mode mode = hpx::runtime_mode_default, )¶ Main non-blocking entry point for launching the HPX runtime system.
This is a simplified main, non-blocking entry point, which can be used to set up the runtime for an HPX application (the runtime system will be set up in console mode or worker mode depending on the command line settings). It will return immediately after that. Use
hpx::wait
andhpx::stop
to synchronize with the runtime system’s execution. This overload will schedule the function given byf
as a HPX thread. It will not callhpx_main
.- Return
- The function returns true if command line processing succeeded and the runtime system was started successfully. It will return false otherwise.
- Note
- The created runtime system instance will be executed in console or worker mode depending on the command line arguments passed in
argc
/argv
. - Parameters
f
: [in] The function to be scheduled as an HPX thread. Usually this function represents the main entry point of any HPX application. Iff
isnullptr
the HPX runtime environment will be started without invokingf
.argc
: [in] The number of command line arguments passed inargv
. This is usually the unchanged value as passed by the operating system (tomain()
).argv
: [in] The command line arguments for this application, usually that is the value as passed by the operating system (tomain()
).mode
: [in] The mode the created runtime environment should be initialized in. There has to be exactly one locality in each HPX application which is executed in console mode (hpx::runtime_mode_console), all other localities have to be run in worker mode (hpx::runtime_mode_worker). Normally this is set up automatically, but sometimes it is necessary to explicitly specify the mode.
-
bool
start
(util::function_nonser<int(int, char **)> const &f, int argc, char **argv, hpx::runtime_mode mode = hpx::runtime_mode_default, )¶ Main non-blocking entry point for launching the HPX runtime system.
This is a simplified main, non-blocking entry point, which can be used to set up the runtime for an HPX application (the runtime system will be set up in console mode or worker mode depending on the command line settings). It will return immediately after that. Use
hpx::wait
andhpx::stop
to synchronize with the runtime system’s execution. This overload will schedule the function given byf
as a HPX thread. It will not callhpx_main
.- Return
- The function returns true if command line processing succeeded and the runtime system was started successfully. It will return false otherwise.
- Note
- The created runtime system instance will be executed in console or worker mode depending on the command line arguments passed in
argc
/argv
. - Parameters
f
: [in] The function to be scheduled as an HPX thread. Usually this function represents the main entry point of any HPX application. Iff
isnullptr
the HPX runtime environment will be started without invokingf
.argc
: [in] The number of command line arguments passed inargv
. This is usually the unchanged value as passed by the operating system (tomain()
).argv
: [in] The command line arguments for this application, usually that is the value as passed by the operating system (tomain()
).mode
: [in] The mode the created runtime environment should be initialized in. There has to be exactly one locality in each HPX application which is executed in console mode (hpx::runtime_mode_console), all other localities have to be run in worker mode (hpx::runtime_mode_worker). Normally this is set up automatically, but sometimes it is necessary to explicitly specify the mode.
-
bool
start
(util::function_nonser<int(int, char **)> const &f, int argc, char **argv, std::vector<std::string> const &cfg, hpx::runtime_mode mode = hpx::runtime_mode_default, )¶ Main non-blocking entry point for launching the HPX runtime system.
This is a simplified main, non-blocking entry point, which can be used to set up the runtime for an HPX application (the runtime system will be set up in console mode or worker mode depending on the command line settings). It will return immediately after that. Use
hpx::wait
andhpx::stop
to synchronize with the runtime system’s execution. This overload will schedule the function given byf
as a HPX thread. It will not callhpx_main
.- Return
- The function returns true if command line processing succeeded and the runtime system was started successfully. It will return false otherwise.
- Note
- The created runtime system instance will be executed in console or worker mode depending on the command line arguments passed in
argc
/argv
. - Parameters
f
: [in] The function to be scheduled as an HPX thread. Usually this function represents the main entry point of any HPX application. Iff
isnullptr
the HPX runtime environment will be started without invokingf
.argc
: [in] The number of command line arguments passed inargv
. This is usually the unchanged value as passed by the operating system (tomain()
).argv
: [in] The command line arguments for this application, usually that is the value as passed by the operating system (tomain()
).cfg
: A list of configuration settings which will be added to the system configuration before the runtime instance is run. Each of the entries in this list must have the format of a fully defined key/value pair from an ini-file (for instance ‘hpx.component.enabled=1’)mode
: [in] The mode the created runtime environment should be initialized in. There has to be exactly one locality in each HPX application which is executed in console mode (hpx::runtime_mode_console), all other localities have to be run in worker mode (hpx::runtime_mode_worker). Normally this is set up automatically, but sometimes it is necessary to explicitly specify the mode.
-
int
finalize
(double shutdown_timeout, double localwait = -1.0, error_code &ec = throws)¶ Main function to gracefully terminate the HPX runtime system.
The function hpx::finalize is the main way to (gracefully) exit any HPX application. It should be called from one locality only (usually the console) and it will notify all connected localities to finish execution. Only after all other localities have exited this function will return, allowing to exit the console locality as well.
During the execution of this function the runtime system will invoke all registered shutdown functions (see hpx::init) on all localities.
The default value (
-1.0
) will try to find a globally set timeout value (can be set as the configuration parameterhpx.shutdown_timeout
), and if that is not set or-1.0
as well, it will disable any timeout, each connected locality will wait for all existing HPX-threads to terminate.- Parameters
shutdown_timeout
: This parameter allows to specify a timeout (in microseconds), specifying how long any of the connected localities should wait for pending tasks to be executed. After this timeout, all suspended HPX-threads will be aborted. Note, that this function will not abort any running HPX-threads. In any case the shutdown will not proceed as long as there is at least one pending/running HPX-thread.
The default value (
-1.0
) will try to find a globally set wait time value (can be set as the configuration parameter “hpx.finalize_wait_time”), and if this is not set or-1.0
as well, it will disable any addition local wait time before proceeding.- Parameters
localwait
: This parameter allows to specify a local wait time (in microseconds) before the connected localities will be notified and the overall shutdown process starts.
This function will block and wait for all connected localities to exit before returning to the caller. It should be the last HPX-function called by any application.
- Return
- This function will always return zero.
- Note
- As long as ec is not pre-initialized to hpx::throws this function doesn’t throw but returns the result code using the parameter ec. Otherwise it throws an instance of hpx::exception.
- Parameters
ec
: [in,out] this represents the error status on exit, if this is pre-initialized to hpx::throws the function will throw on error instead.
Using this function is an alternative to hpx::disconnect, these functions do not need to be called both.
-
int
finalize
(error_code &ec = throws)¶ Main function to gracefully terminate the HPX runtime system.
The function hpx::finalize is the main way to (gracefully) exit any HPX application. It should be called from one locality only (usually the console) and it will notify all connected localities to finish execution. Only after all other localities have exited this function will return, allowing to exit the console locality as well.
During the execution of this function the runtime system will invoke all registered shutdown functions (see hpx::init) on all localities.
This function will block and wait for all connected localities to exit before returning to the caller. It should be the last HPX-function called by any application.
- Return
- This function will always return zero.
- Note
- As long as ec is not pre-initialized to hpx::throws this function doesn’t throw but returns the result code using the parameter ec. Otherwise it throws an instance of hpx::exception.
- Parameters
ec
: [in,out] this represents the error status on exit, if this is pre-initialized to hpx::throws the function will throw on error instead.
Using this function is an alternative to hpx::disconnect, these functions do not need to be called both.
-
HPX_NORETURN void hpx::terminate()
Terminate any application non-gracefully.
The function hpx::terminate is the non-graceful way to exit any application immediately. It can be called from any locality and will terminate all localities currently used by the application.
- Note
- This function will cause HPX to call
std::terminate()
on all localities associated with this application. If the function is called not from an HPX thread it will fail and return an error using the argument ec.
-
int
disconnect
(double shutdown_timeout, double localwait = -1.0, error_code &ec = throws)¶ Disconnect this locality from the application.
The function hpx::disconnect can be used to disconnect a locality from a running HPX application.
During the execution of this function the runtime system will invoke all registered shutdown functions (see hpx::init) on this locality.
The default value (
-1.0
) will try to find a globally set timeout value (can be set as the configuration parameter “hpx.shutdown_timeout”), and if that is not set or-1.0
as well, it will disable any timeout, each connected locality will wait for all existing HPX-threads to terminate.- Parameters
shutdown_timeout
: This parameter allows to specify a timeout (in microseconds), specifying how long this locality should wait for pending tasks to be executed. After this timeout, all suspended HPX-threads will be aborted. Note, that this function will not abort any running HPX-threads. In any case the shutdown will not proceed as long as there is at least one pending/running HPX-thread.
The default value (
-1.0
) will try to find a globally set wait time value (can be set as the configuration parameterhpx.finalize_wait_time
), and if this is not set or-1.0
as well, it will disable any addition local wait time before proceeding.- Parameters
localwait
: This parameter allows to specify a local wait time (in microseconds) before the connected localities will be notified and the overall shutdown process starts.
This function will block and wait for this locality to finish executing before returning to the caller. It should be the last HPX-function called by any locality being disconnected.
- Return
- This function will always return zero.
- Note
- As long as ec is not pre-initialized to hpx::throws this function doesn’t throw but returns the result code using the parameter ec. Otherwise it throws an instance of hpx::exception.
- Parameters
ec
: [in,out] this represents the error status on exit, if this is pre-initialized to hpx::throws the function will throw on error instead.
-
int
disconnect
(error_code &ec = throws)¶ Disconnect this locality from the application.
The function hpx::disconnect can be used to disconnect a locality from a running HPX application.
During the execution of this function the runtime system will invoke all registered shutdown functions (see hpx::init) on this locality.
This function will block and wait for this locality to finish executing before returning to the caller. It should be the last HPX-function called by any locality being disconnected.
- Return
- This function will always return zero.
- Note
- As long as ec is not pre-initialized to hpx::throws this function doesn’t throw but returns the result code using the parameter ec. Otherwise it throws an instance of hpx::exception.
- Parameters
ec
: [in,out] this represents the error status on exit, if this is pre-initialized to hpx::throws the function will throw on error instead.
-
int
stop
(error_code &ec = throws)¶ Stop the runtime system.
This function will block and wait for this locality to finish executing before returning to the caller. It should be the last HPX-function called on every locality. This function should be used only if the runtime system was started using
hpx::start
.- Return
- The function returns the value, which has been returned from the user supplied main HPX function (usually
hpx_main
).
-
int
suspend
(error_code &ec = throws)¶ Suspend the runtime system.
The function hpx::suspend is used to suspend the HPX runtime system. It can only be used when running HPX on a single locality. It will block waiting for all thread pools to be empty. This function only be called when the runtime is running, or already suspended in which case this function will do nothing.
- Return
- This function will always return zero.
- Note
- As long as ec is not pre-initialized to hpx::throws this function doesn’t throw but returns the result code using the parameter ec. Otherwise it throws an instance of hpx::exception.
- Parameters
ec
: [in,out] this represents the error status on exit, if this is pre-initialized to hpx::throws the function will throw on error instead.
-
int
resume
(error_code &ec = throws)¶ Resume the HPX runtime system.
The function hpx::resume is used to resume the HPX runtime system. It can only be used when running HPX on a single locality. It will block waiting for all thread pools to be resumed. This function only be called when the runtime suspended, or already running in which case this function will do nothing.
- Return
- This function will always return zero.
- Note
- As long as ec is not pre-initialized to hpx::throws this function doesn’t throw but returns the result code using the parameter ec. Otherwise it throws an instance of hpx::exception.
- Parameters
ec
: [in,out] this represents the error status on exit, if this is pre-initialized to hpx::throws the function will throw on error instead.
-
boost::system::error_category const &
get_hpx_category
()¶ Returns generic HPX error category used for new errors.
-
boost::system::error_category const &
get_hpx_rethrow_category
()¶ Returns generic HPX error category used for errors re-thrown after the exception has been de-serialized.
-
error_code
make_success_code
(throwmode mode = plain)¶ Returns error_code(hpx::success, “success”, mode).
-
std::string
diagnostic_information
(exception_info const &xi)¶ Extract the diagnostic information embedded in the given exception and return a string holding a formatted message.
The function hpx::diagnostic_information can be used to extract all diagnostic information stored in the given exception instance as a formatted string. This simplifies debug output as it composes the diagnostics into one, easy to use function call. This includes the name of the source file and line number, the sequence number of the OS-thread and the HPX-thread id, the locality id and the stack backtrace of the point where the original exception was thrown.
- Return
- The formatted string holding all of the available diagnostic information stored in the given exception instance.
- See
- hpx::get_error_locality_id(), hpx::get_error_host_name(), hpx::get_error_process_id(), hpx::get_error_function_name(), hpx::get_error_file_name(), hpx::get_error_line_number(), hpx::get_error_os_thread(), hpx::get_error_thread_id(), hpx::get_error_thread_description(), hpx::get_error(), hpx::get_error_backtrace(), hpx::get_error_env(), hpx::get_error_what(), hpx::get_error_config(), hpx::get_error_state()
- Parameters
xi
: The parametere
will be inspected for all diagnostic information elements which have been stored at the point where the exception was thrown. This parameter can be one of the following types: hpx::exception_info, hpx::error_code, std::exception, or std::exception_ptr.
- Exceptions
std::bad_alloc
: (if any of the required allocation operations fail)
-
std::string
get_error_what
(exception_info const &xi)¶ Return the error message of the thrown exception.
The function hpx::get_error_what can be used to extract the diagnostic information element representing the error message as stored in the given exception instance.
- Return
- The error message stored in the exception If the exception instance does not hold this information, the function will return an empty string.
- See
- hpx::diagnostic_information(), hpx::get_error_host_name(), hpx::get_error_process_id(), hpx::get_error_function_name(), hpx::get_error_file_name(), hpx::get_error_line_number(), hpx::get_error_os_thread(), hpx::get_error_thread_id(), hpx::get_error_thread_description(), hpx::get_error() hpx::get_error_backtrace(), hpx::get_error_env(), hpx::get_error_config(), hpx::get_error_state()
- Parameters
xi
: The parametere
will be inspected for the requested diagnostic information elements which have been stored at the point where the exception was thrown. This parameter can be one of the following types: hpx::exception_info, hpx::error_code, std::exception, or std::exception_ptr.
- Exceptions
std::bad_alloc
: (if one of the required allocations fails)
-
std::uint32_t
get_error_locality_id
(hpx::exception_info const &xi)¶ Return the locality id where the exception was thrown.
The function hpx::get_error_locality_id can be used to extract the diagnostic information element representing the locality id as stored in the given exception instance.
- Return
- The locality id of the locality where the exception was thrown. If the exception instance does not hold this information, the function will return hpx::naming::invalid_locality_id.
- See
- hpx::diagnostic_information(), hpx::get_error_host_name(), hpx::get_error_process_id(), hpx::get_error_function_name(), hpx::get_error_file_name(), hpx::get_error_line_number(), hpx::get_error_os_thread(), hpx::get_error_thread_id(), hpx::get_error_thread_description(), hpx::get_error(), hpx::get_error_backtrace(), hpx::get_error_env(), hpx::get_error_what(), hpx::get_error_config(), hpx::get_error_state()
- Parameters
xi
: The parametere
will be inspected for the requested diagnostic information elements which have been stored at the point where the exception was thrown. This parameter can be one of the following types: hpx::exception_info, hpx::error_code, std::exception, or std::exception_ptr.
- Exceptions
nothing
:
-
error
get_error
(hpx::exception const &e)¶ Return the locality id where the exception was thrown.
The function hpx::get_error can be used to extract the diagnostic information element representing the error value code as stored in the given exception instance.
- Return
- The error value code of the locality where the exception was thrown. If the exception instance does not hold this information, the function will return hpx::naming::invalid_locality_id.
- See
- hpx::diagnostic_information(), hpx::get_error_host_name(), hpx::get_error_process_id(), hpx::get_error_function_name(), hpx::get_error_file_name(), hpx::get_error_line_number(), hpx::get_error_os_thread(), hpx::get_error_thread_id(), hpx::get_error_thread_description(), hpx::get_error_backtrace(), hpx::get_error_env(), hpx::get_error_what(), hpx::get_error_config(), hpx::get_error_state()
- Parameters
e
: The parametere
will be inspected for the requested diagnostic information elements which have been stored at the point where the exception was thrown. This parameter can be one of the following types: hpx::exception, hpx::error_code, or std::exception_ptr.
- Exceptions
nothing
:
-
error
get_error
(hpx::error_code const &e)¶ Return the locality id where the exception was thrown.
The function hpx::get_error can be used to extract the diagnostic information element representing the error value code as stored in the given exception instance.
- Return
- The error value code of the locality where the exception was thrown. If the exception instance does not hold this information, the function will return hpx::naming::invalid_locality_id.
- See
- hpx::diagnostic_information(), hpx::get_error_host_name(), hpx::get_error_process_id(), hpx::get_error_function_name(), hpx::get_error_file_name(), hpx::get_error_line_number(), hpx::get_error_os_thread(), hpx::get_error_thread_id(), hpx::get_error_thread_description(), hpx::get_error_backtrace(), hpx::get_error_env(), hpx::get_error_what(), hpx::get_error_config(), hpx::get_error_state()
- Parameters
e
: The parametere
will be inspected for the requested diagnostic information elements which have been stored at the point where the exception was thrown. This parameter can be one of the following types: hpx::exception, hpx::error_code, or std::exception_ptr.
- Exceptions
nothing
:
-
std::string
get_error_host_name
(hpx::exception_info const &xi)¶ Return the hostname of the locality where the exception was thrown.
The function hpx::get_error_host_name can be used to extract the diagnostic information element representing the host name as stored in the given exception instance.
- Return
- The hostname of the locality where the exception was thrown. If the exception instance does not hold this information, the function will return and empty string.
- See
- hpx::diagnostic_information() hpx::get_error_process_id(), hpx::get_error_function_name(), hpx::get_error_file_name(), hpx::get_error_line_number(), hpx::get_error_os_thread(), hpx::get_error_thread_id(), hpx::get_error_thread_description(), hpx::get_error() hpx::get_error_backtrace(), hpx::get_error_env(), hpx::get_error_what(), hpx::get_error_config(), hpx::get_error_state()
- Parameters
xi
: The parametere
will be inspected for the requested diagnostic information elements which have been stored at the point where the exception was thrown. This parameter can be one of the following types: hpx::exception_info, hpx::error_code, std::exception, or std::exception_ptr.
- Exceptions
std::bad_alloc
: (if one of the required allocations fails)
-
std::int64_t
get_error_process_id
(hpx::exception_info const &xi)¶ Return the (operating system) process id of the locality where the exception was thrown.
The function hpx::get_error_process_id can be used to extract the diagnostic information element representing the process id as stored in the given exception instance.
- Return
- The process id of the OS-process which threw the exception If the exception instance does not hold this information, the function will return 0.
- See
- hpx::diagnostic_information(), hpx::get_error_host_name(), hpx::get_error_function_name(), hpx::get_error_file_name(), hpx::get_error_line_number(), hpx::get_error_os_thread(), hpx::get_error_thread_id(), hpx::get_error_thread_description(), hpx::get_error(), hpx::get_error_backtrace(), hpx::get_error_env(), hpx::get_error_what(), hpx::get_error_config(), hpx::get_error_state()
- Parameters
xi
: The parametere
will be inspected for the requested diagnostic information elements which have been stored at the point where the exception was thrown. This parameter can be one of the following types: hpx::exception_info, hpx::error_code, std::exception, or std::exception_ptr.
- Exceptions
nothing
:
-
std::string
get_error_env
(hpx::exception_info const &xi)¶ Return the environment of the OS-process at the point the exception was thrown.
The function hpx::get_error_env can be used to extract the diagnostic information element representing the environment of the OS-process collected at the point the exception was thrown.
- Return
- The environment from the point the exception was thrown. If the exception instance does not hold this information, the function will return an empty string.
- See
- hpx::diagnostic_information(), hpx::get_error_host_name(), hpx::get_error_process_id(), hpx::get_error_function_name(), hpx::get_error_file_name(), hpx::get_error_line_number(), hpx::get_error_os_thread(), hpx::get_error_thread_id(), hpx::get_error_thread_description(), hpx::get_error(), hpx::get_error_backtrace(), hpx::get_error_what(), hpx::get_error_config(), hpx::get_error_state()
- Parameters
xi
: The parametere
will be inspected for the requested diagnostic information elements which have been stored at the point where the exception was thrown. This parameter can be one of the following types: hpx::exception_info, hpx::error_code, std::exception, or std::exception_ptr.
- Exceptions
std::bad_alloc
: (if one of the required allocations fails)
-
std::string
get_error_function_name
(hpx::exception_info const &xi)¶ Return the function name from which the exception was thrown.
The function hpx::get_error_function_name can be used to extract the diagnostic information element representing the name of the function as stored in the given exception instance.
- Return
- The name of the function from which the exception was thrown. If the exception instance does not hold this information, the function will return an empty string.
- See
- hpx::diagnostic_information(), hpx::get_error_host_name(), hpx::get_error_process_id() hpx::get_error_file_name(), hpx::get_error_line_number(), hpx::get_error_os_thread(), hpx::get_error_thread_id(), hpx::get_error_thread_description(), hpx::get_error(), hpx::get_error_backtrace(), hpx::get_error_env(), hpx::get_error_what(), hpx::get_error_config(), hpx::get_error_state()
- Parameters
xi
: The parametere
will be inspected for the requested diagnostic information elements which have been stored at the point where the exception was thrown. This parameter can be one of the following types: hpx::exception_info, hpx::error_code, std::exception, or std::exception_ptr.
- Exceptions
std::bad_alloc
: (if one of the required allocations fails)
-
std::string
get_error_backtrace
(hpx::exception_info const &xi)¶ Return the stack backtrace from the point the exception was thrown.
The function hpx::get_error_backtrace can be used to extract the diagnostic information element representing the stack backtrace collected at the point the exception was thrown.
- Return
- The stack back trace from the point the exception was thrown. If the exception instance does not hold this information, the function will return an empty string.
- See
- hpx::diagnostic_information(), hpx::get_error_host_name(), hpx::get_error_process_id(), hpx::get_error_function_name(), hpx::get_error_file_name(), hpx::get_error_line_number(), hpx::get_error_os_thread(), hpx::get_error_thread_id(), hpx::get_error_thread_description(), hpx::get_error(), hpx::get_error_env(), hpx::get_error_what(), hpx::get_error_config(), hpx::get_error_state()
- Parameters
xi
: The parametere
will be inspected for the requested diagnostic information elements which have been stored at the point where the exception was thrown. This parameter can be one of the following types: hpx::exception_info, hpx::error_code, std::exception, or std::exception_ptr.
- Exceptions
std::bad_alloc
: (if one of the required allocations fails)
-
std::string
get_error_file_name
(hpx::exception_info const &xi)¶ Return the (source code) file name of the function from which the exception was thrown.
The function hpx::get_error_file_name can be used to extract the diagnostic information element representing the name of the source file as stored in the given exception instance.
- Return
- The name of the source file of the function from which the exception was thrown. If the exception instance does not hold this information, the function will return an empty string.
- See
- hpx::diagnostic_information(), hpx::get_error_host_name(), hpx::get_error_process_id(), hpx::get_error_function_name(), hpx::get_error_line_number(), hpx::get_error_os_thread(), hpx::get_error_thread_id(), hpx::get_error_thread_description(), hpx::get_error(), hpx::get_error_backtrace(), hpx::get_error_env(), hpx::get_error_what(), hpx::get_error_config(), hpx::get_error_state()
- Parameters
xi
: The parametere
will be inspected for the requested diagnostic information elements which have been stored at the point where the exception was thrown. This parameter can be one of the following types: hpx::exception_info, hpx::error_code, std::exception, or std::exception_ptr.
- Exceptions
std::bad_alloc
: (if one of the required allocations fails)
-
long
get_error_line_number
(hpx::exception_info const &xi)¶ Return the line number in the (source code) file of the function from which the exception was thrown.
The function hpx::get_error_line_number can be used to extract the diagnostic information element representing the line number as stored in the given exception instance.
- Return
- The line number of the place where the exception was thrown. If the exception instance does not hold this information, the function will return -1.
- See
- hpx::diagnostic_information(), hpx::get_error_host_name(), hpx::get_error_process_id(), hpx::get_error_function_name(), hpx::get_error_file_name() hpx::get_error_os_thread(), hpx::get_error_thread_id(), hpx::get_error_thread_description(), hpx::get_error(), hpx::get_error_backtrace(), hpx::get_error_env(), hpx::get_error_what(), hpx::get_error_config(), hpx::get_error_state()
- Parameters
xi
: The parametere
will be inspected for the requested diagnostic information elements which have been stored at the point where the exception was thrown. This parameter can be one of the following types: hpx::exception_info, hpx::error_code, std::exception, or std::exception_ptr.
- Exceptions
nothing
:
-
std::size_t
get_error_os_thread
(hpx::exception_info const &xi)¶ Return the sequence number of the OS-thread used to execute HPX-threads from which the exception was thrown.
The function hpx::get_error_os_thread can be used to extract the diagnostic information element representing the sequence number of the OS-thread as stored in the given exception instance.
- Return
- The sequence number of the OS-thread used to execute the HPX-thread from which the exception was thrown. If the exception instance does not hold this information, the function will return std::size(-1).
- See
- hpx::diagnostic_information(), hpx::get_error_host_name(), hpx::get_error_process_id(), hpx::get_error_function_name(), hpx::get_error_file_name(), hpx::get_error_line_number(), hpx::get_error_thread_id(), hpx::get_error_thread_description(), hpx::get_error(), hpx::get_error_backtrace(), hpx::get_error_env(), hpx::get_error_what(), hpx::get_error_config(), hpx::get_error_state()
- Parameters
xi
: The parametere
will be inspected for the requested diagnostic information elements which have been stored at the point where the exception was thrown. This parameter can be one of the following types: hpx::exception_info, hpx::error_code, std::exception, or std::exception_ptr.
- Exceptions
nothing
:
-
std::size_t
get_error_thread_id
(hpx::exception_info const &xi)¶ Return the unique thread id of the HPX-thread from which the exception was thrown.
The function hpx::get_error_thread_id can be used to extract the diagnostic information element representing the HPX-thread id as stored in the given exception instance.
- Return
- The unique thread id of the HPX-thread from which the exception was thrown. If the exception instance does not hold this information, the function will return std::size_t(0).
- See
- hpx::diagnostic_information(), hpx::get_error_host_name(), hpx::get_error_process_id(), hpx::get_error_function_name(), hpx::get_error_file_name(), hpx::get_error_line_number(), hpx::get_error_os_thread() hpx::get_error_thread_description(), hpx::get_error(), hpx::get_error_backtrace(), hpx::get_error_env(), hpx::get_error_what(), hpx::get_error_config(), hpx::get_error_state()
- Parameters
xi
: The parametere
will be inspected for the requested diagnostic information elements which have been stored at the point where the exception was thrown. This parameter can be one of the following types: hpx::exception_info, hpx::error_code, std::exception, or std::exception_ptr.
- Exceptions
nothing
:
-
std::string
get_error_thread_description
(hpx::exception_info const &xi)¶ Return any additionally available thread description of the HPX-thread from which the exception was thrown.
The function hpx::get_error_thread_description can be used to extract the diagnostic information element representing the additional thread description as stored in the given exception instance.
- Return
- Any additionally available thread description of the HPX-thread from which the exception was thrown. If the exception instance does not hold this information, the function will return an empty string.
- See
- hpx::diagnostic_information(), hpx::get_error_host_name(), hpx::get_error_process_id(), hpx::get_error_function_name(), hpx::get_error_file_name(), hpx::get_error_line_number(), hpx::get_error_os_thread(), hpx::get_error_thread_id(), hpx::get_error_backtrace(), hpx::get_error_env(), hpx::get_error(), hpx::get_error_state(), hpx::get_error_what(), hpx::get_error_config()
- Parameters
xi
: The parametere
will be inspected for the requested diagnostic information elements which have been stored at the point where the exception was thrown. This parameter can be one of the following types: hpx::exception_info, hpx::error_code, std::exception, or std::exception_ptr.
- Exceptions
std::bad_alloc
: (if one of the required allocations fails)
-
std::string
get_error_config
(hpx::exception_info const &xi)¶ Return the HPX configuration information point from which the exception was thrown.
The function hpx::get_error_config can be used to extract the HPX configuration information element representing the full HPX configuration information as stored in the given exception instance.
- Return
- Any additionally available HPX configuration information the point from which the exception was thrown. If the exception instance does not hold this information, the function will return an empty string.
- See
- hpx::diagnostic_information(), hpx::get_error_host_name(), hpx::get_error_process_id(), hpx::get_error_function_name(), hpx::get_error_file_name(), hpx::get_error_line_number(), hpx::get_error_os_thread(), hpx::get_error_thread_id(), hpx::get_error_backtrace(), hpx::get_error_env(), hpx::get_error(), hpx::get_error_state() hpx::get_error_what(), hpx::get_error_thread_description()
- Parameters
xi
: The parametere
will be inspected for the requested diagnostic information elements which have been stored at the point where the exception was thrown. This parameter can be one of the following types: hpx::exception_info, hpx::error_code, std::exception, or std::exception_ptr.
- Exceptions
std::bad_alloc
: (if one of the required allocations fails)
-
std::string
get_error_state
(hpx::exception_info const &xi)¶ Return the HPX runtime state information at which the exception was thrown.
The function hpx::get_error_state can be used to extract the HPX runtime state information element representing the state the runtime system is currently in as stored in the given exception instance.
- Return
- The point runtime state at the point at which the exception was thrown. If the exception instance does not hold this information, the function will return an empty string.
- See
- hpx::diagnostic_information(), hpx::get_error_host_name(), hpx::get_error_process_id(), hpx::get_error_function_name(), hpx::get_error_file_name(), hpx::get_error_line_number(), hpx::get_error_os_thread(), hpx::get_error_thread_id(), hpx::get_error_backtrace(), hpx::get_error_env(), hpx::get_error(), hpx::get_error_what(), hpx::get_error_thread_description()
- Parameters
xi
: The parametere
will be inspected for the requested diagnostic information elements which have been stored at the point where the exception was thrown. This parameter can be one of the following types: hpx::exception_info, hpx::error_code, std::exception, or std::exception_ptr.
- Exceptions
std::bad_alloc
: (if one of the required allocations fails)
-
bool
register_thread
(runtime *rt, char const *name, error_code &ec = throws)¶ Register the current kernel thread with HPX, this should be done once for each external OS-thread intended to invoke HPX functionality. Calling this function more than once will silently fail.
-
void
unregister_thread
(runtime *rt)¶ Unregister the thread from HPX, this should be done once in the end before the external thread exists.
-
naming::gid_type const &
get_locality
()¶ The function get_locality returns a reference to the locality prefix.
-
std::size_t
get_runtime_instance_number
()¶ The function get_runtime_instance_number returns a unique number associated with the runtime instance the current thread is running in.
-
bool
register_on_exit
(util::function_nonser<void()> const&)¶ Register a function to be called during system shutdown.
-
bool
is_starting
()¶ Test whether the runtime system is currently being started.
This function returns whether the runtime system is currently being started or not, e.g. whether the current state of the runtime system is hpx::state_startup
- Note
- This function needs to be executed on a HPX-thread. It will return false otherwise.
-
bool
tolerate_node_faults
()¶ Test if HPX runs in fault-tolerant mode.
This function returns whether the runtime system is running in fault-tolerant mode
-
bool
is_running
()¶ Test whether the runtime system is currently running.
This function returns whether the runtime system is currently running or not, e.g. whether the current state of the runtime system is hpx::state_running
- Note
- This function needs to be executed on a HPX-thread. It will return false otherwise.
-
bool
is_stopped
()¶ Test whether the runtime system is currently stopped.
This function returns whether the runtime system is currently stopped or not, e.g. whether the current state of the runtime system is hpx::state_stopped
- Note
- This function needs to be executed on a HPX-thread. It will return false otherwise.
-
bool
is_stopped_or_shutting_down
()¶ Test whether the runtime system is currently being shut down.
This function returns whether the runtime system is currently being shut down or not, e.g. whether the current state of the runtime system is hpx::state_stopped or hpx::state_shutdown
- Note
- This function needs to be executed on a HPX-thread. It will return false otherwise.
-
std::size_t
get_num_worker_threads
()¶ Return the number of worker OS- threads used to execute HPX threads.
This function returns the number of OS-threads used to execute HPX threads. If the function is called while no HPX runtime system is active, it will return zero.
-
std::uint64_t
get_system_uptime
()¶ Return the system uptime measure on the thread executing this call.
This function returns the system uptime measured in nanoseconds for the thread executing this call. If the function is called while no HPX runtime system is active, it will return zero.
-
void
start_active_counters
(error_code &ec = throws)¶ Start all active performance counters, optionally naming the section of code.
- Note
- As long as ec is not pre-initialized to hpx::throws this function doesn’t throw but returns the result code using the parameter ec. Otherwise it throws an instance of hpx::exception.
- Note
- The active counters are those which have been specified on the command line while executing the application (see command line option –hpx:print-counter)
- Parameters
ec
: [in,out] this represents the error status on exit, if this is pre-initialized to hpx::throws the function will throw on error instead.
-
void
reset_active_counters
(error_code &ec = throws)¶ Resets all active performance counters.
- Note
- As long as ec is not pre-initialized to hpx::throws this function doesn’t throw but returns the result code using the parameter ec. Otherwise it throws an instance of hpx::exception.
- Note
- The active counters are those which have been specified on the command line while executing the application (see command line option –hpx:print-counter)
- Parameters
ec
: [in,out] this represents the error status on exit, if this is pre-initialized to hpx::throws the function will throw on error instead.
-
void
reinit_active_counters
(bool reset = true, error_code &ec = throws)¶ Re-initialize all active performance counters.
- Note
- As long as ec is not pre-initialized to hpx::throws this function doesn’t throw but returns the result code using the parameter ec. Otherwise it throws an instance of hpx::exception.
- Note
- The active counters are those which have been specified on the command line while executing the application (see command line option –hpx:print-counter)
- Parameters
reset
: [in] Reset the current values before re-initializing counters (default: true)ec
: [in,out] this represents the error status on exit, if this is pre-initialized to hpx::throws the function will throw on error instead.
-
void
stop_active_counters
(error_code &ec = throws)¶ Stop all active performance counters.
- Note
- As long as ec is not pre-initialized to hpx::throws this function doesn’t throw but returns the result code using the parameter ec. Otherwise it throws an instance of hpx::exception.
- Note
- The active counters are those which have been specified on the command line while executing the application (see command line option –hpx:print-counter)
- Parameters
ec
: [in,out] this represents the error status on exit, if this is pre-initialized to hpx::throws the function will throw on error instead.
-
void
evaluate_active_counters
(bool reset = false, char const *description = nullptr, error_code &ec = throws)¶ Evaluate and output all active performance counters, optionally naming the point in code marked by this function.
- Note
- As long as ec is not pre-initialized to hpx::throws this function doesn’t throw but returns the result code using the parameter ec. Otherwise it throws an instance of hpx::exception.
- Note
- The output generated by this function is redirected to the destination specified by the corresponding command line options (see –hpx:print-counter-destination).
- Note
- The active counters are those which have been specified on the command line while executing the application (see command line option –hpx:print-counter)
- Parameters
reset
: [in] this is an optional flag allowing to reset the counter value after it has been evaluated.description
: [in] this is an optional value naming the point in the code marked by the call to this function.ec
: [in,out] this represents the error status on exit, if this is pre-initialized to hpx::throws the function will throw on error instead.
-
serialization::binary_filter *
create_binary_filter
(char const *binary_filter_type, bool compress, serialization::binary_filter *next_filter = nullptr, error_code &ec = throws)¶ Create an instance of a binary filter plugin.
- Note
- As long as ec is not pre-initialized to hpx::throws this function doesn’t throw but returns the result code using the parameter ec. Otherwise it throws an instance of hpx::exception.
- Parameters
binary_filter_type
: [in] The type of the binary filter to createcompress
: [in] The created filter should support compressionnext_filter
: [in] Use this as the filter to dispatch the invocation into.ec
: [in,out] this represents the error status on exit, if this is pre-initialized to hpx::throws the function will throw on error instead.
-
std::vector<Client>
find_all_from_basename
(std::string base_name, std::size_t num_ids)¶ Return all registered ids from all localities from the given base name.
This function locates all ids which were registered with the given base name. It returns a list of futures representing those ids.
Return all registered clients from all localities from the given base name.
- Return
- A list of futures representing the ids which were registered using the given base name.
- Note
- The futures will become ready even if the event (for instance, binding the name to an id) has already happened in the past. This is important in order to reliably retrieve ids from a name, even if the name was already registered.
- Parameters
base_name
: [in] The base name for which to retrieve the registered ids.num_ids
: [in] The number of registered ids to expect.
This function locates all ids which were registered with the given base name. It returns a list of futures representing those ids.
- Return
- A list of futures representing the ids which were registered using the given base name.
- Note
- The futures embedded in the returned client objects will become ready even if the event (for instance, binding the name to an id) has already happened in the past. This is important in order to reliably retrieve ids from a name, even if the name was already registered.
- Template Parameters
Client
: The client type to return
- Parameters
base_name
: [in] The base name for which to retrieve the registered ids.num_ids
: [in] The number of registered ids to expect.
-
std::vector<Client>
find_from_basename
(std::string base_name, std::vector<std::size_t> const &ids)¶ Return registered ids from the given base name and sequence numbers.
This function locates the ids which were registered with the given base name and the given sequence numbers. It returns a list of futures representing those ids.
Return registered clients from the given base name and sequence numbers.
- Return
- A list of futures representing the ids which were registered using the given base name and sequence numbers.
- Note
- The futures will become ready even if the event (for instance, binding the name to an id) has already happened in the past. This is important in order to reliably retrieve ids from a name, even if the name was already registered.
- Parameters
base_name
: [in] The base name for which to retrieve the registered ids.ids
: [in] The sequence numbers of the registered ids.
This function locates the ids which were registered with the given base name and the given sequence numbers. It returns a list of futures representing those ids.
- Return
- A list of futures representing the ids which were registered using the given base name and sequence numbers.
- Note
- The futures embedded in the returned client objects will become ready even if the event (for instance, binding the name to an id) has already happened in the past. This is important in order to reliably retrieve ids from a name, even if the name was already registered.
- Template Parameters
Client
: The client type to return
- Parameters
base_name
: [in] The base name for which to retrieve the registered ids.ids
: [in] The sequence numbers of the registered ids.
-
Client
find_from_basename
(std::string base_name, std::size_t sequence_nr = ~0U)¶ Return registered id from the given base name and sequence number.
This function locates the id which was registered with the given base name and the given sequence number. It returns a future representing those id.
This function locates the id which was registered with the given base name and the given sequence number. It returns a future representing those id.
- Return
- A representing the id which was registered using the given base name and sequence numbers.
- Note
- The future will become ready even if the event (for instance, binding the name to an id) has already happened in the past. This is important in order to reliably retrieve ids from a name, even if the name was already registered.
- Parameters
base_name
: [in] The base name for which to retrieve the registered ids.sequence_nr
: [in] The sequence number of the registered id.
- Return
- A representing the id which was registered using the given base name and sequence numbers.
- Note
- The future embedded in the returned client object will become ready even if the event (for instance, binding the name to an id) has already happened in the past. This is important in order to reliably retrieve ids from a name, even if the name was already registered.
- Template Parameters
Client
: The client type to return
- Parameters
base_name
: [in] The base name for which to retrieve the registered ids.sequence_nr
: [in] The sequence number of the registered id.
-
hpx::future<bool>
register_with_basename
(std::string base_name, hpx::id_type id, std::size_t sequence_nr = ~0U)¶ Register the given id using the given base name.
The function registers the given ids using the provided base name.
- Return
- A future representing the result of the registration operation itself.
- Note
- The operation will fail if the given sequence number is not unique.
- Parameters
base_name
: [in] The base name for which to retrieve the registered ids.id
: [in] The id to register using the given base name.sequence_nr
: [in, optional] The sequential number to use for the registration of the id. This number has to be unique system wide for each registration using the same base name. The default is the current locality identifier. Also, the sequence numbers have to be consecutive starting from zero.
-
hpx::future<bool>
register_with_basename
(std::string base_name, hpx::future<hpx::id_type> f, std::size_t sequence_nr = ~0U)¶ Register the id wrapped in the given future using the given base name.
The function registers the object the given future refers to using the provided base name.
- Return
- A future representing the result of the registration operation itself.
- Note
- The operation will fail if the given sequence number is not unique.
- Parameters
base_name
: [in] The base name for which to retrieve the registered ids.f
: [in] The future which should be registered using the given base name.sequence_nr
: [in, optional] The sequential number to use for the registration of the id. This number has to be unique system wide for each registration using the same base name. The default is the current locality identifier. Also, the sequence numbers have to be consecutive starting from zero.
-
template<typename
Client
, typenameStub
>
hpx::future<bool>register_with_basename
(std::string base_name, components::client_base<Client, Stub> &client, std::size_t sequence_nr = ~0U)¶ Register the id wrapped in the given client using the given base name.
The function registers the object the given client refers to using the provided base name.
- Return
- A future representing the result of the registration operation itself.
- Note
- The operation will fail if the given sequence number is not unique.
- Template Parameters
Client
: The client type to register
- Parameters
base_name
: [in] The base name for which to retrieve the registered ids.client
: [in] The client which should be registered using the given base name.sequence_nr
: [in, optional] The sequential number to use for the registration of the id. This number has to be unique system wide for each registration using the same base name. The default is the current locality identifier. Also, the sequence numbers have to be consecutive starting from zero.
-
Client
unregister_with_basename
(std::string base_name, std::size_t sequence_nr = ~0U)¶ Unregister the given id using the given base name.
The function unregisters the given ids using the provided base name.
Unregister the given base name.
- Return
- A future representing the result of the un-registration operation itself.
- Parameters
base_name
: [in] The base name for which to retrieve the registered ids.sequence_nr
: [in, optional] The sequential number to use for the un-registration. This number has to be the same as has been used with register_with_basename before.
The function unregisters the given ids using the provided base name.
- Return
- A future representing the result of the un-registration operation itself.
- Template Parameters
Client
: The client type to return
- Parameters
base_name
: [in] The base name for which to retrieve the registered ids.sequence_nr
: [in, optional] The sequential number to use for the un-registration. This number has to be the same as has been used with register_with_basename before.
-
naming::id_type
find_here
(error_code &ec = throws)¶ Return the global id representing this locality.
The function find_here() can be used to retrieve the global id usable to refer to the current locality.
- Note
- Generally, the id of a locality can be used for instance to create new instances of components and to invoke plain actions (global functions).
- Return
- The global id representing the locality this function has been called on.
- Note
- As long as ec is not pre-initialized to hpx::throws this function doesn’t throw but returns the result code using the parameter ec. Otherwise it throws an instance of hpx::exception.
- Note
- This function will return meaningful results only if called from an HPX-thread. It will return hpx::naming::invalid_id otherwise.
- See
- hpx::find_all_localities(), hpx::find_locality()
- Parameters
ec
: [in,out] this represents the error status on exit, if this is pre-initialized to hpx::throws the function will throw on error instead.
-
naming::id_type
find_root_locality
(error_code &ec = throws)¶ Return the global id representing the root locality.
The function find_root_locality() can be used to retrieve the global id usable to refer to the root locality. The root locality is the locality where the main AGAS service is hosted.
- Note
- Generally, the id of a locality can be used for instance to create new instances of components and to invoke plain actions (global functions).
- Return
- The global id representing the root locality for this application.
- Note
- As long as ec is not pre-initialized to hpx::throws this function doesn’t throw but returns the result code using the parameter ec. Otherwise it throws an instance of hpx::exception.
- Note
- This function will return meaningful results only if called from an HPX-thread. It will return hpx::naming::invalid_id otherwise.
- See
- hpx::find_all_localities(), hpx::find_locality()
- Parameters
ec
: [in,out] this represents the error status on exit, if this is pre-initialized to hpx::throws the function will throw on error instead.
-
std::vector<naming::id_type>
find_all_localities
(error_code &ec = throws)¶ Return the list of global ids representing all localities available to this application.
The function find_all_localities() can be used to retrieve the global ids of all localities currently available to this application.
- Note
- Generally, the id of a locality can be used for instance to create new instances of components and to invoke plain actions (global functions).
- Return
- The global ids representing the localities currently available to this application.
- Note
- As long as ec is not pre-initialized to hpx::throws this function doesn’t throw but returns the result code using the parameter ec. Otherwise it throws an instance of hpx::exception.
- Note
- This function will return meaningful results only if called from an HPX-thread. It will return an empty vector otherwise.
- See
- hpx::find_here(), hpx::find_locality()
- Parameters
ec
: [in,out] this represents the error status on exit, if this is pre-initialized to hpx::throws the function will throw on error instead.
-
std::vector<naming::id_type>
find_all_localities
(components::component_type type, error_code &ec = throws)¶ Return the list of global ids representing all localities available to this application which support the given component type.
The function find_all_localities() can be used to retrieve the global ids of all localities currently available to this application which support the creation of instances of the given component type.
- Note
- Generally, the id of a locality can be used for instance to create new instances of components and to invoke plain actions (global functions).
- Return
- The global ids representing the localities currently available to this application which support the creation of instances of the given component type. If no localities supporting the given component type are currently available, this function will return an empty vector.
- Note
- As long as ec is not pre-initialized to hpx::throws this function doesn’t throw but returns the result code using the parameter ec. Otherwise it throws an instance of hpx::exception.
- Note
- This function will return meaningful results only if called from an HPX-thread. It will return an empty vector otherwise.
- See
- hpx::find_here(), hpx::find_locality()
- Parameters
type
: [in] The type of the components for which the function should return the available localities.ec
: [in,out] this represents the error status on exit, if this is pre-initialized to hpx::throws the function will throw on error instead.
-
std::vector<naming::id_type>
find_remote_localities
(error_code &ec = throws)¶ Return the list of locality ids of remote localities supporting the given component type. By default this function will return the list of all remote localities (all but the current locality).
The function find_remote_localities() can be used to retrieve the global ids of all remote localities currently available to this application (i.e. all localities except the current one).
- Note
- Generally, the id of a locality can be used for instance to create new instances of components and to invoke plain actions (global functions).
- Return
- The global ids representing the remote localities currently available to this application.
- Note
- As long as ec is not pre-initialized to hpx::throws this function doesn’t throw but returns the result code using the parameter ec. Otherwise it throws an instance of hpx::exception.
- Note
- This function will return meaningful results only if called from an HPX-thread. It will return an empty vector otherwise.
- See
- hpx::find_here(), hpx::find_locality()
- Parameters
ec
: [in,out] this represents the error status on exit, if this is pre-initialized to hpx::throws the function will throw on error instead.
-
std::vector<naming::id_type>
find_remote_localities
(components::component_type type, error_code &ec = throws)¶ Return the list of locality ids of remote localities supporting the given component type. By default this function will return the list of all remote localities (all but the current locality).
The function find_remote_localities() can be used to retrieve the global ids of all remote localities currently available to this application (i.e. all localities except the current one) which support the creation of instances of the given component type.
- Note
- Generally, the id of a locality can be used for instance to create new instances of components and to invoke plain actions (global functions).
- Return
- The global ids representing the remote localities currently available to this application.
- Note
- As long as ec is not pre-initialized to hpx::throws this function doesn’t throw but returns the result code using the parameter ec. Otherwise it throws an instance of hpx::exception.
- Note
- This function will return meaningful results only if called from an HPX-thread. It will return an empty vector otherwise.
- See
- hpx::find_here(), hpx::find_locality()
- Parameters
type
: [in] The type of the components for which the function should return the available remote localities.ec
: [in,out] this represents the error status on exit, if this is pre-initialized to hpx::throws the function will throw on error instead.
-
naming::id_type
find_locality
(components::component_type type, error_code &ec = throws)¶ Return the global id representing an arbitrary locality which supports the given component type.
The function find_locality() can be used to retrieve the global id of an arbitrary locality currently available to this application which supports the creation of instances of the given component type.
- Note
- Generally, the id of a locality can be used for instance to create new instances of components and to invoke plain actions (global functions).
- Return
- The global id representing an arbitrary locality currently available to this application which supports the creation of instances of the given component type. If no locality supporting the given component type is currently available, this function will return hpx::naming::invalid_id.
- Note
- As long as ec is not pre-initialized to hpx::throws this function doesn’t throw but returns the result code using the parameter ec. Otherwise it throws an instance of hpx::exception.
- Note
- This function will return meaningful results only if called from an HPX-thread. It will return hpx::naming::invalid_id otherwise.
- See
- hpx::find_here(), hpx::find_all_localities()
- Parameters
type
: [in] The type of the components for which the function should return any available locality.ec
: [in,out] this represents the error status on exit, if this is pre-initialized to hpx::throws the function will throw on error instead.
-
naming::id_type
get_colocation_id
(launch::sync_policy, naming::id_type const &id, error_code &ec = throws)¶ Return the id of the locality where the object referenced by the given id is currently located on.
The function hpx::get_colocation_id() returns the id of the locality where the given object is currently located.
- Note
- As long as ec is not pre-initialized to hpx::throws this function doesn’t throw but returns the result code using the parameter ec. Otherwise it throws an instance of hpx::exception.
- See
- hpx::get_colocation_id()
- Parameters
id
: [in] The id of the object to locate.ec
: [in,out] this represents the error status on exit, if this is pre-initialized to hpx::throws the function will throw on error instead.
-
lcos::future<naming::id_type>
get_colocation_id
(naming::id_type const &id)¶ Asynchronously return the id of the locality where the object referenced by the given id is currently located on.
- See
- hpx::get_colocation_id(launch::sync_policy)
- Parameters
id
: [in] The id of the object to locate.
Returns a future referring to the pointer to the underlying memory of a component.
The function hpx::get_ptr can be used to extract a future referring to the pointer to the underlying memory of a given component.
- Return
- This function returns a future representing the pointer to the underlying memory for the component instance with the given id.
- Note
- This function will successfully return the requested result only if the given component is currently located on the calling locality. Otherwise the function will raise an error.
- Note
- The component instance the returned pointer refers to can not be migrated as long as there is at least one copy of the returned shared_ptr alive.
- Parameters
id
: [in] The global id of the component for which the pointer to the underlying memory should be retrieved.
- Template Parameters
The
: only template parameter has to be the type of the server side component.
Returns a future referring to the pointer to the underlying memory of a component.
The function hpx::get_ptr can be used to extract a future referring to the pointer to the underlying memory of a given component.
- Return
- This function returns a future representing the pointer to the underlying memory for the component instance with the given id.
- Note
- This function will successfully return the requested result only if the given component is currently located on the calling locality. Otherwise the function will raise an error.
- Note
- The component instance the returned pointer refers to can not be migrated as long as there is at least one copy of the returned shared_ptr alive.
- Parameters
c
: [in] A client side representation of the component for which the pointer to the underlying memory should be retrieved.
Returns the pointer to the underlying memory of a component.
The function hpx::get_ptr_sync can be used to extract the pointer to the underlying memory of a given component.
- Return
- This function returns the pointer to the underlying memory for the component instance with the given id.
- Note
- This function will successfully return the requested result only if the given component is currently located on the requesting locality. Otherwise the function will raise and error.
- Note
- The component instance the returned pointer refers to can not be migrated as long as there is at least one copy of the returned shared_ptr alive.
- Note
- As long as ec is not pre-initialized to hpx::throws this function doesn’t throw but returns the result code using the parameter ec. Otherwise it throws an instance of hpx::exception.
- Parameters
p
: [in] The parameter p represents a placeholder type to turn make the call synchronous.id
: [in] The global id of the component for which the pointer to the underlying memory should be retrieved.ec
: [in,out] this represents the error status on exit, if this is pre-initialized to hpx::throws the function will throw on error instead.
- Template Parameters
The
: only template parameter has to be the type of the server side component.
Returns the pointer to the underlying memory of a component.
The function hpx::get_ptr_sync can be used to extract the pointer to the underlying memory of a given component.
- Return
- This function returns the pointer to the underlying memory for the component instance with the given id.
- Note
- This function will successfully return the requested result only if the given component is currently located on the requesting locality. Otherwise the function will raise and error.
- Note
- The component instance the returned pointer refers to can not be migrated as long as there is at least one copy of the returned shared_ptr alive.
- Note
- As long as ec is not pre-initialized to hpx::throws this function doesn’t throw but returns the result code using the parameter ec. Otherwise it throws an instance of hpx::exception.
- Parameters
p
: [in] The parameter p represents a placeholder type to turn make the call synchronous.c
: [in] A client side representation of the component for which the pointer to the underlying memory should be retrieved.ec
: [in,out] this represents the error status on exit, if this is pre-initialized to hpx::throws the function will throw on error instead.
-
std::uint32_t
get_locality_id
(error_code &ec = throws)¶ Return the number of the locality this function is being called from.
This function returns the id of the current locality.
- Note
- The returned value is zero based and its maximum value is smaller than the overall number of localities the current application is running on (as returned by get_num_localities()).
- Note
- As long as ec is not pre-initialized to hpx::throws this function doesn’t throw but returns the result code using the parameter ec. Otherwise it throws an instance of hpx::exception.
- Note
- This function needs to be executed on a HPX-thread. It will fail otherwise (it will return -1).
- Parameters
ec
: [in,out] this represents the error status on exit, if this is pre-initialized to hpx::throws the function will throw on error instead.
-
std::string
get_locality_name
()¶ Return the name of the locality this function is called on.
This function returns the name for the locality on which this function is called.
- Return
- This function returns the name for the locality on which the function is called. The name is retrieved from the underlying networking layer and may be different for different parcelports.
- See
- future<std::string> get_locality_name(naming::id_type const& id)
-
future<std::string>
get_locality_name
(naming::id_type const &id)¶ Return the name of the referenced locality.
This function returns a future referring to the name for the locality of the given id.
- Return
- This function returns the name for the locality of the given id. The name is retrieved from the underlying networking layer and may be different for different parcel ports.
- See
- std::string get_locality_name()
- Parameters
id
: [in] The global id of the locality for which the name should be retrieved
-
std::uint32_t
get_initial_num_localities
()¶ Return the number of localities which were registered at startup for the running application.
The function get_initial_num_localities returns the number of localities which were connected to the console at application startup.
- Note
- As long as ec is not pre-initialized to hpx::throws this function doesn’t throw but returns the result code using the parameter ec. Otherwise it throws an instance of hpx::exception.
- See
- hpx::find_all_localities, hpx::get_num_localities
-
lcos::future<std::uint32_t>
get_num_localities
()¶ Asynchronously return the number of localities which are currently registered for the running application.
The function get_num_localities asynchronously returns the number of localities currently connected to the console. The returned future represents the actual result.
- Note
- This function will return meaningful results only if called from an HPX-thread. It will return 0 otherwise.
- See
- hpx::find_all_localities, hpx::get_num_localities
-
std::uint32_t
get_num_localities
(launch::sync_policy, error_code &ec = throws)¶ Return the number of localities which are currently registered for the running application.
The function get_num_localities returns the number of localities currently connected to the console.
- Note
- This function will return meaningful results only if called from an HPX-thread. It will return 0 otherwise.
- Note
- As long as ec is not pre-initialized to hpx::throws this function doesn’t throw but returns the result code using the parameter ec. Otherwise it throws an instance of hpx::exception.
- See
- hpx::find_all_localities, hpx::get_num_localities
- Parameters
ec
: [in,out] this represents the error status on exit, if this is pre-initialized to hpx::throws the function will throw on error instead.
-
lcos::future<std::uint32_t>
get_num_localities
(components::component_type t)¶ Asynchronously return the number of localities which are currently registered for the running application.
The function get_num_localities asynchronously returns the number of localities currently connected to the console which support the creation of the given component type. The returned future represents the actual result.
- Note
- This function will return meaningful results only if called from an HPX-thread. It will return 0 otherwise.
- See
- hpx::find_all_localities, hpx::get_num_localities
- Parameters
t
: The component type for which the number of connected localities should be retrieved.
-
std::uint32_t
get_num_localities
(launch::sync_policy, components::component_type t, error_code &ec = throws)¶ Synchronously return the number of localities which are currently registered for the running application.
The function get_num_localities returns the number of localities currently connected to the console which support the creation of the given component type. The returned future represents the actual result.
- Note
- This function will return meaningful results only if called from an HPX-thread. It will return 0 otherwise.
- See
- hpx::find_all_localities, hpx::get_num_localities
- Parameters
t
: The component type for which the number of connected localities should be retrieved.ec
: [in,out] this represents the error status on exit, if this is pre-initialized to hpx::throws the function will throw on error instead.
-
std::size_t
get_os_thread_count
()¶ Return the number of OS-threads running in the runtime instance the current HPX-thread is associated with.
-
std::size_t
get_os_thread_count
(threads::executor const &exec)¶ Return the number of worker OS- threads used by the given executor to execute HPX threads.
This function returns the number of cores used to execute HPX threads for the given executor. If the function is called while no HPX runtime system is active, it will return zero. If the executor is not valid, this function will fall back to retrieving the number of OS threads used by HPX.
- Parameters
exec
: [in] The executor to be used.
-
std::string
get_thread_name
()¶ Return the name of the calling thread.
This function returns the name of the calling thread. This name uniquely identifies the thread in the context of HPX. If the function is called while no HPX runtime system is active, the result will be “<unknown>”.
-
std::size_t
get_worker_thread_num
()¶ Return the number of the current OS-thread running in the runtime instance the current HPX-thread is executed with.
This function returns the zero based index of the OS-thread which executes the current HPX-thread.
- Note
- The returned value is zero based and its maximum value is smaller than the overall number of OS-threads executed (as returned by get_os_thread_count().
- Note
- This function needs to be executed on a HPX-thread. It will fail otherwise (it will return -1).
-
std::size_t
get_worker_thread_num
(error_code &ec)¶ Return the number of the current OS-thread running in the runtime instance the current HPX-thread is executed with.
This function returns the zero based index of the OS-thread which executes the current HPX-thread.
- Note
- The returned value is zero based and its maximum value is smaller than the overall number of OS-threads executed (as returned by get_os_thread_count(). It will return -1 if the current thread is not a known thread or if the runtime is not in running state.
- Note
- This function needs to be executed on a HPX-thread. It will fail otherwise (it will return -1).
- Parameters
ec
: [in,out] this represents the error status on exit.
-
void
report_error
(std::size_t num_thread, std::exception_ptr const &e)¶ The function report_error reports the given exception to the console.
-
void
report_error
(std::exception_ptr const &e)¶ The function report_error reports the given exception to the console.
-
char const *
get_runtime_mode_name
(runtime_mode state)¶ Get the readable string representing the name of the given runtime_mode constant.
-
runtime_mode
get_runtime_mode_from_name
(std::string const &mode)¶ Get the internal representation (runtime_mode constant) from the readable string representing the name.
-
parcel_write_handler_type
set_parcel_write_handler
(parcel_write_handler_type const &f)¶ Set the default parcel write handler which is invoked once a parcel has been sent if no explicit write handler was specified.
- Return
- The function returns the parcel write handler which was installed before this function was called.
- Note
- If no parcel handler function is registered by the user the system will call a default parcel handler function which is not performing any actions. However, this default function will terminate the application in case of any errors detected during preparing or sending the parcel.
- Parameters
f
: The new parcel write handler to use from this point on
-
void
register_pre_shutdown_function
(shutdown_function_type f)¶ Add a function to be executed by a HPX thread during hpx::finalize() but guaranteed before any shutdown function is executed (system-wide)
Any of the functions registered with register_pre_shutdown_function are guaranteed to be executed by an HPX thread during the execution of hpx::finalize() before any of the registered shutdown functions are executed (see: hpx::register_shutdown_function()).
- Note
- If this function is called while the pre-shutdown functions are being executed, or after that point, it will raise a invalid_status exception.
- See
- hpx::register_shutdown_function()
- Parameters
f
: [in] The function to be registered to run by an HPX thread as a pre-shutdown function.
-
void
register_shutdown_function
(shutdown_function_type f)¶ Add a function to be executed by a HPX thread during hpx::finalize() but guaranteed after any pre-shutdown function is executed (system-wide)
Any of the functions registered with register_shutdown_function are guaranteed to be executed by an HPX thread during the execution of hpx::finalize() after any of the registered pre-shutdown functions are executed (see: hpx::register_pre_shutdown_function()).
- Note
- If this function is called while the shutdown functions are being executed, or after that point, it will raise a invalid_status exception.
- See
- hpx::register_pre_shutdown_function()
- Parameters
f
: [in] The function to be registered to run by an HPX thread as a shutdown function.
-
void
register_pre_startup_function
(startup_function_type f)¶ Add a function to be executed by a HPX thread before hpx_main but guaranteed before any startup function is executed (system-wide).
Any of the functions registered with register_pre_startup_function are guaranteed to be executed by an HPX thread before any of the registered startup functions are executed (see hpx::register_startup_function()).
This function is one of the few API functions which can be called before the runtime system has been fully initialized. It will automatically stage the provided startup function to the runtime system during its initialization (if necessary).
- Note
- If this function is called while the pre-startup functions are being executed or after that point, it will raise a invalid_status exception.
- Parameters
f
: [in] The function to be registered to run by an HPX thread as a pre-startup function.
-
void
register_startup_function
(startup_function_type f)¶ Add a function to be executed by a HPX thread before hpx_main but guaranteed after any pre-startup function is executed (system-wide).
Any of the functions registered with register_startup_function are guaranteed to be executed by an HPX thread after any of the registered pre-startup functions are executed (see: hpx::register_pre_startup_function()), but before hpx_main is being called.
This function is one of the few API functions which can be called before the runtime system has been fully initialized. It will automatically stage the provided startup function to the runtime system during its initialization (if necessary).
- Note
- If this function is called while the startup functions are being executed or after that point, it will raise a invalid_status exception.
- Parameters
f
: [in] The function to be registered to run by an HPX thread as a startup function.
-
void
trigger_lco_event
(naming::id_type const &id, naming::address &&addr, bool move_credits = true)¶ Trigger the LCO referenced by the given id.
- Parameters
id
: [in] This represents the id of the LCO which should be triggered.addr
: [in] This represents the addr of the LCO which should be triggered.move_credits
: [in] If this is set to true then it is ok to send all credits in id along with the generated message. The default value is true.
-
void
trigger_lco_event
(naming::id_type const &id, bool move_credits = true)¶ Trigger the LCO referenced by the given id.
- Parameters
id
: [in] This represents the id of the LCO which should be triggered.move_credits
: [in] If this is set to true then it is ok to send all credits in id along with the generated message. The default value is true.
-
void
trigger_lco_event
(naming::id_type const &id, naming::address &&addr, naming::id_type const &cont, bool move_credits = true)¶ Trigger the LCO referenced by the given id.
- Parameters
id
: [in] This represents the id of the LCO which should be triggered.addr
: [in] This represents the addr of the LCO which should be triggered.cont
: [in] This represents the LCO to trigger after completion.move_credits
: [in] If this is set to true then it is ok to send all credits in id along with the generated message. The default value is true.
-
void
trigger_lco_event
(naming::id_type const &id, naming::id_type const &cont, bool move_credits = true)¶ Trigger the LCO referenced by the given id.
- Parameters
id
: [in] This represents the id of the LCO which should be triggered.cont
: [in] This represents the LCO to trigger after completion.move_credits
: [in] If this is set to true then it is ok to send all credits in id along with the generated message. The default value is true.
-
template<typename
Result
>
voidset_lco_value
(naming::id_type const &id, naming::address &&addr, Result &&t, bool move_credits = true)¶ Set the result value for the LCO referenced by the given id.
- Parameters
id
: [in] This represents the id of the LCO which should receive the given value.addr
: [in] This represents the addr of the LCO which should be triggered.t
: [in] This is the value which should be sent to the LCO.move_credits
: [in] If this is set to true then it is ok to send all credits in id along with the generated message. The default value is true.
-
template<typename
Result
>
std::enable_if<!std::is_same<typename util::decay<Result>::type, naming::address>::value>::typeset_lco_value
(naming::id_type const &id, Result &&t, bool move_credits = true)¶ Set the result value for the (managed) LCO referenced by the given id.
- Parameters
id
: [in] This represents the id of the LCO which should receive the given value.t
: [in] This is the value which should be sent to the LCO.move_credits
: [in] If this is set to true then it is ok to send all credits in id along with the generated message. The default value is true.
-
template<typename
Result
>
std::enable_if<!std::is_same<typename util::decay<Result>::type, naming::address>::value>::typeset_lco_value_unmanaged
(naming::id_type const &id, Result &&t, bool move_credits = true)¶ Set the result value for the (unmanaged) LCO referenced by the given id.
- Parameters
id
: [in] This represents the id of the LCO which should receive the given value.t
: [in] This is the value which should be sent to the LCO.move_credits
: [in] If this is set to true then it is ok to send all credits in id along with the generated message. The default value is true.
-
template<typename
Result
>
voidset_lco_value
(naming::id_type const &id, naming::address &&addr, Result &&t, naming::id_type const &cont, bool move_credits = true)¶ Set the result value for the LCO referenced by the given id.
- Parameters
id
: [in] This represents the id of the LCO which should receive the given value.addr
: [in] This represents the addr of the LCO which should be triggered.t
: [in] This is the value which should be sent to the LCO.cont
: [in] This represents the LCO to trigger after completion.move_credits
: [in] If this is set to true then it is ok to send all credits in id along with the generated message. The default value is true.
-
template<typename
Result
>
std::enable_if<!std::is_same<typename util::decay<Result>::type, naming::address>::value>::typeset_lco_value
(naming::id_type const &id, Result &&t, naming::id_type const &cont, bool move_credits = true)¶ Set the result value for the (managed) LCO referenced by the given id.
- Parameters
id
: [in] This represents the id of the LCO which should receive the given value.t
: [in] This is the value which should be sent to the LCO.cont
: [in] This represents the LCO to trigger after completion.move_credits
: [in] If this is set to true then it is ok to send all credits in id along with the generated message. The default value is true.
-
template<typename
Result
>
std::enable_if<!std::is_same<typename util::decay<Result>::type, naming::address>::value>::typeset_lco_value_unmanaged
(naming::id_type const &id, Result &&t, naming::id_type const &cont, bool move_credits = true)¶ Set the result value for the (unmanaged) LCO referenced by the given id.
- Parameters
id
: [in] This represents the id of the LCO which should receive the given value.t
: [in] This is the value which should be sent to the LCO.cont
: [in] This represents the LCO to trigger after completion.move_credits
: [in] If this is set to true then it is ok to send all credits in id along with the generated message. The default value is true.
-
void
set_lco_error
(naming::id_type const &id, naming::address &&addr, std::exception_ptr const &e, bool move_credits = true)¶ Set the error state for the LCO referenced by the given id.
- Parameters
id
: [in] This represents the id of the LCO which should receive the error value.addr
: [in] This represents the addr of the LCO which should be triggered.e
: [in] This is the error value which should be sent to the LCO.move_credits
: [in] If this is set to true then it is ok to send all credits in id along with the generated message. The default value is true.
-
void
set_lco_error
(naming::id_type const &id, naming::address &&addr, std::exception_ptr &&e, bool move_credits = true)¶ Set the error state for the LCO referenced by the given id.
- Parameters
id
: [in] This represents the id of the LCO which should receive the error value.addr
: [in] This represents the addr of the LCO which should be triggered.e
: [in] This is the error value which should be sent to the LCO.move_credits
: [in] If this is set to true then it is ok to send all credits in id along with the generated message. The default value is true.
-
void
set_lco_error
(naming::id_type const &id, std::exception_ptr const &e, bool move_credits = true)¶ Set the error state for the LCO referenced by the given id.
- Parameters
id
: [in] This represents the id of the LCO which should receive the error value.e
: [in] This is the error value which should be sent to the LCO.move_credits
: [in] If this is set to true then it is ok to send all credits in id along with the generated message. The default value is true.
-
void
set_lco_error
(naming::id_type const &id, std::exception_ptr &&e, bool move_credits = true)¶ Set the error state for the LCO referenced by the given id.
- Parameters
id
: [in] This represents the id of the LCO which should receive the error value.e
: [in] This is the error value which should be sent to the LCO.move_credits
: [in] If this is set to true then it is ok to send all credits in id along with the generated message. The default value is true.
-
void
set_lco_error
(naming::id_type const &id, naming::address &&addr, std::exception_ptr const &e, naming::id_type const &cont, bool move_credits = true)¶ Set the error state for the LCO referenced by the given id.
- Parameters
id
: [in] This represents the id of the LCO which should receive the error value.addr
: [in] This represents the addr of the LCO which should be triggered.e
: [in] This is the error value which should be sent to the LCO.cont
: [in] This represents the LCO to trigger after completion.move_credits
: [in] If this is set to true then it is ok to send all credits in id along with the generated message. The default value is true.
-
void
set_lco_error
(naming::id_type const &id, naming::address &&addr, std::exception_ptr &&e, naming::id_type const &cont, bool move_credits = true)¶ Set the error state for the LCO referenced by the given id.
- Parameters
id
: [in] This represents the id of the LCO which should receive the error value.addr
: [in] This represents the addr of the LCO which should be triggered.e
: [in] This is the error value which should be sent to the LCO.cont
: [in] This represents the LCO to trigger after completion.move_credits
: [in] If this is set to true then it is ok to send all credits in id along with the generated message. The default value is true.
-
void
set_lco_error
(naming::id_type const &id, std::exception_ptr const &e, naming::id_type const &cont, bool move_credits = true)¶ Set the error state for the LCO referenced by the given id.
- Parameters
id
: [in] This represents the id of the LCO which should receive the error value.e
: [in] This is the error value which should be sent to the LCO.cont
: [in] This represents the LCO to trigger after completion.move_credits
: [in] If this is set to true then it is ok to send all credits in id along with the generated message. The default value is true.
-
void
set_lco_error
(naming::id_type const &id, std::exception_ptr &&e, naming::id_type const &cont, bool move_credits = true)¶ Set the error state for the LCO referenced by the given id.
- Parameters
id
: [in] This represents the id of the LCO which should receive the error value.e
: [in] This is the error value which should be sent to the LCO.cont
: [in] This represents the LCO to trigger after completion.move_credits
: [in] If this is set to true then it is ok to send all credits in id along with the generated message. The default value is true.
-
template<typename Component, typename ... Ts><unspecified> hpx::new_(id_type const & locality, Ts &&... vs)
Create one or more new instances of the given Component type on the specified locality.
This function creates one or more new instances of the given Component type on the specified locality and returns a future object for the global address which can be used to reference the new component instance.
- Note
- This function requires to specify an explicit template argument which will define what type of component(s) to create, for instance:
hpx::future<hpx::id_type> f = hpx::new_<some_component>(hpx::find_here(), ...); hpx::id_type id = f.get();
- Return
- The function returns different types depending on its use:
- If the explicit template argument Component represents a component type (
traits::is_component<Component>::value
evaluates to true), the function will return an hpx::future object instance which can be used to retrieve the global address of the newly created component. - If the explicit template argument Component represents a client side object (
traits::is_client<Component>::value
evaluates to true), the function will return a new instance of that type which can be used to refer to the newly created component instance.
- If the explicit template argument Component represents a component type (
- Parameters
locality
: [in] The global address of the locality where the new instance should be created on.vs
: [in] Any number of arbitrary arguments (passed by value, by const reference or by rvalue reference) which will be forwarded to the constructor of the created component instance.
-
template<typename Component, typename ... Ts><unspecified> hpx::local_new(Ts &&... vs)
Create one new instance of the given Component type on the current locality.
This function creates one new instance of the given Component type on the current locality and returns a future object for the global address which can be used to reference the new component instance.
- Note
- This function requires to specify an explicit template argument which will define what type of component(s) to create, for instance:
hpx::future<hpx::id_type> f = hpx::local_new<some_component>(...); hpx::id_type id = f.get();
- Return
- The function returns different types depending on its use:
- If the explicit template argument Component represents a component type (
traits::is_component<Component>::value
evaluates to true), the function will return an hpx::future object instance which can be used to retrieve the global address of the newly created component. If the first argument ishpx::launch::sync
the function will directly return anhpx::id_type
. - If the explicit template argument Component represents a client side object (
traits::is_client<Component>::value
evaluates to true), the function will return a new instance of that type which can be used to refer to the newly created component instance.
- If the explicit template argument Component represents a component type (
- Note
- The difference of this funtion to hpx::new_ is that it can be used in cases where the supplied arguments are non-copyable and non-movable. All operations are guaranteed to be local only.
- Parameters
vs
: [in] Any number of arbitrary arguments (passed by value, by const reference or by rvalue reference) which will be forwarded to the constructor of the created component instance.
-
template<typename Component, typename ... Ts><unspecified> hpx::new_(id_type const & locality, std::size_t count, Ts &&... vs)
Create multiple new instances of the given Component type on the specified locality.
This function creates multiple new instances of the given Component type on the specified locality and returns a future object for the global address which can be used to reference the new component instance.
- Note
- This function requires to specify an explicit template argument which will define what type of component(s) to create, for instance:
hpx::future<std::vector<hpx::id_type> > f = hpx::new_<some_component[]>(hpx::find_here(), 10, ...); hpx::id_type id = f.get();
- Return
- The function returns different types depending on its use:
- If the explicit template argument Component represents an array of a component type (i.e. Component[], where
traits::is_component<Component>::value
evaluates to true), the function will return an hpx::future object instance which holds a std::vector<hpx::id_type>, where each of the items in this vector is a global address of one of the newly created components. - If the explicit template argument Component represents an array of a client side object type (i.e. Component[], where
traits::is_client<Component>::value
evaluates to true), the function will return an hpx::future object instance which holds a std::vector<hpx::id_type>, where each of the items in this vector is a client side instance of the given type, each representing one of the newly created components.
- If the explicit template argument Component represents an array of a component type (i.e. Component[], where
- Parameters
locality
: [in] The global address of the locality where the new instance should be created on.count
: [in] The number of component instances to createvs
: [in] Any number of arbitrary arguments (passed by value, by const reference or by rvalue reference) which will be forwarded to the constructor of the created component instance.
-
template<typename Component, typename DistPolicy, typename ... Ts><unspecified> hpx::new_(DistPolicy const & policy, Ts &&... vs)
Create one or more new instances of the given Component type based on the given distribution policy.
This function creates one or more new instances of the given Component type on the localities defined by the given distribution policy and returns a future object for global address which can be used to reference the new component instance(s).
- Note
- This function requires to specify an explicit template argument which will define what type of component(s) to create, for instance:
hpx::future<hpx::id_type> f = hpx::new_<some_component>(hpx::default_layout, ...); hpx::id_type id = f.get();
- Return
- The function returns different types depending on its use:
- If the explicit template argument Component represents a component type (
traits::is_component<Component>::value
evaluates to true), the function will return an hpx::future object instance which can be used to retrieve the global address of the newly created component. - If the explicit template argument Component represents a client side object (
traits::is_client<Component>::value
evaluates to true), the function will return a new instance of that type which can be used to refer to the newly created component instance.
- If the explicit template argument Component represents a component type (
- Parameters
policy
: [in] The distribution policy used to decide where to place the newly created.vs
: [in] Any number of arbitrary arguments (passed by value, by const reference or by rvalue reference) which will be forwarded to the constructor of the created component instance.
-
template<typename Component, typename DistPolicy, typename ... Ts><unspecified> hpx::new_(DistPolicy const & policy, std::size_t count, Ts &&... vs)
Create multiple new instances of the given Component type on the localities as defined by the given distribution policy.
This function creates multiple new instances of the given Component type on the localities defined by the given distribution policy and returns a future object for the global address which can be used to reference the new component instance.
- Note
- This function requires to specify an explicit template argument which will define what type of component(s) to create, for instance:
hpx::future<std::vector<hpx::id_type> > f = hpx::new_<some_component[]>(hpx::default_layout, 10, ...); hpx::id_type id = f.get();
- Return
- The function returns different types depending on its use:
- If the explicit template argument Component represents an array of a component type (i.e. Component[], where
traits::is_component<Component>::value
evaluates to true), the function will return an hpx::future object instance which holds a std::vector<hpx::id_type>, where each of the items in this vector is a global address of one of the newly created components. - If the explicit template argument Component represents an array of a client side object type (i.e. Component[], where
traits::is_client<Component>::value
evaluates to true), the function will return an hpx::future object instance which holds a std::vector<hpx::id_type>, where each of the items in this vector is a client side instance of the given type, each representing one of the newly created components.
- If the explicit template argument Component represents an array of a component type (i.e. Component[], where
- Parameters
policy
: [in] The distribution policy used to decide where to place the newly created.count
: [in] The number of component instances to createvs
: [in] Any number of arbitrary arguments (passed by value, by const reference or by rvalue reference) which will be forwarded to the constructor of the created component instance.
-
template<typename ...
Ts
>
tuple<future<Ts>...>split_future
(future<tuple<Ts...>> &&f)¶ The function split_future is an operator allowing to split a given future of a sequence of values (any tuple, std::pair, or std::array) into an equivalent container of futures where each future represents one of the values from the original future. In some sense this function provides the inverse operation of when_all.
- Return
- Returns an equivalent container (same container type as passed as the argument) of futures, where each future refers to the corresponding value in the input parameter. All of the returned futures become ready once the input future has become ready. If the input future is exceptional, all output futures will be exceptional as well.
- Note
- The following cases are special: here the returned futures are directly representing the futures which were passed to the function.
tuple<future<void> > split_future(future<tuple<> > && f); array<future<void>, 1> split_future(future<array<T, 0> > && f);
- Parameters
f
: [in] A future holding an arbitrary sequence of values stored in a tuple-like container. This facility supports hpx::util::tuple<>, std::pair<T1, T2>, and std::array<T, N>
-
template<typename
T
>
std::vector<future<T>>split_future
(future<std::vector<T>> &&f, std::size_t size)¶ The function split_future is an operator allowing to split a given future of a sequence of values (any std::vector) into a std::vector of futures where each future represents one of the values from the original std::vector. In some sense this function provides the inverse operation of when_all.
- Return
- Returns a std::vector of futures, where each future refers to the corresponding value in the input parameter. All of the returned futures become ready once the input future has become ready. If the input future is exceptional, all output futures will be exceptional as well.
- Parameters
f
: [in] A future holding an arbitrary sequence of values stored in a std::vector.size
: [in] The number of elements the vector will hold once the input future has become ready
-
template<typename
InputIter
>
voidwait_all
(InputIter first, InputIter last)¶ The function wait_all is an operator allowing to join on the result of all given futures. It AND-composes all future objects given and returns after they finished executing.
- Note
- The function wait_all returns after all futures have become ready. All input futures are still valid after wait_all returns.
- Parameters
first
: The iterator pointing to the first element of a sequence of future or shared_future objects for which wait_all should wait.last
: The iterator pointing to the last element of a sequence of future or shared_future objects for which wait_all should wait.
-
template<typename
R
>
voidwait_all
(std::vector<future<R>> &&futures)¶ The function wait_all is an operator allowing to join on the result of all given futures. It AND-composes all future objects given and returns after they finished executing.
- Note
- The function wait_all returns after all futures have become ready. All input futures are still valid after wait_all returns.
- Parameters
futures
: A vector or array holding an arbitrary amount of future or shared_future objects for which wait_all should wait.
-
template<typename
R
, std::size_tN
>
voidwait_all
(std::array<future<R>, N> &&futures)¶ The function wait_all is an operator allowing to join on the result of all given futures. It AND-composes all future objects given and returns after they finished executing.
- Note
- The function wait_all returns after all futures have become ready. All input futures are still valid after wait_all returns.
- Parameters
futures
: A vector or array holding an arbitrary amount of future or shared_future objects for which wait_all should wait.
-
template<typename ...
T
>
voidwait_all
(T&&... futures)¶ The function wait_all is an operator allowing to join on the result of all given futures. It AND-composes all future objects given and returns after they finished executing.
- Note
- The function wait_all returns after all futures have become ready. All input futures are still valid after wait_all returns.
- Parameters
futures
: An arbitrary number of future or shared_future objects, possibly holding different types for which wait_all should wait.
-
template<typename
InputIter
>
InputIterwait_all_n
(InputIter begin, std::size_t count)¶ The function wait_all_n is an operator allowing to join on the result of all given futures. It AND-composes all future objects given and returns after they finished executing.
- Return
- The function wait_all_n will return an iterator referring to the first element in the input sequence after the last processed element.
- Note
- The function wait_all_n returns after all futures have become ready. All input futures are still valid after wait_all_n returns.
- Parameters
begin
: The iterator pointing to the first element of a sequence of future or shared_future objects for which wait_all_n should wait.count
: The number of elements in the sequence starting at first.
-
template<typename
InputIter
, typenameContainer
= vector<future<typename std::iterator_traits<InputIter>::value_type>>>
future<Container>when_all
(InputIter first, InputIter last)¶ The function when_all is an operator allowing to join on the result of all given futures. It AND-composes all future objects given and returns a new future object representing the same list of futures after they finished executing.
- Return
- Returns a future holding the same list of futures as has been passed to when_all.
- future<Container<future<R>>>: If the input cardinality is unknown at compile time and the futures are all of the same type. The order of the futures in the output container will be the same as given by the input iterator.
- Note
- Calling this version of when_all where first == last, returns a future with an empty container that is immediately ready. Each future and shared_future is waited upon and then copied into the collection of the output (returned) future, maintaining the order of the futures in the input collection. The future returned by when_all will not throw an exception, but the futures held in the output collection may.
- Parameters
first
: [in] The iterator pointing to the first element of a sequence of future or shared_future objects for which when_all should wait.last
: [in] The iterator pointing to the last element of a sequence of future or shared_future objects for which when_all should wait.
-
template<typename
Range
>
future<Range>when_all
(Range &&values)¶ The function when_all is an operator allowing to join on the result of all given futures. It AND-composes all future objects given and returns a new future object representing the same list of futures after they finished executing.
- Return
- Returns a future holding the same list of futures as has been passed to when_all.
- future<Container<future<R>>>: If the input cardinality is unknown at compile time and the futures are all of the same type.
- Note
- Calling this version of when_all where the input container is empty, returns a future with an empty container that is immediately ready. Each future and shared_future is waited upon and then copied into the collection of the output (returned) future, maintaining the order of the futures in the input collection. The future returned by when_all will not throw an exception, but the futures held in the output collection may.
- Parameters
values
: [in] A range holding an arbitrary amount of future or shared_future objects for which when_all should wait.
-
template<typename ...
T
>
future<tuple<future<T>...>>when_all
(T&&... futures)¶ The function when_all is an operator allowing to join on the result of all given futures. It AND-composes all future objects given and returns a new future object representing the same list of futures after they finished executing.
- Return
- Returns a future holding the same list of futures as has been passed to when_all.
- future<tuple<future<T0>, future<T1>, future<T2>…>>: If inputs are fixed in number and are of heterogeneous types. The inputs can be any arbitrary number of future objects.
- future<tuple<>> if when_all is called with zero arguments. The returned future will be initially ready.
- Note
- Each future and shared_future is waited upon and then copied into the collection of the output (returned) future, maintaining the order of the futures in the input collection. The future returned by when_all will not throw an exception, but the futures held in the output collection may.
- Parameters
futures
: [in] An arbitrary number of future or shared_future objects, possibly holding different types for which when_all should wait.
-
template<typename
InputIter
, typenameContainer
= vector<future<typename std::iterator_traits<InputIter>::value_type>>>
future<Container>when_all_n
(InputIter begin, std::size_t count)¶ The function when_all_n is an operator allowing to join on the result of all given futures. It AND-composes all future objects given and returns a new future object representing the same list of futures after they finished executing.
- Return
- Returns a future holding the same list of futures as has been passed to when_all_n.
- future<Container<future<R>>>: If the input cardinality is unknown at compile time and the futures are all of the same type. The order of the futures in the output vector will be the same as given by the input iterator.
- Note
- As long as ec is not pre-initialized to hpx::throws this function doesn’t throw but returns the result code using the parameter ec. Otherwise it throws an instance of hpx::exception.
- Note
- None of the futures in the input sequence are invalidated.
- Parameters
begin
: [in] The iterator pointing to the first element of a sequence of future or shared_future objects for which wait_all_n should wait.count
: [in] The number of elements in the sequence starting at first.
- Exceptions
This
: function will throw errors which are encountered while setting up the requested operation only. Errors encountered while executing the operations delivering the results to be stored in the futures are reported through the futures themselves.
-
template<typename
InputIter
>
voidwait_any
(InputIter first, InputIter last, error_code &ec = throws)¶ The function wait_any is a non-deterministic choice operator. It OR-composes all future objects given and returns after one future of that list finishes execution.
- Note
- The function wait_any returns after at least one future has become ready. All input futures are still valid after wait_any returns.
- Note
- As long as ec is not pre-initialized to hpx::throws this function doesn’t throw but returns the result code using the parameter ec. Otherwise it throws an instance of hpx::exception.
- Note
- None of the futures in the input sequence are invalidated.
- Parameters
first
: [in] The iterator pointing to the first element of a sequence of future or shared_future objects for which wait_any should wait.last
: [in] The iterator pointing to the last element of a sequence of future or shared_future objects for which wait_any should wait.ec
: [in,out] this represents the error status on exit, if this is pre-initialized to hpx::throws the function will throw on error instead.
-
template<typename
R
>
voidwait_any
(std::vector<future<R>> &futures, error_code &ec = throws)¶ The function wait_any is a non-deterministic choice operator. It OR-composes all future objects given and returns after one future of that list finishes execution.
- Note
- The function wait_any returns after at least one future has become ready. All input futures are still valid after wait_any returns.
- Note
- As long as ec is not pre-initialized to hpx::throws this function doesn’t throw but returns the result code using the parameter ec. Otherwise it throws an instance of hpx::exception.
- Note
- None of the futures in the input sequence are invalidated.
- Parameters
futures
: [in] A vector holding an arbitrary amount of future or shared_future objects for which wait_any should wait.ec
: [in,out] this represents the error status on exit, if this is pre-initialized to hpx::throws the function will throw on error instead.
-
template<typename R, std:;size_t N>void hpx::wait_any(std::array< future< R >, N > & futures, error_code & ec = throws)
The function wait_any is a non-deterministic choice operator. It OR-composes all future objects given and returns after one future of that list finishes execution.
- Note
- The function wait_any returns after at least one future has become ready. All input futures are still valid after wait_any returns.
- Note
- As long as ec is not pre-initialized to hpx::throws this function doesn’t throw but returns the result code using the parameter ec. Otherwise it throws an instance of hpx::exception.
- Note
- None of the futures in the input sequence are invalidated.
- Parameters
futures
: [in] Amn array holding an arbitrary amount of future or shared_future objects for which wait_any should wait.ec
: [in,out] this represents the error status on exit, if this is pre-initialized to hpx::throws the function will throw on error instead.
-
template<typename ...
T
>
voidwait_any
(error_code &ec, T&&... futures)¶ The function wait_any is a non-deterministic choice operator. It OR-composes all future objects given and returns after one future of that list finishes execution.
- Note
- The function wait_any returns after at least one future has become ready. All input futures are still valid after wait_any returns.
- Note
- As long as ec is not pre-initialized to hpx::throws this function doesn’t throw but returns the result code using the parameter ec. Otherwise it throws an instance of hpx::exception.
- Note
- None of the futures in the input sequence are invalidated.
- Parameters
futures
: [in] An arbitrary number of future or shared_future objects, possibly holding different types for which wait_any should wait.ec
: [in,out] this represents the error status on exit, if this is pre-initialized to hpx::throws the function will throw on error instead.
-
template<typename ...
T
>
voidwait_any
(T&&... futures)¶ The function wait_any is a non-deterministic choice operator. It OR-composes all future objects given and returns after one future of that list finishes execution.
- Note
- The function wait_any returns after at least one future has become ready. All input futures are still valid after wait_any returns.
- Note
- None of the futures in the input sequence are invalidated.
- Parameters
futures
: [in] An arbitrary number of future or shared_future objects, possibly holding different types for which wait_any should wait.
-
template<typename
InputIter
>
InputIterwait_any_n
(InputIter first, std::size_t count, error_code &ec = throws)¶ The function wait_any_n is a non-deterministic choice operator. It OR-composes all future objects given and returns after one future of that list finishes execution.
- Note
- The function wait_any_n returns after at least one future has become ready. All input futures are still valid after wait_any_n returns.
- Return
- The function wait_all_n will return an iterator referring to the first element in the input sequence after the last processed element.
- Note
- As long as ec is not pre-initialized to hpx::throws this function doesn’t throw but returns the result code using the parameter ec. Otherwise it throws an instance of hpx::exception.
- Note
- None of the futures in the input sequence are invalidated.
- Parameters
first
: [in] The iterator pointing to the first element of a sequence of future or shared_future objects for which wait_any_n should wait.count
: [in] The number of elements in the sequence starting at first.ec
: [in,out] this represents the error status on exit, if this is pre-initialized to hpx::throws the function will throw on error instead.
-
template<typename
InputIter
, typenameContainer
= vector<future<typename std::iterator_traits<InputIter>::value_type>>>
future<when_any_result<Container>>when_any
(InputIter first, InputIter last)¶ The function when_any is a non-deterministic choice operator. It OR-composes all future objects given and returns a new future object representing the same list of futures after one future of that list finishes execution.
- Return
- Returns a when_any_result holding the same list of futures as has been passed to when_any and an index pointing to a ready future.
- future<when_any_result<Container<future<R>>>>: If the input cardinality is unknown at compile time and the futures are all of the same type. The order of the futures in the output container will be the same as given by the input iterator.
- Parameters
first
: [in] The iterator pointing to the first element of a sequence of future or shared_future objects for which when_any should wait.last
: [in] The iterator pointing to the last element of a sequence of future or shared_future objects for which when_any should wait.
-
template<typename
Range
>
future<when_any_result<Range>>when_any
(Range &values)¶ The function when_any is a non-deterministic choice operator. It OR-composes all future objects given and returns a new future object representing the same list of futures after one future of that list finishes execution.
- Return
- Returns a when_any_result holding the same list of futures as has been passed to when_any and an index pointing to a ready future.
- future<when_any_result<Container<future<R>>>>: If the input cardinality is unknown at compile time and the futures are all of the same type. The order of the futures in the output container will be the same as given by the input iterator.
- Parameters
values
: [in] A range holding an arbitrary amount of futures or shared_future objects for which when_any should wait.
-
template<typename ...
T
>
future<when_any_result<tuple<future<T>...>>>when_any
(T&&... futures)¶ The function when_any is a non-deterministic choice operator. It OR-composes all future objects given and returns a new future object representing the same list of futures after one future of that list finishes execution.
- Return
- Returns a when_any_result holding the same list of futures as has been passed to when_any and an index pointing to a ready future..
- future<when_any_result<tuple<future<T0>, future<T1>…>>>: If inputs are fixed in number and are of heterogeneous types. The inputs can be any arbitrary number of future objects.
- future<when_any_result<tuple<>>> if when_any is called with zero arguments. The returned future will be initially ready.
- Parameters
futures
: [in] An arbitrary number of future or shared_future objects, possibly holding different types for which when_any should wait.
-
template<typename
InputIter
, typenameContainer
= vector<future<typename std::iterator_traits<InputIter>::value_type>>>
future<when_any_result<Container>>when_any_n
(InputIter first, std::size_t count)¶ The function when_any_n is a non-deterministic choice operator. It OR-composes all future objects given and returns a new future object representing the same list of futures after one future of that list finishes execution.
- Return
- Returns a when_any_result holding the same list of futures as has been passed to when_any and an index pointing to a ready future.
- future<when_any_result<Container<future<R>>>>: If the input cardinality is unknown at compile time and the futures are all of the same type. The order of the futures in the output container will be the same as given by the input iterator.
- Note
- None of the futures in the input sequence are invalidated.
- Parameters
first
: [in] The iterator pointing to the first element of a sequence of future or shared_future objects for which when_any_n should wait.count
: [in] The number of elements in the sequence starting at first.
-
template<typename
InputIter
>
future<vector<future<typename std::iterator_traits<InputIter>::value_type>>>wait_some
(std::size_t n, Iterator first, Iterator last, error_code &ec = throws)¶ The function wait_some is an operator allowing to join on the result of all given futures. It AND-composes all future objects given and returns a new future object representing the same list of futures after n of them finished executing.
- Note
- The future returned by the function wait_some becomes ready when at least n argument futures have become ready.
- Return
- Returns a future holding the same list of futures as has been passed to wait_some.
- future<vector<future<R>>>: If the input cardinality is unknown at compile time and the futures are all of the same type.
- Note
- Calling this version of wait_some where first == last, returns a future with an empty vector that is immediately ready. Each future and shared_future is waited upon and then copied into the collection of the output (returned) future, maintaining the order of the futures in the input collection. The future returned by wait_some will not throw an exception, but the futures held in the output collection may.
- Parameters
n
: [in] The number of futures out of the arguments which have to become ready in order for the returned future to get ready.first
: [in] The iterator pointing to the first element of a sequence of future or shared_future objects for which when_all should wait.last
: [in] The iterator pointing to the last element of a sequence of future or shared_future objects for which when_all should wait.ec
: [in,out] this represents the error status on exit, if this is pre-initialized to hpx::throws the function will throw on error instead.
-
template<typename
R
>
voidwait_some
(std::size_t n, std::vector<future<R>> &&futures, error_code &ec = throws)¶ The function wait_some is an operator allowing to join on the result of all given futures. It AND-composes all future objects given and returns a new future object representing the same list of futures after n of them finished executing.
- Note
- The function wait_all returns after n futures have become ready. All input futures are still valid after wait_all returns.
- Note
- Each future and shared_future is waited upon and then copied into the collection of the output (returned) future, maintaining the order of the futures in the input collection. The future returned by wait_some will not throw an exception, but the futures held in the output collection may.
- Parameters
n
: [in] The number of futures out of the arguments which have to become ready in order for the returned future to get ready.futures
: [in] A vector holding an arbitrary amount of future or shared_future objects for which wait_some should wait.ec
: [in,out] this represents the error status on exit, if this is pre-initialized to hpx::throws the function will throw on error instead.
-
template<typename
R
, std::size_tN
>
voidwait_some
(std::size_t n, std::array<future<R>, N> &&futures, error_code &ec = throws)¶ The function wait_some is an operator allowing to join on the result of all given futures. It AND-composes all future objects given and returns a new future object representing the same list of futures after n of them finished executing.
- Note
- The function wait_all returns after n futures have become ready. All input futures are still valid after wait_all returns.
- Note
- Each future and shared_future is waited upon and then copied into the collection of the output (returned) future, maintaining the order of the futures in the input collection. The future returned by wait_some will not throw an exception, but the futures held in the output collection may.
- Parameters
n
: [in] The number of futures out of the arguments which have to become ready in order for the returned future to get ready.futures
: [in] An array holding an arbitrary amount of future or shared_future objects for which wait_some should wait.ec
: [in,out] this represents the error status on exit, if this is pre-initialized to hpx::throws the function will throw on error instead.
-
template<typename ...
T
>
voidwait_some
(std::size_t n, T&&... futures, error_code &ec = throws)¶ The function wait_some is an operator allowing to join on the result of all given futures. It AND-composes all future objects given and returns a new future object representing the same list of futures after n of them finished executing.
- Note
- The function wait_all returns after n futures have become ready. All input futures are still valid after wait_all returns.
- Note
- Calling this version of wait_some where first == last, returns a future with an empty vector that is immediately ready. Each future and shared_future is waited upon and then copied into the collection of the output (returned) future, maintaining the order of the futures in the input collection. The future returned by wait_some will not throw an exception, but the futures held in the output collection may.
- Parameters
n
: [in] The number of futures out of the arguments which have to become ready in order for the returned future to get ready.futures
: [in] An arbitrary number of future or shared_future objects, possibly holding different types for which wait_some should wait.ec
: [in,out] this represents the error status on exit, if this is pre-initialized to hpx::throws the function will throw on error instead.
-
template<typename
InputIter
>
InputIterwait_some_n
(std::size_t n, Iterator first, std::size_t count, error_code &ec = throws)¶ The function wait_some_n is an operator allowing to join on the result of all given futures. It AND-composes all future objects given and returns a new future object representing the same list of futures after n of them finished executing.
- Note
- The function wait_all returns after n futures have become ready. All input futures are still valid after wait_all returns.
- Return
- This function returns an Iterator referring to the first element after the last processed input element.
- Note
- Calling this version of wait_some_n where count == 0, returns a future with the same elements as the arguments that is immediately ready. Possibly none of the futures in that vector are ready. Each future and shared_future is waited upon and then copied into the collection of the output (returned) future, maintaining the order of the futures in the input collection. The future returned by wait_some_n will not throw an exception, but the futures held in the output collection may.
- Parameters
n
: [in] The number of futures out of the arguments which have to become ready in order for the returned future to get ready.first
: [in] The iterator pointing to the first element of a sequence of future or shared_future objects for which when_all should wait.count
: [in] The number of elements in the sequence starting at first.ec
: [in,out] this represents the error status on exit, if this is pre-initialized to hpx::throws the function will throw on error instead.
-
template<typename
InputIter
, typenameContainer
= vector<future<typename std::iterator_traits<InputIter>::value_type>>>
future<when_some_result<Container>>when_some
(std::size_t n, Iterator first, Iterator last, error_code &ec = throws)¶ The function when_some is an operator allowing to join on the result of all given futures. It AND-composes all future objects given and returns a new future object representing the same list of futures after n of them finished executing.
- Note
- The future returned by the function when_some becomes ready when at least n argument futures have become ready.
- Return
- Returns a when_some_result holding the same list of futures as has been passed to when_some and indices pointing to ready futures.
- future<when_some_result<Container<future<R>>>>: If the input cardinality is unknown at compile time and the futures are all of the same type. The order of the futures in the output container will be the same as given by the input iterator.
- Note
- Calling this version of when_some where first == last, returns a future with an empty container that is immediately ready. Each future and shared_future is waited upon and then copied into the collection of the output (returned) future, maintaining the order of the futures in the input collection. The future returned by when_some will not throw an exception, but the futures held in the output collection may.
- Parameters
n
: [in] The number of futures out of the arguments which have to become ready in order for the returned future to get ready.first
: [in] The iterator pointing to the first element of a sequence of future or shared_future objects for which when_all should wait.last
: [in] The iterator pointing to the last element of a sequence of future or shared_future objects for which when_all should wait.ec
: [in,out] this represents the error status on exit, if this is pre-initialized to hpx::throws the function will throw on error instead.
-
template<typename
Range
>
future<when_some_result<Range>>when_some
(std::size_t n, Range &&futures, error_code &ec = throws)¶ The function when_some is an operator allowing to join on the result of all given futures. It AND-composes all future objects given and returns a new future object representing the same list of futures after n of them finished executing.
- Note
- The future returned by the function when_some becomes ready when at least n argument futures have become ready.
- Return
- Returns a when_some_result holding the same list of futures as has been passed to when_some and indices pointing to ready futures.
- future<when_some_result<Container<future<R>>>>: If the input cardinality is unknown at compile time and the futures are all of the same type. The order of the futures in the output container will be the same as given by the input iterator.
- Note
- Each future and shared_future is waited upon and then copied into the collection of the output (returned) future, maintaining the order of the futures in the input collection. The future returned by when_some will not throw an exception, but the futures held in the output collection may.
- Parameters
n
: [in] The number of futures out of the arguments which have to become ready in order for the returned future to get ready.futures
: [in] A container holding an arbitrary amount of future or shared_future objects for which when_some should wait.ec
: [in,out] this represents the error status on exit, if this is pre-initialized to hpx::throws the function will throw on error instead.
-
template<typename ...
T
>
future<when_some_result<tuple<future<T>...>>>when_some
(std::size_t n, error_code &ec, T&&... futures)¶ The function when_some is an operator allowing to join on the result of all given futures. It AND-composes all future objects given and returns a new future object representing the same list of futures after n of them finished executing.
- Note
- The future returned by the function when_some becomes ready when at least n argument futures have become ready.
- Return
- Returns a when_some_result holding the same list of futures as has been passed to when_some and an index pointing to a ready future..
- future<when_some_result<tuple<future<T0>, future<T1>…>>>: If inputs are fixed in number and are of heterogeneous types. The inputs can be any arbitrary number of future objects.
- future<when_some_result<tuple<>>> if when_some is called with zero arguments. The returned future will be initially ready.
- Note
- Each future and shared_future is waited upon and then copied into the collection of the output (returned) future, maintaining the order of the futures in the input collection. The future returned by when_some will not throw an exception, but the futures held in the output collection may.
- Parameters
n
: [in] The number of futures out of the arguments which have to become ready in order for the returned future to get ready.ec
: [in,out] this represents the error status on exit, if this is pre-initialized to hpx::throws the function will throw on error instead.futures
: [in] An arbitrary number of future or shared_future objects, possibly holding different types for which when_some should wait.
-
template<typename ...
T
>
future<when_some_result<tuple<future<T>...>>>when_some
(std::size_t n, T&&... futures)¶ The function when_some is an operator allowing to join on the result of all given futures. It AND-composes all future objects given and returns a new future object representing the same list of futures after n of them finished executing.
- Note
- The future returned by the function when_some becomes ready when at least n argument futures have become ready.
- Return
- Returns a when_some_result holding the same list of futures as has been passed to when_some and an index pointing to a ready future..
- future<when_some_result<tuple<future<T0>, future<T1>…>>>: If inputs are fixed in number and are of heterogeneous types. The inputs can be any arbitrary number of future objects.
- future<when_some_result<tuple<>>> if when_some is called with zero arguments. The returned future will be initially ready.
- Note
- Each future and shared_future is waited upon and then copied into the collection of the output (returned) future, maintaining the order of the futures in the input collection. The future returned by when_some will not throw an exception, but the futures held in the output collection may.
- Parameters
n
: [in] The number of futures out of the arguments which have to become ready in order for the returned future to get ready.futures
: [in] An arbitrary number of future or shared_future objects, possibly holding different types for which when_some should wait.
-
template<typename
InputIter
, typenameContainer
= vector<future<typename std::iterator_traits<InputIter>::value_type>>>
future<when_some_result<Container>>when_some_n
(std::size_t n, Iterator first, std::size_t count, error_code &ec = throws)¶ The function when_some_n is an operator allowing to join on the result of all given futures. It AND-composes all future objects given and returns a new future object representing the same list of futures after n of them finished executing.
- Note
- The future returned by the function when_some_n becomes ready when at least n argument futures have become ready.
- Return
- Returns a when_some_result holding the same list of futures as has been passed to when_some and indices pointing to ready futures.
- future<when_some_result<Container<future<R>>>>: If the input cardinality is unknown at compile time and the futures are all of the same type. The order of the futures in the output container will be the same as given by the input iterator.
- Note
- Calling this version of when_some_n where count == 0, returns a future with the same elements as the arguments that is immediately ready. Possibly none of the futures in that container are ready. Each future and shared_future is waited upon and then copied into the collection of the output (returned) future, maintaining the order of the futures in the input collection. The future returned by when_some_n will not throw an exception, but the futures held in the output collection may.
- Parameters
n
: [in] The number of futures out of the arguments which have to become ready in order for the returned future to get ready.first
: [in] The iterator pointing to the first element of a sequence of future or shared_future objects for which when_all should wait.count
: [in] The number of elements in the sequence starting at first.ec
: [in,out] this represents the error status on exit, if this is pre-initialized to hpx::throws the function will throw on error instead.
-
template<typename
F
, typenameFuture
>
voidwait_each
(F &&f, std::vector<Future> &&futures)¶ The function wait_each is an operator allowing to join on the results of all given futures. It AND-composes all future objects given and returns after they finished executing. Additionally, the supplied function is called for each of the passed futures as soon as the future has become ready. wait_each returns after all futures have been become ready.
- Note
- This function consumes the futures as they are passed on to the supplied function. The callback should take one or two parameters, namely either a future to be processed or a type that std::size_t is implicitly convertible to as the first parameter and the future as the second parameter. The first parameter will correspond to the index of the current future in the collection.
- Parameters
f
: The function which will be called for each of the input futures once the future has become ready.futures
: A vector holding an arbitrary amount of future or shared_future objects for which wait_each should wait.
-
template<typename
F
, typenameIterator
>
voidwait_each
(F &&f, Iterator begin, Iterator end)¶ The function wait_each is an operator allowing to join on the results of all given futures. It AND-composes all future objects given and returns after they finished executing. Additionally, the supplied function is called for each of the passed futures as soon as the future has become ready. wait_each returns after all futures have been become ready.
- Note
- This function consumes the futures as they are passed on to the supplied function. The callback should take one or two parameters, namely either a future to be processed or a type that std::size_t is implicitly convertible to as the first parameter and the future as the second parameter. The first parameter will correspond to the index of the current future in the collection.
- Parameters
f
: The function which will be called for each of the input futures once the future has become ready.begin
: The iterator pointing to the first element of a sequence of future or shared_future objects for which wait_each should wait.end
: The iterator pointing to the last element of a sequence of future or shared_future objects for which wait_each should wait.
-
template<typename
F
, typename ...T
>
voidwait_each
(F &&f, T&&... futures)¶ The function wait_each is an operator allowing to join on the results of all given futures. It AND-composes all future objects given and returns after they finished executing. Additionally, the supplied function is called for each of the passed futures as soon as the future has become ready. wait_each returns after all futures have been become ready.
- Note
- This function consumes the futures as they are passed on to the supplied function. The callback should take one or two parameters, namely either a future to be processed or a type that std::size_t is implicitly convertible to as the first parameter and the future as the second parameter. The first parameter will correspond to the index of the current future in the collection.
- Parameters
f
: The function which will be called for each of the input futures once the future has become ready.futures
: An arbitrary number of future or shared_future objects, possibly holding different types for which wait_each should wait.
-
template<typename
F
, typenameIterator
>
voidwait_each_n
(F &&f, Iterator begin, std::size_t count)¶ The function wait_each is an operator allowing to join on the result of all given futures. It AND-composes all future objects given and returns after they finished executing. Additionally, the supplied function is called for each of the passed futures as soon as the future has become ready.
- Note
- This function consumes the futures as they are passed on to the supplied function. The callback should take one or two parameters, namely either a future to be processed or a type that std::size_t is implicitly convertible to as the first parameter and the future as the second parameter. The first parameter will correspond to the index of the current future in the collection.
- Parameters
f
: The function which will be called for each of the input futures once the future has become ready.begin
: The iterator pointing to the first element of a sequence of future or shared_future objects for which wait_each_n should wait.count
: The number of elements in the sequence starting at first.
-
template<typename
F
, typenameFuture
>
future<void>when_each
(F &&f, std::vector<Future> &&futures)¶ The function when_each is an operator allowing to join on the results of all given futures. It AND-composes all future objects given and returns a new future object representing the event of all those futures having finished executing. It also calls the supplied callback for each of the futures which becomes ready.
- Note
- This function consumes the futures as they are passed on to the supplied function. The callback should take one or two parameters, namely either a future to be processed or a type that std::size_t is implicitly convertible to as the first parameter and the future as the second parameter. The first parameter will correspond to the index of the current future in the collection.
- Return
- Returns a future representing the event of all input futures being ready.
- Parameters
f
: The function which will be called for each of the input futures once the future has become ready.futures
: A vector holding an arbitrary amount of future or shared_future objects for which wait_each should wait.
-
template<typename
F
, typenameIterator
>
future<Iterator>when_each
(F &&f, Iterator begin, Iterator end)¶ The function when_each is an operator allowing to join on the results of all given futures. It AND-composes all future objects given and returns a new future object representing the event of all those futures having finished executing. It also calls the supplied callback for each of the futures which becomes ready.
- Note
- This function consumes the futures as they are passed on to the supplied function. The callback should take one or two parameters, namely either a future to be processed or a type that std::size_t is implicitly convertible to as the first parameter and the future as the second parameter. The first parameter will correspond to the index of the current future in the collection.
- Return
- Returns a future representing the event of all input futures being ready.
- Parameters
f
: The function which will be called for each of the input futures once the future has become ready.begin
: The iterator pointing to the first element of a sequence of future or shared_future objects for which wait_each should wait.end
: The iterator pointing to the last element of a sequence of future or shared_future objects for which wait_each should wait.
-
template<typename
F
, typename ...Ts
>
future<void>when_each
(F &&f, Ts&&... futures)¶ The function when_each is an operator allowing to join on the results of all given futures. It AND-composes all future objects given and returns a new future object representing the event of all those futures having finished executing. It also calls the supplied callback for each of the futures which becomes ready.
- Note
- This function consumes the futures as they are passed on to the supplied function. The callback should take one or two parameters, namely either a future to be processed or a type that std::size_t is implicitly convertible to as the first parameter and the future as the second parameter. The first parameter will correspond to the index of the current future in the collection.
- Return
- Returns a future representing the event of all input futures being ready.
- Parameters
f
: The function which will be called for each of the input futures once the future has become ready.futures
: An arbitrary number of future or shared_future objects, possibly holding different types for which wait_each should wait.
-
template<typename
F
, typenameIterator
>
future<Iterator>when_each_n
(F &&f, Iterator begin, std::size_t count)¶ The function when_each is an operator allowing to join on the results of all given futures. It AND-composes all future objects given and returns a new future object representing the event of all those futures having finished executing. It also calls the supplied callback for each of the futures which becomes ready.
- Note
- This function consumes the futures as they are passed on to the supplied function. The callback should take one or two parameters, namely either a future to be processed or a type that std::size_t is implicitly convertible to as the first parameter and the future as the second parameter. The first parameter will correspond to the index of the current future in the collection.
- Return
- Returns a future holding the iterator pointing to the first element after the last one.
- Parameters
f
: The function which will be called for each of the input futures once the future has become ready.begin
: The iterator pointing to the first element of a sequence of future or shared_future objects for which wait_each_n should wait.count
: The number of elements in the sequence starting at first.
Variables
-
error_code
throws
¶ Predefined error_code object used as “throw on error” tag.
The predefined hpx::error_code object hpx::throws is supplied for use as a “throw on error” tag.
Functions that specify an argument in the form ‘error_code& ec=throws’ (with appropriate namespace qualifiers), have the following error handling semantics:
If &ec != &throws and an error occurred: ec.value() returns the implementation specific error number for the particular error that occurred and ec.category() returns the error_category for ec.value().
If &ec != &throws and an error did not occur, ec.clear().
If an error occurs and &ec == &throws, the function throws an exception of type hpx::exception or of a type derived from it. The exception’s get_errorcode() member function returns a reference to an hpx::error_code object with the behavior as specified above.
-
error_code
-
namespace
actions
¶
-
namespace
applier
¶
-
namespace
components
¶ Functions
-
template<typename
Component
>
future<naming::id_type>migrate_from_storage
(naming::id_type const &to_resurrect, naming::id_type const &target = naming::invalid_id)¶ Migrate the component with the given id from the specified target storage (resurrect the object)
The function migrate_from_storage<Component> will migrate the component referenced by to_resurrect from the storage facility specified where the object is currently stored on. It returns a future referring to the migrated component instance. The component instance is resurrected on the locality specified by target_locality.
- Return
- A future representing the global id of the migrated component instance. This should be the same as to_resurrect.
- Parameters
to_resurrect
: [in] The global id of the component to migrate.target
: [in] The optional locality to resurrect the object on. By default the object is resurrected on the locality it was located on last.
- Template Parameters
The
: only template argument specifies the component type of the component to migrate from the given storage facility.
-
template<typename
Component
>
future<naming::id_type>migrate_to_storage
(naming::id_type const &to_migrate, naming::id_type const &target_storage)¶ Migrate the component with the given id to the specified target storage
The function migrate_to_storage<Component> will migrate the component referenced by to_migrate to the storage facility specified with target_storage. It returns a future referring to the migrated component instance.
- Return
- A future representing the global id of the migrated component instance. This should be the same as migrate_to.
- Parameters
to_migrate
: [in] The global id of the component to migrate.target_storage
: [in] The id of the storage facility to migrate this object to.
- Template Parameters
The
: only template argument specifies the component type of the component to migrate to the given storage facility.
-
template<typename
Derived
, typenameStub
>
Derivedmigrate_to_storage
(client_base<Derived, Stub> const &to_migrate, hpx::components::component_storage const &target_storage)¶ Migrate the given component to the specified target storage
The function migrate_to_storage will migrate the component referenced by to_migrate to the storage facility specified with target_storage. It returns a future referring to the migrated component instance.
- Return
- A client side representation of representing of the migrated component instance. This should be the same as migrate_to.
- Parameters
to_migrate
: [in] The client side representation of the component to migrate.target_storage
: [in] The id of the storage facility to migrate this object to.
-
template<typename
Component
>
future<naming::id_type>copy
(naming::id_type const &to_copy)¶ Copy given component to the specified target locality.
The function copy<Component> will create a copy of the component referenced by to_copy on the locality specified with target_locality. It returns a future referring to the newly created component instance.
- Return
- A future representing the global id of the newly (copied) component instance.
- Note
- The new component instance is created on the locality of the component instance which is to be copied.
- Parameters
to_copy
: [in] The global id of the component to copy
- Template Parameters
The
: only template argument specifies the component type to create.
-
template<typename
Component
>
future<naming::id_type>copy
(naming::id_type const &to_copy, naming::id_type const &target_locality)¶ Copy given component to the specified target locality.
The function copy<Component> will create a copy of the component referenced by to_copy on the locality specified with target_locality. It returns a future referring to the newly created component instance.
- Return
- A future representing the global id of the newly (copied) component instance.
- Parameters
to_copy
: [in] The global id of the component to copytarget_locality
: [in ] The locality where the copy should be created.
- Template Parameters
The
: only template argument specifies the component type to create.
-
template<typename
Derived
, typenameStub
>
Derivedcopy
(client_base<Derived, Stub> const &to_copy, naming::id_type const &target_locality = naming::invalid_id)¶ Copy given component to the specified target locality.
The function copy will create a copy of the component referenced by the client side object to_copy on the locality specified with target_locality. It returns a new client side object future referring to the newly created component instance.
- Return
- A future representing the global id of the newly (copied) component instance.
- Note
- If the second argument is omitted (or is invalid_id) the new component instance is created on the locality of the component instance which is to be copied.
- Parameters
to_copy
: [in] The client side object representing the component to copytarget_locality
: [in, optional] The locality where the copy should be created (default is same locality as source).
- Template Parameters
The
: only template argument specifies the component type to create.
-
template<typename
Component
, typenameDistPolicy
>
future<naming::id_type>migrate
(naming::id_type const &to_migrate, DistPolicy const &policy)¶ Migrate the given component to the specified target locality
The function migrate<Component> will migrate the component referenced by to_migrate to the locality specified with target_locality. It returns a future referring to the migrated component instance.
- Return
- A future representing the global id of the migrated component instance. This should be the same as migrate_to.
- Parameters
to_migrate
: [in] The client side representation of the component to migrate.policy
: [in] A distribution policy which will be used to determine the locality to migrate this object to.
- Template Parameters
Component
: Specifies the component type of the component to migrate.DistPolicy
: Specifies the distribution policy to use to determine the destination locality.
-
template<typename
Derived
, typenameStub
, typenameDistPolicy
>
Derivedmigrate
(client_base<Derived, Stub> const &to_migrate, DistPolicy const &policy)¶ Migrate the given component to the specified target locality
The function migrate<Component> will migrate the component referenced by to_migrate to the locality specified with target_locality. It returns a future referring to the migrated component instance.
- Return
- A future representing the global id of the migrated component instance. This should be the same as migrate_to.
- Parameters
to_migrate
: [in] The client side representation of the component to migrate.policy
: [in] A distribution policy which will be used to determine the locality to migrate this object to.
- Template Parameters
Derived
: Specifies the component type of the component to migrate.DistPolicy
: Specifies the distribution policy to use to determine the destination locality.
-
template<typename
Component
>
future<naming::id_type>migrate
(naming::id_type const &to_migrate, naming::id_type const &target_locality)¶ Migrate the component with the given id to the specified target locality
The function migrate<Component> will migrate the component referenced by to_migrate to the locality specified with target_locality. It returns a future referring to the migrated component instance.
- Return
- A future representing the global id of the migrated component instance. This should be the same as migrate_to.
- Parameters
to_migrate
: [in] The global id of the component to migrate.target_locality
: [in] The locality where the component should be migrated to.
- Template Parameters
Component
: Specifies the component type of the component to migrate.
-
template<typename
Derived
, typenameStub
>
Derivedmigrate
(client_base<Derived, Stub> const &to_migrate, naming::id_type const &target_locality)¶ Migrate the given component to the specified target locality
The function migrate<Component> will migrate the component referenced by to_migrate to the locality specified with target_locality. It returns a future referring to the migrated component instance.
- Return
- A client side representation of representing of the migrated component instance. This should be the same as migrate_to.
- Parameters
to_migrate
: [in] The client side representation of the component to migrate.target_locality
: [in] The id of the locality to migrate this object to.
- Template Parameters
Derived
: Specifies the component type of the component to migrate.
Variables
-
char const *const
default_binpacking_counter_name
= "/runtime{locality/total}/count/component@"¶
-
binpacking_distribution_policy const
binpacked
¶ A predefined instance of the binpacking distribution_policy. It will represent the local locality and will place all items to create here.
-
colocating_distribution_policy const
colocated
¶ A predefined instance of the co-locating distribution_policy. It will represent the local locality and will place all items to create here.
-
default_distribution_policy const
default_layout
= {}¶ A predefined instance of the default distribution_policy. It will represent the local locality and will place all items to create here.
-
template<typename
-
namespace
lcos
¶ Functions
-
template<typename Action, typename ArgN, ...>hpx::future<std::vector<decltype(Action(hpx::id_type, ArgN, ...))> > hpx::lcos::broadcast(std::vector< hpx::id_type > const & ids, ArgN argN, ...)
Perform a distributed broadcast operation.
The function hpx::lcos::broadcast performs a distributed broadcast operation resulting in action invocations on a given set of global identifiers. The action can be either a plain action (in which case the global identifiers have to refer to localities) or a component action (in which case the global identifiers have to refer to instances of a component type which exposes the action.
The given action is invoked asynchronously on all given identifiers, and the arguments ArgN are passed along to those invocations.
- Return
- This function returns a future representing the result of the overall reduction operation.
- Note
- If decltype(Action(…)) is void, then the result of this function is future<void>.
- Parameters
ids
: [in] A list of global identifiers identifying the target objects for which the given action will be invoked.argN
: [in] Any number of arbitrary arguments (passed by const reference) which will be forwarded to the action invocation.
-
template<typename Action, typename ArgN, ...>void hpx::lcos::broadcast_apply(std::vector< hpx::id_type > const & ids, ArgN argN, ...)
Perform an asynchronous (fire&forget) distributed broadcast operation.
The function hpx::lcos::broadcast_apply performs an asynchronous (fire&forget) distributed broadcast operation resulting in action invocations on a given set of global identifiers. The action can be either a plain action (in which case the global identifiers have to refer to localities) or a component action (in which case the global identifiers have to refer to instances of a component type which exposes the action.
The given action is invoked asynchronously on all given identifiers, and the arguments ArgN are passed along to those invocations.
- Parameters
ids
: [in] A list of global identifiers identifying the target objects for which the given action will be invoked.argN
: [in] Any number of arbitrary arguments (passed by const reference) which will be forwarded to the action invocation.
-
template<typename Action, typename ArgN, ...>hpx::future<std::vector<decltype(Action(hpx::id_type, ArgN, ..., std::size_t))> > hpx::lcos::broadcast_with_index(std::vector< hpx::id_type > const & ids, ArgN argN, ...)
Perform a distributed broadcast operation.
The function hpx::lcos::broadcast_with_index performs a distributed broadcast operation resulting in action invocations on a given set of global identifiers. The action can be either a plain action (in which case the global identifiers have to refer to localities) or a component action (in which case the global identifiers have to refer to instances of a component type which exposes the action.
The given action is invoked asynchronously on all given identifiers, and the arguments ArgN are passed along to those invocations.
The function passes the index of the global identifier in the given list of identifiers as the last argument to the action.
- Return
- This function returns a future representing the result of the overall reduction operation.
- Note
- If decltype(Action(…)) is void, then the result of this function is future<void>.
- Parameters
ids
: [in] A list of global identifiers identifying the target objects for which the given action will be invoked.argN
: [in] Any number of arbitrary arguments (passed by const reference) which will be forwarded to the action invocation.
-
template<typename Action, typename ArgN, ...>void hpx::lcos::broadcast_apply_with_index(std::vector< hpx::id_type > const & ids, ArgN argN, ...)
Perform an asynchronous (fire&forget) distributed broadcast operation.
The function hpx::lcos::broadcast_apply_with_index performs an asynchronous (fire&forget) distributed broadcast operation resulting in action invocations on a given set of global identifiers. The action can be either a plain action (in which case the global identifiers have to refer to localities) or a component action (in which case the global identifiers have to refer to instances of a component type which exposes the action.
The given action is invoked asynchronously on all given identifiers, and the arguments ArgN are passed along to those invocations.
The function passes the index of the global identifier in the given list of identifiers as the last argument to the action.
- Parameters
ids
: [in] A list of global identifiers identifying the target objects for which the given action will be invoked.argN
: [in] Any number of arbitrary arguments (passed by const reference) which will be forwarded to the action invocation.
-
template<typename Action, typename FoldOp, typename Init, typename ArgN, ...>hpx::future<decltype(Action(hpx::id_type, ArgN, ...))> hpx::lcos::fold(std::vector< hpx::id_type > const & ids, FoldOp && fold_op, Init && init, ArgN argN, ...)
Perform a distributed fold operation.
The function hpx::lcos::fold performs a distributed folding operation over results returned from action invocations on a given set of global identifiers. The action can be either a plain action (in which case the global identifiers have to refer to localities) or a component action (in which case the global identifiers have to refer to instances of a component type which exposes the action.
- Note
- The type of the initial value must be convertible to the result type returned from the invoked action.
- Return
- This function returns a future representing the result of the overall folding operation.
- Parameters
ids
: [in] A list of global identifiers identifying the target objects for which the given action will be invoked.fold_op
: [in] A binary function expecting two results as returned from the action invocations. The function (or function object) is expected to return the result of the folding operation performed on its arguments.init
: [in] The initial value to be used for the folding operationargN
: [in] Any number of arbitrary arguments (passed by value, by const reference or by rvalue reference) which will be forwarded to the action invocation.
-
template<typename Action, typename FoldOp, typename Init, typename ArgN, ...>hpx::future<decltype(Action(hpx::id_type, ArgN, ..., std::size_t))> hpx::lcos::fold_with_index(std::vector< hpx::id_type > const & ids, FoldOp && fold_op, Init && init, ArgN argN, ...)
Perform a distributed folding operation.
The function hpx::lcos::fold_with_index performs a distributed folding operation over results returned from action invocations on a given set of global identifiers. The action can be either plain action (in which case the global identifiers have to refer to localities) or a component action (in which case the global identifiers have to refer to instances of a component type which exposes the action.
The function passes the index of the global identifier in the given list of identifiers as the last argument to the action.
- Note
- The type of the initial value must be convertible to the result type returned from the invoked action.
- Return
- This function returns a future representing the result of the overall folding operation.
- Parameters
ids
: [in] A list of global identifiers identifying the target objects for which the given action will be invoked.fold_op
: [in] A binary function expecting two results as returned from the action invocations. The function (or function object) is expected to return the result of the folding operation performed on its arguments.init
: [in] The initial value to be used for the folding operationargN
: [in] Any number of arbitrary arguments (passed by value, by const reference or by rvalue reference) which will be forwarded to the action invocation.
-
template<typename Action, typename FoldOp, typename Init, typename ArgN, ...>hpx::future<decltype(Action(hpx::id_type, ArgN, ...))> hpx::lcos::inverse_fold(std::vector< hpx::id_type > const & ids, FoldOp && fold_op, Init && init, ArgN argN, ...)
Perform a distributed inverse folding operation.
The function hpx::lcos::inverse_fold performs an inverse distributed folding operation over results returned from action invocations on a given set of global identifiers. The action can be either a plain action (in which case the global identifiers have to refer to localities) or a component action (in which case the global identifiers have to refer to instances of a component type which exposes the action.
- Note
- The type of the initial value must be convertible to the result type returned from the invoked action.
- Return
- This function returns a future representing the result of the overall folding operation.
- Parameters
ids
: [in] A list of global identifiers identifying the target objects for which the given action will be invoked.fold_op
: [in] A binary function expecting two results as returned from the action invocations. The function (or function object) is expected to return the result of the folding operation performed on its arguments.init
: [in] The initial value to be used for the folding operationargN
: [in] Any number of arbitrary arguments (passed by value, by const reference or by rvalue reference) which will be forwarded to the action invocation.
-
template<typename Action, typename FoldOp, typename Init, typename ArgN, ...>hpx::future<decltype(Action(hpx::id_type, ArgN, ..., std::size_t))> hpx::lcos::inverse_fold_with_index(std::vector< hpx::id_type > const & ids, FoldOp && fold_op, Init && init, ArgN argN, ...)
Perform a distributed inverse folding operation.
The function hpx::lcos::inverse_fold_with_index performs an inverse distributed folding operation over results returned from action invocations on a given set of global identifiers. The action can be either plain action (in which case the global identifiers have to refer to localities) or a component action (in which case the global identifiers have to refer to instances of a component type which exposes the action.
The function passes the index of the global identifier in the given list of identifiers as the last argument to the action.
- Note
- The type of the initial value must be convertible to the result type returned from the invoked action.
- Return
- This function returns a future representing the result of the overall folding operation.
- Parameters
ids
: [in] A list of global identifiers identifying the target objects for which the given action will be invoked.fold_op
: [in] A binary function expecting two results as returned from the action invocations. The function (or function object) is expected to return the result of the folding operation performed on its arguments.init
: [in] The initial value to be used for the folding operationargN
: [in] Any number of arbitrary arguments (passed by value, by const reference or by rvalue reference) which will be forwarded to the action invocation.
-
template<typename
T
>
hpx::future<std::vector<T>>gather_here
(char const *basename, hpx::future<T> result, std::size_t num_sites = std::size_t(-1), std::size_t generation = std::size_t(-1), std::size_t this_site = std::size_t(-1))¶ Gather a set of values from different call sites
This function receives a set of values from all call sites operating on the given base name.
- Note
- Each gather operation has to be accompanied with a unique usage of the HPX_REGISTER_GATHER macro to define the necessary internal facilities used by gather_here and gather_there
- Return
- This function returns a future holding a vector with all gathered values. It will become ready once the gather operation has been completed.
- Parameters
basename
: The base name identifying the gather operationresult
: A future referring to the value to transmit to the central gather point from this call site.num_sites
: The number of participating sites (default: all localities).generation
: The generational counter identifying the sequence number of the gather operation performed on the given base name. This is optional and needs to be supplied only if the gather operation on the given base name has to be performed more than once.this_site
: The sequence number of this invocation (usually the locality id). This value is optional and defaults to whatever hpx::get_locality_id() returns.
-
template<typename
T
>
hpx::future<void>gather_there
(char const *basename, hpx::future<T> result, std::size_t generation = std::size_t(-1), std::size_t root_site = 0, std::size_t this_site = std::size_t(-1))¶ Gather a given value at the given call site
This function transmits the value given by result to a central gather site (where the corresponding gather_here is executed)
- Note
- Each gather operation has to be accompanied with a unique usage of the HPX_REGISTER_GATHER macro to define the necessary internal facilities used by gather_here and gather_there
- Return
- This function returns a future which will become ready once the gather operation has been completed.
- Parameters
basename
: The base name identifying the gather operationresult
: A future referring to the value to transmit to the central gather point from this call site.generation
: The generational counter identifying the sequence number of the gather operation performed on the given base name. This is optional and needs to be supplied only if the gather operation on the given base name has to be performed more than once.root_site
: The sequence number of the central gather point (usually the locality id). This value is optional and defaults to 0.this_site
: The sequence number of this invocation (usually the locality id). This value is optional and defaults to whatever hpx::get_locality_id() returns.
-
template<typename
T
>
hpx::future<std::vector<typename std::decay<T>::type>>gather_here
(char const *basename, T &&result, std::size_t num_sites = std::size_t(-1), std::size_t generation = std::size_t(-1), std::size_t this_site = std::size_t(-1))¶ Gather a set of values from different call sites
This function receives a set of values from all call sites operating on the given base name.
- Note
- Each gather operation has to be accompanied with a unique usage of the HPX_REGISTER_GATHER macro to define the necessary internal facilities used by gather_here and gather_there
- Return
- This function returns a future holding a vector with all gathered values. It will become ready once the gather operation has been completed.
- Parameters
basename
: The base name identifying the gather operationresult
: The value to transmit to the central gather point from this call site.num_sites
: The number of participating sites (default: all localities).generation
: The generational counter identifying the sequence number of the gather operation performed on the given base name. This is optional and needs to be supplied only if the gather operation on the given base name has to be performed more than once.this_site
: The sequence number of this invocation (usually the locality id). This value is optional and defaults to whatever hpx::get_locality_id() returns.
-
template<typename
T
>
hpx::future<void>gather_there
(char const *basename, T &&result, std::size_t generation = std::size_t(-1), std::size_t root_site = 0, std::size_t this_site = std::size_t(-1))¶ Gather a given value at the given call site
This function transmits the value given by result to a central gather site (where the corresponding gather_here is executed)
- Note
- Each gather operation has to be accompanied with a unique usage of the HPX_REGISTER_GATHER macro to define the necessary internal facilities used by gather_here and gather_there
- Return
- This function returns a future which will become ready once the gather operation has been completed.
- Parameters
basename
: The base name identifying the gather operationresult
: The value to transmit to the central gather point from this call site.generation
: The generational counter identifying the sequence number of the gather operation performed on the given base name. This is optional and needs to be supplied only if the gather operation on the given base name has to be performed more than once.root_site
: The sequence number of the central gather point (usually the locality id). This value is optional and defaults to 0.this_site
: The sequence number of this invocation (usually the locality id). This value is optional and defaults to whatever hpx::get_locality_id() returns.
-
template<typename Action, typename ReduceOp, typename ArgN, ...>hpx::future<decltype(Action(hpx::id_type, ArgN, ...))> hpx::lcos::reduce(std::vector< hpx::id_type > const & ids, ReduceOp && reduce_op, ArgN argN, ...)
Perform a distributed reduction operation.
The function hpx::lcos::reduce performs a distributed reduction operation over results returned from action invocations on a given set of global identifiers. The action can be either a plain action (in which case the global identifiers have to refer to localities) or a component action (in which case the global identifiers have to refer to instances of a component type which exposes the action.
- Return
- This function returns a future representing the result of the overall reduction operation.
- Parameters
ids
: [in] A list of global identifiers identifying the target objects for which the given action will be invoked.reduce_op
: [in] A binary function expecting two results as returned from the action invocations. The function (or function object) is expected to return the result of the reduction operation performed on its arguments.argN
: [in] Any number of arbitrary arguments (passed by by const reference) which will be forwarded to the action invocation.
-
template<typename Action, typename ReduceOp, typename ArgN, ...>hpx::future<decltype(Action(hpx::id_type, ArgN, ..., std::size_t))> hpx::lcos::reduce_with_index(std::vector< hpx::id_type > const & ids, ReduceOp && reduce_op, ArgN argN, ...)
Perform a distributed reduction operation.
The function hpx::lcos::reduce_with_index performs a distributed reduction operation over results returned from action invocations on a given set of global identifiers. The action can be either plain action (in which case the global identifiers have to refer to localities) or a component action (in which case the global identifiers have to refer to instances of a component type which exposes the action.
The function passes the index of the global identifier in the given list of identifiers as the last argument to the action.
- Return
- This function returns a future representing the result of the overall reduction operation.
- Parameters
ids
: [in] A list of global identifiers identifying the target objects for which the given action will be invoked.reduce_op
: [in] A binary function expecting two results as returned from the action invocations. The function (or function object) is expected to return the result of the reduction operation performed on its arguments.argN
: [in] Any number of arbitrary arguments (passed by by const reference) which will be forwarded to the action invocation.
-
-
namespace
naming
¶ Functions
-
id_type
unmanaged
(id_type const &id)¶ The helper function hpx::unmanaged can be used to generate a global identifier which does not participate in the automatic garbage collection.
- Return
- This function returns a new global id referencing the same object as the parameter id. The only difference is that the returned global identifier does not participate in the automatic garbage collection.
- Note
- This function allows to apply certain optimizations to the process of memory management in HPX. It however requires the user to take full responsibility for keeping the referenced objects alive long enough.
- Parameters
id
: [in] The id to generated the unmanaged global id from This parameter can be itself a managed or a unmanaged global id.
-
id_type
-
namespace
parallel
¶
-
namespace
execution
¶ Typedefs
-
using
service_executor
= threads::executors::service_executor¶ A service_executor exposes one of the predefined HPX thread pools through an executor interface.
- Note
- All tasks executed by one of these executors will run on one of the OS-threads dedicated for the given thread pool. The tasks will not run as HPX-threads.
-
using
io_pool_executor
= threads::executors::io_pool_executor¶ A io_pool_executor exposes the predefined HPX IO thread pool through an executor interface.
- Note
- All tasks executed by one of these executors will run on one of the OS-threads dedicated for the IO thread pool. The tasks will not run as HPX-threads.
-
using
parcel_pool_executor
= threads::executors::parcel_pool_executor¶ A io_pool_executor exposes the predefined HPX parcel thread pool through an executor interface.
- Note
- All tasks executed by one of these executors will run on one of the OS-threads dedicated for the parcel thread pool. The tasks will not run as HPX-threads.
-
using
timer_pool_executor
= threads::executors::timer_pool_executor¶ A io_pool_executor exposes the predefined HPX timer thread pool through an executor interface.
- Note
- All tasks executed by one of these executors will run on one of the OS-threads dedicated for the timer thread pool. The tasks will not run as HPX-threads.
-
using
main_pool_executor
= threads::executors::main_pool_executor¶ A io_pool_executor exposes the predefined HPX main thread pool through an executor interface.
- Note
- All tasks executed by one of these executors will run on one of the OS-threads dedicated for the main thread pool. The tasks will not run as HPX-threads.
-
using
local_priority_queue_executor
= threads::executors::local_priority_queue_executor¶ Creates a new local_priority_queue_executor
- Parameters
max_punits
: [in] The maximum number of processing units to associate with the newly created executor.min_punits
: [in] The minimum number of processing units to associate with the newly created executor (default: 1).
Variables
-
task_policy_tag HPX_CONSTEXPR_OR_CONST hpx::parallel::execution::task
Default sequential execution policy object.
-
HPX_STATIC_CONSTEXPR sequenced_policy hpx::parallel::execution::seq
Default sequential execution policy object.
-
HPX_STATIC_CONSTEXPR parallel_policy hpx::parallel::execution::par
Default parallel execution policy object.
-
HPX_STATIC_CONSTEXPR parallel_unsequenced_policy hpx::parallel::execution::par_unseq
Default vector execution policy object.
-
using
-
namespace [anonymous]¶
-
namespace [anonymous]¶
-
namespace
v1
¶ Functions
-
template<typename
ExPolicy
, typenameFwdIter1
, typenameFwdIter2
>
std::enable_if<execution::is_execution_policy<ExPolicy>::value, typename util::detail::algorithm_result<ExPolicy, FwdIter2>::type>::typeadjacent_difference
(ExPolicy &&policy, FwdIter1 first, FwdIter1 last, FwdIter2 dest)¶ Assigns each value in the range given by result its corresponding element in the range [first, last] and the one preceding it except *result, which is assigned *first
The difference operations in the parallel
adjacent_difference invoked with an execution policy object of type sequenced_policy execute in sequential order in the calling thread.- Note
- Complexity: Exactly (last - first) - 1 application of the binary operator and (last - first) assignments.
- Template Parameters
ExPolicy
: The type of the execution policy to use (deduced). It describes the manner in which the execution of the algorithm may be parallelized and the manner in which it executes the assignments.FwdIter1
: The type of the source iterators used for the input range (deduced). This iterator type must meet the requirements of an forward iterator.FwdIter2
: The type of the source iterators used for the output range (deduced). This iterator type must meet the requirements of an forward iterator.
- Parameters
policy
: The execution policy to use for the scheduling of the iterations.first
: Refers to the beginning of the sequence of elements of the range the algorithm will be applied to.last
: Refers to the end of the sequence of elements of the range the algorithm will be applied to.dest
: Refers to the beginning of the sequence of elements the results will be assigned to.
The difference operations in the parallel adjacent_difference invoked with an execution policy object of type parallel_policy or parallel_task_policy are permitted to execute in an unordered fashion in unspecified threads, and indeterminately sequenced within each thread.
This overload of
adjacent_find is available if the user decides to provide their algorithm their own binary predicate op.- Return
- The adjacent_difference algorithm returns a hpx::future<FwdIter2> if the execution policy is of type sequenced_task_policy or parallel_task_policy and returns FwdIter2 otherwise. The adjacent_find algorithm returns an iterator to the last element in the output range.
-
template<typename
ExPolicy
, typenameFwdIter1
, typenameFwdIter2
, typenameOp
>
std::enable_if<execution::is_execution_policy<ExPolicy>::value, typename util::detail::algorithm_result<ExPolicy, FwdIter2>::type>::typeadjacent_difference
(ExPolicy &&policy, FwdIter1 first, FwdIter1 last, FwdIter2 dest, Op &&op)¶ Assigns each value in the range given by result its corresponding element in the range [first, last] and the one preceding it except *result, which is assigned *first
The difference operations in the parallel
adjacent_difference invoked with an execution policy object of type sequenced_policy execute in sequential order in the calling thread.- Note
- Complexity: Exactly (last - first) - 1 application of the binary operator and (last - first) assignments.
- Template Parameters
ExPolicy
: The type of the execution policy to use (deduced). It describes the manner in which the execution of the algorithm may be parallelized and the manner in which it executes the assignments.FwdIter1
: The type of the source iterators used for the input range (deduced). This iterator type must meet the requirements of an forward iterator.FwdIter2
: The type of the source iterators used for the output range (deduced). This iterator type must meet the requirements of an forward iterator.Op
: The type of the function/function object to use (deduced). Unlike its sequential form, the parallel overload of adjacent_difference requires Op to meet the requirements of CopyConstructible.
- Parameters
policy
: The execution policy to use for the scheduling of the iterations.first
: Refers to the beginning of the sequence of elements of the range the algorithm will be applied to.last
: Refers to the end of the sequence of elements of the range the algorithm will be applied to.dest
: Refers to the beginning of the sequence of elements the results will be assigned to.op
: The binary operator which returns the difference of elements. The signature should be equivalent to the following:bool op(const Type1 &a, const Type1 &b);
The signature does not need to have const &, but the function must not modify the objects passed to it. The types
Type1 must be such that objects of type FwdIter1 can be dereferenced and then implicitly converted to the dereferenced type of dest.
The difference operations in the parallel adjacent_difference invoked with an execution policy object of type parallel_policy or parallel_task_policy are permitted to execute in an unordered fashion in unspecified threads, and indeterminately sequenced within each thread.
- Return
- The adjacent_difference algorithm returns a hpx::future<FwdIter2> if the execution policy is of type sequenced_task_policy or parallel_task_policy and returns FwdIter2 otherwise. The adjacent_find algorithm returns an iterator to the last element in the output range.
-
template<typename
ExPolicy
, typenameFwdIter
, typenamePred
= detail::equal_to>
std::enable_if<execution::is_execution_policy<ExPolicy>::value, typename util::detail::algorithm_result<ExPolicy, FwdIter>::type>::typeadjacent_find
(ExPolicy &&policy, FwdIter first, FwdIter last, Pred &&op = Pred())¶ Searches the range [first, last) for two consecutive identical elements. This version uses the given binary predicate op
The comparison operations in the parallel
adjacent_find invoked with an execution policy object of type sequenced_policy execute in sequential order in the calling thread.- Note
- Complexity: Exactly the smaller of (result - first) + 1 and (last - first) - 1 application of the predicate where result is the value returned
- Template Parameters
ExPolicy
: The type of the execution policy to use (deduced). It describes the manner in which the execution of the algorithm may be parallelized and the manner in which it executes the assignments.FwdIter
: The type of the source iterators used for the range (deduced). This iterator type must meet the requirements of an forward iterator.Pred
: The type of an optional function/function object to use. Unlike its sequential form, the parallel overload of adjacent_find requires Pred to meet the requirements of CopyConstructible. This defaults to std::equal_to<>
- Parameters
policy
: The execution policy to use for the scheduling of the iterations.first
: Refers to the beginning of the sequence of elements of the range the algorithm will be applied to.last
: Refers to the end of the sequence of elements of the range the algorithm will be applied to.op
: The binary predicate which returns true if the elements should be treated as equal. The signature should be equivalent to the following:bool pred(const Type1 &a, const Type1 &b);
The signature does not need to have const &, but the function must not modify the objects passed to it. The types
Type1 must be such that objects of type FwdIter can be dereferenced and then implicitly converted to Type1 .
The comparison operations in the parallel adjacent_find invoked with an execution policy object of type parallel_policy or parallel_task_policy are permitted to execute in an unordered fashion in unspecified threads, and indeterminately sequenced within each thread.
This overload of
adjacent_find is available if the user decides to provide their algorithm their own binary predicate op.- Return
- The adjacent_find algorithm returns a hpx::future<InIter> if the execution policy is of type sequenced_task_policy or parallel_task_policy and returns InIter otherwise. The adjacent_find algorithm returns an iterator to the first of the identical elements. If no such elements are found, last is returned.
-
template<typename
ExPolicy
, typenameFwdIter
, typenameF
, typenameProj
= util::projection_identity>
util::detail::algorithm_result<ExPolicy, bool>::typenone_of
(ExPolicy &&policy, FwdIter first, FwdIter last, F &&f, Proj &&proj = Proj())¶ Checks if unary predicate f returns true for no elements in the range [first, last).
The application of function objects in parallel algorithm invoked with an execution policy object of type
sequenced_policy execute in sequential order in the calling thread.- Note
- Complexity: At most last - first applications of the predicate f
- Template Parameters
ExPolicy
: The type of the execution policy to use (deduced). It describes the manner in which the execution of the algorithm may be parallelized and the manner in which it applies user-provided function objects.FwdIter
: The type of the source iterators used (deduced). This iterator type must meet the requirements of an forward iterator.F
: The type of the function/function object to use (deduced). Unlike its sequential form, the parallel overload of none_of requires F to meet the requirements of CopyConstructible.Proj
: The type of an optional projection function. This defaults to util::projection_identity
- Parameters
policy
: The execution policy to use for the scheduling of the iterations.first
: Refers to the beginning of the sequence of elements the algorithm will be applied to.last
: Refers to the end of the sequence of elements the algorithm will be applied to.f
: Specifies the function (or function object) which will be invoked for each of the elements in the sequence specified by [first, last). The signature of this predicate should be equivalent to:bool pred(const Type &a);
The signature does not need to have const&, but the function must not modify the objects passed to it. The type
Type must be such that an object of type FwdIter can be dereferenced and then implicitly converted to Type.proj
: Specifies the function (or function object) which will be invoked for each of the elements as a projection operation before the actual predicate is invoked.
The application of function objects in parallel algorithm invoked with an execution policy object of type parallel_policy or parallel_task_policy are permitted to execute in an unordered fashion in unspecified threads, and indeterminately sequenced within each thread.
- Return
- The none_of algorithm returns a hpx::future<bool> if the execution policy is of type sequenced_task_policy or parallel_task_policy and returns bool otherwise. The none_of algorithm returns true if the unary predicate f returns true for no elements in the range, false otherwise. It returns true if the range is empty.
-
template<typename
ExPolicy
, typenameFwdIter
, typenameF
, typenameProj
= util::projection_identity>
util::detail::algorithm_result<ExPolicy, bool>::typeany_of
(ExPolicy &&policy, FwdIter first, FwdIter last, F &&f, Proj &&proj = Proj())¶ Checks if unary predicate f returns true for at least one element in the range [first, last).
The application of function objects in parallel algorithm invoked with an execution policy object of type
sequenced_policy execute in sequential order in the calling thread.- Note
- Complexity: At most last - first applications of the predicate f
- Template Parameters
ExPolicy
: The type of the execution policy to use (deduced). It describes the manner in which the execution of the algorithm may be parallelized and the manner in which it applies user-provided function objects.FwdIter
: The type of the source iterators used (deduced). This iterator type must meet the requirements of an forward iterator.F
: The type of the function/function object to use (deduced). Unlike its sequential form, the parallel overload of any_of requires F to meet the requirements of CopyConstructible.Proj
: The type of an optional projection function. This defaults to util::projection_identity
- Parameters
policy
: The execution policy to use for the scheduling of the iterations.first
: Refers to the beginning of the sequence of elements the algorithm will be applied to.last
: Refers to the end of the sequence of elements the algorithm will be applied to.f
: Specifies the function (or function object) which will be invoked for each of the elements in the sequence specified by [first, last). The signature of this predicate should be equivalent to:bool pred(const Type &a);
The signature does not need to have const&, but the function must not modify the objects passed to it. The type
Type must be such that an object of type FwdIter can be dereferenced and then implicitly converted to Type.proj
: Specifies the function (or function object) which will be invoked for each of the elements as a projection operation before the actual predicate is invoked.
The application of function objects in parallel algorithm invoked with an execution policy object of type parallel_policy or parallel_task_policy are permitted to execute in an unordered fashion in unspecified threads, and indeterminately sequenced within each thread.
- Return
- The any_of algorithm returns a hpx::future<bool> if the execution policy is of type sequenced_task_policy or parallel_task_policy and returns bool otherwise. The any_of algorithm returns true if the unary predicate f returns true for at least one element in the range, false otherwise. It returns false if the range is empty.
-
template<typename
ExPolicy
, typenameFwdIter
, typenameF
, typenameProj
= util::projection_identity>
util::detail::algorithm_result<ExPolicy, bool>::typeall_of
(ExPolicy &&policy, FwdIter first, FwdIter last, F &&f, Proj &&proj = Proj())¶ Checks if unary predicate f returns true for all elements in the range [first, last).
The application of function objects in parallel algorithm invoked with an execution policy object of type
sequenced_policy execute in sequential order in the calling thread.- Note
- Complexity: At most last - first applications of the predicate f
- Template Parameters
ExPolicy
: The type of the execution policy to use (deduced). It describes the manner in which the execution of the algorithm may be parallelized and the manner in which it applies user-provided function objects.FwdIter
: The type of the source iterators used (deduced). This iterator type must meet the requirements of an forward iterator.F
: The type of the function/function object to use (deduced). Unlike its sequential form, the parallel overload of all_of requires F to meet the requirements of CopyConstructible.Proj
: The type of an optional projection function. This defaults to util::projection_identity
- Parameters
policy
: The execution policy to use for the scheduling of the iterations.first
: Refers to the beginning of the sequence of elements the algorithm will be applied to.last
: Refers to the end of the sequence of elements the algorithm will be applied to.f
: Specifies the function (or function object) which will be invoked for each of the elements in the sequence specified by [first, last). The signature of this predicate should be equivalent to:bool pred(const Type &a);
The signature does not need to have const&, but the function must not modify the objects passed to it. The type
Type must be such that an object of type FwdIter can be dereferenced and then implicitly converted to Type.proj
: Specifies the function (or function object) which will be invoked for each of the elements as a projection operation before the actual predicate is invoked.
The application of function objects in parallel algorithm invoked with an execution policy object of type parallel_policy or parallel_task_policy are permitted to execute in an unordered fashion in unspecified threads, and indeterminately sequenced within each thread.
- Return
- The all_of algorithm returns a hpx::future<bool> if the execution policy is of type sequenced_task_policy or parallel_task_policy and returns bool otherwise. The all_of algorithm returns true if the unary predicate f returns true for all elements in the range, false otherwise. It returns true if the range is empty.
-
template<typename
ExPolicy
, typenameFwdIter1
, typenameFwdIter2
>
util::detail::algorithm_result<ExPolicy, hpx::util::tagged_pair<tag::in(FwdIter1), tag::out(FwdIter2)>>::typecopy
(ExPolicy &&policy, FwdIter1 first, FwdIter1 last, FwdIter2 dest)¶ Copies the elements in the range, defined by [first, last), to another range beginning at dest.
The assignments in the parallel
copy algorithm invoked with an execution policy object of type sequenced_policy execute in sequential order in the calling thread.- Note
- Complexity: Performs exactly last - first assignments.
- Template Parameters
ExPolicy
: The type of the execution policy to use (deduced). It describes the manner in which the execution of the algorithm may be parallelized and the manner in which it executes the assignments.FwdIter1
: The type of the source iterators used (deduced). This iterator type must meet the requirements of an forward iterator.FwdIter2
: The type of the iterator representing the destination range (deduced). This iterator type must meet the requirements of an forward iterator.
- Parameters
policy
: The execution policy to use for the scheduling of the iterations.first
: Refers to the beginning of the sequence of elements the algorithm will be applied to.last
: Refers to the end of the sequence of elements the algorithm will be applied to.dest
: Refers to the beginning of the destination range.
The assignments in the parallel copy algorithm invoked with an execution policy object of type parallel_policy or parallel_task_policy are permitted to execute in an unordered fashion in unspecified threads, and indeterminately sequenced within each thread.
- Return
- The copy algorithm returns a hpx::future<tagged_pair<tag::in(FwdIter1), tag::out(FwdIter2)> > if the execution policy is of type sequenced_task_policy or parallel_task_policy and returns tagged_pair<tag::in(FwdIter1), tag::out(FwdIter2)> otherwise. The copy algorithm returns the pair of the input iterator last and the output iterator to the element in the destination range, one past the last element copied.
-
template<typename
ExPolicy
, typenameFwdIter1
, typenameSize
, typenameFwdIter2
>
util::detail::algorithm_result<ExPolicy, hpx::util::tagged_pair<tag::in(FwdIter1), tag::out(FwdIter2)>>::typecopy_n
(ExPolicy &&policy, FwdIter1 first, Size count, FwdIter2 dest)¶ Copies the elements in the range [first, first + count), starting from first and proceeding to first + count - 1., to another range beginning at dest.
The assignments in the parallel
copy_n algorithm invoked with an execution policy object of type sequenced_policy execute in sequential order in the calling thread.- Note
- Complexity: Performs exactly count assignments, if count > 0, no assignments otherwise.
- Template Parameters
ExPolicy
: The type of the execution policy to use (deduced). It describes the manner in which the execution of the algorithm may be parallelized and the manner in which it executes the assignments.FwdIter1
: The type of the source iterators used (deduced). This iterator type must meet the requirements of an forward iterator.Size
: The type of the argument specifying the number of elements to apply f to.FwdIter2
: The type of the iterator representing the destination range (deduced). This iterator type must meet the requirements of an forward iterator.
- Parameters
policy
: The execution policy to use for the scheduling of the iterations.first
: Refers to the beginning of the sequence of elements the algorithm will be applied to.count
: Refers to the number of elements starting at first the algorithm will be applied to.dest
: Refers to the beginning of the destination range.
The assignments in the parallel copy_n algorithm invoked with an execution policy object of type parallel_policy or parallel_task_policy are permitted to execute in an unordered fashion in unspecified threads, and indeterminately sequenced within each thread.
- Return
- The copy_n algorithm returns a hpx::future<tagged_pair<tag::in(FwdIter1), tag::out(FwdIter2)> > if the execution policy is of type sequenced_task_policy or parallel_task_policy and returns tagged_pair<tag::in(FwdIter1), tag::out(FwdIter2)> otherwise. The copy algorithm returns the pair of the input iterator forwarded to the first element after the last in the input sequence and the output iterator to the element in the destination range, one past the last element copied.
-
template<typename
ExPolicy
, typenameFwdIter1
, typenameFwdIter2
, typenameF
, typenameProj
= util::projection_identity>
util::detail::algorithm_result<ExPolicy, hpx::util::tagged_pair<tag::in(FwdIter1), tag::out(FwdIter2)>>::typecopy_if
(ExPolicy &&policy, FwdIter1 first, FwdIter1 last, FwdIter2 dest, F &&f, Proj &&proj = Proj())¶ Copies the elements in the range, defined by [first, last), to another range beginning at dest. Copies only the elements for which the predicate f returns true. The order of the elements that are not removed is preserved.
The assignments in the parallel
copy_if algorithm invoked with an execution policy object of type sequenced_policy execute in sequential order in the calling thread.- Note
- Complexity: Performs not more than last - first assignments, exactly last - first applications of the predicate f.
- Template Parameters
ExPolicy
: The type of the execution policy to use (deduced). It describes the manner in which the execution of the algorithm may be parallelized and the manner in which it executes the assignments.FwdIter1
: The type of the source iterators used (deduced). This iterator type must meet the requirements of an forward iterator.FwdIter2
: The type of the iterator representing the destination range (deduced). This iterator type must meet the requirements of an forward iterator.F
: The type of the function/function object to use (deduced). Unlike its sequential form, the parallel overload of copy_if requires F to meet the requirements of CopyConstructible.Proj
: The type of an optional projection function. This defaults to util::projection_identity
- Parameters
policy
: The execution policy to use for the scheduling of the iterations.first
: Refers to the beginning of the sequence of elements the algorithm will be applied to.last
: Refers to the end of the sequence of elements the algorithm will be applied to.dest
: Refers to the beginning of the destination range.f
: Specifies the function (or function object) which will be invoked for each of the elements in the sequence specified by [first, last).This is an unary predicate which returns true for the required elements. The signature of this predicate should be equivalent to:bool pred(const Type &a);
The signature does not need to have const&, but the function must not modify the objects passed to it. The type
Type must be such that an object of type FwdIter1 can be dereferenced and then implicitly converted to Type.proj
: Specifies the function (or function object) which will be invoked for each of the elements as a projection operation before the actual predicate is invoked.
The assignments in the parallel copy_if algorithm invoked with an execution policy object of type parallel_policy or parallel_task_policy are permitted to execute in an unordered fashion in unspecified threads, and indeterminately sequenced within each thread.
- Return
- The copy_if algorithm returns a hpx::future<tagged_pair<tag::in(FwdIter1), tag::out(FwdIter2)> > if the execution policy is of type sequenced_task_policy or parallel_task_policy and returns tagged_pair<tag::in(FwdIter1), tag::out(FwdIter2)> otherwise. The copy algorithm returns the pair of the input iterator forwarded to the first element after the last in the input sequence and the output iterator to the element in the destination range, one past the last element copied.
-
template<typename
ExPolicy
, typenameFwdIterB
, typenameFwdIterE
, typenameT
, typenameProj
= util::projection_identity>
util::detail::algorithm_result<ExPolicy, typename std::iterator_traits<FwdIterB>::difference_type>::typecount
(ExPolicy &&policy, FwdIterB first, FwdIterE last, T const &value, Proj &&proj = Proj())¶ Returns the number of elements in the range [first, last) satisfying a specific criteria. This version counts the elements that are equal to the given value.
The comparisons in the parallel
count algorithm invoked with an execution policy object of type sequenced_policy execute in sequential order in the calling thread.- Note
- Complexity: Performs exactly last - first comparisons.
- Template Parameters
ExPolicy
: The type of the execution policy to use (deduced). It describes the manner in which the execution of the algorithm may be parallelized and the manner in which it executes the comparisons.FwdIterB
: The type of the source begin iterator used (deduced). This iterator type must meet the requirements of an forward iterator.FwdIterE
: The type of the source end iterator used (deduced). This iterator type must meet the requirements of an forward iterator.T
: The type of the value to search for (deduced).Proj
: The type of an optional projection function. This defaults to util::projection_identity
- Parameters
policy
: The execution policy to use for the scheduling of the iterations.first
: Refers to the beginning of the sequence of elements the algorithm will be applied to.last
: Refers to the end of the sequence of elements the algorithm will be applied to.value
: The value to search for.proj
: Specifies the function (or function object) which will be invoked for each of the elements as a projection operation before the actual predicate is invoked.
- Note
- The comparisons in the parallel count algorithm invoked with an execution policy object of type parallel_policy or parallel_task_policy are permitted to execute in an unordered fashion in unspecified threads, and indeterminately sequenced within each thread.
- Return
- The count algorithm returns a hpx::future<difference_type> if the execution policy is of type sequenced_task_policy or parallel_task_policy and returns difference_type otherwise (where difference_type is defined by std::iterator_traits<FwdIterB>::difference_type. The count algorithm returns the number of elements satisfying the given criteria.
-
template<typename
ExPolicy
, typenameFwdIterB
, typenameFwdIterE
, typenameF
, typenameProj
= util::projection_identity>
util::detail::algorithm_result<ExPolicy, typename std::iterator_traits<FwdIterB>::difference_type>::typecount_if
(ExPolicy &&policy, FwdIterB first, FwdIterE last, F &&f, Proj &&proj = Proj())¶ Returns the number of elements in the range [first, last) satisfying a specific criteria. This version counts elements for which predicate f returns true.
- Note
- Complexity: Performs exactly last - first applications of the predicate.
- Note
- The assignments in the parallel count_if algorithm invoked with an execution policy object of type sequenced_policy execute in sequential order in the calling thread.
- Note
- The assignments in the parallel count_if algorithm invoked with an execution policy object of type parallel_policy or parallel_task_policy are permitted to execute in an unordered fashion in unspecified threads, and indeterminately sequenced within each thread.
- Return
- The count_if algorithm returns hpx::future<difference_type> if the execution policy is of type sequenced_task_policy or parallel_task_policy and returns difference_type otherwise (where difference_type is defined by std::iterator_traits<FwdIterB>::difference_type. The count algorithm returns the number of elements satisfying the given criteria.
- Template Parameters
ExPolicy
: The type of the execution policy to use (deduced). It describes the manner in which the execution of the algorithm may be parallelized and the manner in which it executes the comparisons.FwdIterB
: The type of the source begin iterator used (deduced). This iterator type must meet the requirements of an forward iterator.FwdIterE
: The type of the source end iterator used (deduced). This iterator type must meet the requirements of an forward iterator.F
: The type of the function/function object to use (deduced). Unlike its sequential form, the parallel overload of count_if requires F to meet the requirements of CopyConstructible.Proj
: The type of an optional projection function. This defaults to util::projection_identity
- Parameters
policy
: The execution policy to use for the scheduling of the iterations.first
: Refers to the beginning of the sequence of elements the algorithm will be applied to.last
: Refers to the end of the sequence of elements the algorithm will be applied to.f
: Specifies the function (or function object) which will be invoked for each of the elements in the sequence specified by [first, last).This is an unary predicate which returns true for the required elements. The signature of this predicate should be equivalent to:bool pred(const Type &a);
The signature does not need to have const&, but the function must not modify the objects passed to it. The type
Type must be such that an object of type FwdIterB can be dereferenced and then implicitly converted to Type.proj
: Specifies the function (or function object) which will be invoked for each of the elements as a projection operation before the actual predicate is invoked.
-
template<typename
ExPolicy
, typenameFwdIter
>
util::detail::algorithm_result<ExPolicy>::typedestroy
(ExPolicy &&policy, FwdIter first, FwdIter last)¶ Destroys objects of type typename iterator_traits<ForwardIt>::value_type in the range [first, last).
The operations in the parallel
destroy algorithm invoked with an execution policy object of type sequenced_policy execute in sequential order in the calling thread.- Note
- Complexity: Performs exactly last - first operations.
- Template Parameters
ExPolicy
: The type of the execution policy to use (deduced). It describes the manner in which the execution of the algorithm may be parallelized and the manner in which it executes the assignments.FwdIter
: The type of the source iterators used (deduced). This iterator type must meet the requirements of an forward iterator.
- Parameters
policy
: The execution policy to use for the scheduling of the iterations.first
: Refers to the beginning of the sequence of elements the algorithm will be applied to.last
: Refers to the end of the sequence of elements the algorithm will be applied to.
The operations in the parallel destroy algorithm invoked with an execution policy object of type parallel_policy or parallel_task_policy are permitted to execute in an unordered fashion in unspecified threads, and indeterminately sequenced within each thread.
- Return
- The destroy algorithm returns a hpx::future<void>, if the execution policy is of type sequenced_task_policy or parallel_task_policy and returns void otherwise.
-
template<typename
ExPolicy
, typenameFwdIter
, typenameSize
>
util::detail::algorithm_result<ExPolicy, FwdIter>::typedestroy_n
(ExPolicy &&policy, FwdIter first, Size count)¶ Destroys objects of type typename iterator_traits<ForwardIt>::value_type in the range [first, first + count).
The operations in the parallel
destroy_n algorithm invoked with an execution policy object of type sequenced_policy execute in sequential order in the calling thread.- Note
- Complexity: Performs exactly count operations, if count > 0, no assignments otherwise.
- Template Parameters
ExPolicy
: The type of the execution policy to use (deduced). It describes the manner in which the execution of the algorithm may be parallelized and the manner in which it executes the assignments.FwdIter
: The type of the source iterators used (deduced). This iterator type must meet the requirements of an forward iterator.Size
: The type of the argument specifying the number of elements to apply this algorithm to.
- Parameters
policy
: The execution policy to use for the scheduling of the iterations.first
: Refers to the beginning of the sequence of elements the algorithm will be applied to.count
: Refers to the number of elements starting at first the algorithm will be applied to.
The operations in the parallel destroy_n algorithm invoked with an execution policy object of type parallel_policy or parallel_task_policy are permitted to execute in an unordered fashion in unspecified threads, and indeterminately sequenced within each thread.
- Return
- The destroy_n algorithm returns a hpx::future<FwdIter> if the execution policy is of type sequenced_task_policy or parallel_task_policy and returns FwdIter otherwise. The destroy_n algorithm returns the iterator to the element in the source range, one past the last element constructed.
-
template<typename
ExPolicy
, typenameFwdIter1
, typenameFwdIter2
, typenamePred
= detail::equal_to>
std::enable_if<execution::is_execution_policy<ExPolicy>::value, typename util::detail::algorithm_result<ExPolicy, bool>::type>::typeequal
(ExPolicy &&policy, FwdIter1 first1, FwdIter1 last1, FwdIter2 first2, FwdIter2 last2, Pred &&op = Pred())¶ Returns true if the range [first1, last1) is equal to the range [first2, last2), and false otherwise.
The comparison operations in the parallel
equal algorithm invoked with an execution policy object of type sequenced_policy execute in sequential order in the calling thread.- Note
- Complexity: At most min(last1 - first1, last2 - first2) applications of the predicate f.
- Template Parameters
ExPolicy
: The type of the execution policy to use (deduced). It describes the manner in which the execution of the algorithm may be parallelized and the manner in which it executes the assignments.FwdIter1
: The type of the source iterators used for the first range (deduced). This iterator type must meet the requirements of an forward iterator.FwdIter2
: The type of the source iterators used for the second range (deduced). This iterator type must meet the requirements of an forward iterator.Pred
: The type of an optional function/function object to use. Unlike its sequential form, the parallel overload of equal requires Pred to meet the requirements of CopyConstructible. This defaults to std::equal_to<>
- Parameters
policy
: The execution policy to use for the scheduling of the iterations.first1
: Refers to the beginning of the sequence of elements of the first range the algorithm will be applied to.last1
: Refers to the end of the sequence of elements of the first range the algorithm will be applied to.first2
: Refers to the beginning of the sequence of elements of the second range the algorithm will be applied to.last2
: Refers to the end of the sequence of elements of the second range the algorithm will be applied to.op
: The binary predicate which returns true if the elements should be treated as equal. The signature of the predicate function should be equivalent to the following:bool pred(const Type1 &a, const Type2 &b);
The signature does not need to have const &, but the function must not modify the objects passed to it. The types
Type1 and Type2 must be such that objects of types FwdIter1 and FwdIter2 can be dereferenced and then implicitly converted to Type1 and Type2 respectively
The comparison operations in the parallel equal algorithm invoked with an execution policy object of type parallel_policy or parallel_task_policy are permitted to execute in an unordered fashion in unspecified threads, and indeterminately sequenced within each thread.
- Note
- The two ranges are considered equal if, for every iterator i in the range [first1,last1), *i equals *(first2 + (i - first1)). This overload of equal uses operator== to determine if two elements are equal.
- Return
- The equal algorithm returns a hpx::future<bool> if the execution policy is of type sequenced_task_policy or parallel_task_policy and returns bool otherwise. The equal algorithm returns true if the elements in the two ranges are equal, otherwise it returns false. If the length of the range [first1, last1) does not equal the length of the range [first2, last2), it returns false.
-
template<typename
ExPolicy
, typenameFwdIter1
, typenameFwdIter2
, typenamePred
= detail::equal_to>
std::enable_if<execution::is_execution_policy<ExPolicy>::value, typename util::detail::algorithm_result<ExPolicy, bool>::type>::typeequal
(ExPolicy &&policy, FwdIter1 first1, FwdIter1 last1, FwdIter2 first2, Pred &&op = Pred())¶ Returns true if the range [first1, last1) is equal to the range starting at first2, and false otherwise.
The comparison operations in the parallel
equal algorithm invoked with an execution policy object of type sequenced_policy execute in sequential order in the calling thread.- Note
- Complexity: At most last1 - first1 applications of the predicate f.
- Template Parameters
ExPolicy
: The type of the execution policy to use (deduced). It describes the manner in which the execution of the algorithm may be parallelized and the manner in which it executes the assignments.FwdIter1
: The type of the source iterators used for the first range (deduced). This iterator type must meet the requirements of an forward iterator.FwdIter2
: The type of the source iterators used for the second range (deduced). This iterator type must meet the requirements of an forward iterator.Pred
: The type of an optional function/function object to use. Unlike its sequential form, the parallel overload of equal requires Pred to meet the requirements of CopyConstructible. This defaults to std::equal_to<>
- Parameters
policy
: The execution policy to use for the scheduling of the iterations.first1
: Refers to the beginning of the sequence of elements of the first range the algorithm will be applied to.last1
: Refers to the end of the sequence of elements of the first range the algorithm will be applied to.first2
: Refers to the beginning of the sequence of elements of the second range the algorithm will be applied to.op
: The binary predicate which returns true if the elements should be treated as equal. The signature of the predicate function should be equivalent to the following:bool pred(const Type1 &a, const Type2 &b);
The signature does not need to have const &, but the function must not modify the objects passed to it. The types
Type1 and Type2 must be such that objects of types FwdIter1 and FwdIter2 can be dereferenced and then implicitly converted to Type1 and Type2 respectively
The comparison operations in the parallel equal algorithm invoked with an execution policy object of type parallel_policy or parallel_task_policy are permitted to execute in an unordered fashion in unspecified threads, and indeterminately sequenced within each thread.
- Note
- The two ranges are considered equal if, for every iterator i in the range [first1,last1), *i equals *(first2 + (i - first1)). This overload of equal uses operator== to determine if two elements are equal.
- Return
- The equal algorithm returns a hpx::future<bool> if the execution policy is of type sequenced_task_policy or parallel_task_policy and returns bool otherwise. The equal algorithm returns true if the elements in the two ranges are equal, otherwise it returns false.
-
template<typename
ExPolicy
, typenameFwdIter1
, typenameFwdIter2
, typenameT
, typenameOp
>
std::enable_if<execution::is_execution_policy<ExPolicy>::value, typename util::detail::algorithm_result<ExPolicy, FwdIter2>::type>::typeexclusive_scan
(ExPolicy &&policy, FwdIter1 first, FwdIter1 last, FwdIter2 dest, T init, Op &&op)¶ Assigns through each iterator i in [result, result + (last - first)) the value of GENERALIZED_NONCOMMUTATIVE_SUM(binary_op, init, *first, …, *(first + (i - result) - 1)).
The reduce operations in the parallel
exclusive_scan algorithm invoked with an execution policy object of type sequenced_policy execute in sequential order in the calling thread.- Note
- Complexity: O(last - first) applications of the predicate op.
- Template Parameters
ExPolicy
: The type of the execution policy to use (deduced). It describes the manner in which the execution of the algorithm may be parallelized and the manner in which it executes the assignments.FwdIter1
: The type of the source iterators used (deduced). This iterator type must meet the requirements of an forward iterator.FwdIter2
: The type of the iterator representing the destination range (deduced). This iterator type must meet the requirements of an forward iterator.T
: The type of the value to be used as initial (and intermediate) values (deduced).Op
: The type of the binary function object used for the reduction operation.
- Parameters
policy
: The execution policy to use for the scheduling of the iterations.first
: Refers to the beginning of the sequence of elements the algorithm will be applied to.last
: Refers to the end of the sequence of elements the algorithm will be applied to.dest
: Refers to the beginning of the destination range.init
: The initial value for the generalized sum.op
: Specifies the function (or function object) which will be invoked for each of the values of the input sequence. This is a binary predicate. The signature of this predicate should be equivalent to:Ret fun(const Type1 &a, const Type1 &b);
The signature does not need to have const&, but the function must not modify the objects passed to it. The types
Type1 and Ret must be such that an object of a type as given by the input sequence can be implicitly converted to any of those types.
The reduce operations in the parallel exclusive_scan algorithm invoked with an execution policy object of type parallel_policy or parallel_task_policy are permitted to execute in an unordered fashion in unspecified threads, and indeterminately sequenced within each thread.
The difference between
exclusive_scan and inclusive_scan is that inclusive_scan includes the ith input element in the ith sum. If op is not mathematically associative, the behavior of inclusive_scan may be non-deterministic.- Return
- The copy_n algorithm returns a hpx::future<FwdIter2> if the execution policy is of type sequenced_task_policy or parallel_task_policy and returns FwdIter2 otherwise. The exclusive_scan algorithm returns the output iterator to the element in the destination range, one past the last element copied.
- Note
- GENERALIZED_NONCOMMUTATIVE_SUM(op, a1, …, aN) is defined as:
- a1 when N is 1
- op(GENERALIZED_NONCOMMUTATIVE_SUM(op, a1, …, aK), GENERALIZED_NONCOMMUTATIVE_SUM(op, aM, …, aN)) where 1 < K+1 = M <= N.
-
template<typename
ExPolicy
, typenameFwdIter1
, typenameFwdIter2
, typenameT
>
std::enable_if<execution::is_execution_policy<ExPolicy>::value, typename util::detail::algorithm_result<ExPolicy, FwdIter2>::type>::typeexclusive_scan
(ExPolicy &&policy, FwdIter1 first, FwdIter1 last, FwdIter2 dest, T init)¶ Assigns through each iterator i in [result, result + (last - first)) the value of GENERALIZED_NONCOMMUTATIVE_SUM(+, init, *first, …, *(first + (i - result) - 1))
The reduce operations in the parallel
exclusive_scan algorithm invoked with an execution policy object of type sequenced_policy execute in sequential order in the calling thread.- Note
- Complexity: O(last - first) applications of the predicate std::plus<T>.
- Template Parameters
ExPolicy
: The type of the execution policy to use (deduced). It describes the manner in which the execution of the algorithm may be parallelized and the manner in which it executes the assignments.FwdIter1
: The type of the source iterators used (deduced). This iterator type must meet the requirements of an forward iterator.FwdIter2
: The type of the iterator representing the destination range (deduced). This iterator type must meet the requirements of an forward iterator.T
: The type of the value to be used as initial (and intermediate) values (deduced).
- Parameters
policy
: The execution policy to use for the scheduling of the iterations.first
: Refers to the beginning of the sequence of elements the algorithm will be applied to.last
: Refers to the end of the sequence of elements the algorithm will be applied to.dest
: Refers to the beginning of the destination range.init
: The initial value for the generalized sum.
The reduce operations in the parallel exclusive_scan algorithm invoked with an execution policy object of type parallel_policy or parallel_task_policy are permitted to execute in an unordered fashion in unspecified threads, and indeterminately sequenced within each thread.
The difference between
exclusive_scan and inclusive_scan is that inclusive_scan includes the ith input element in the ith sum.- Return
- The copy_n algorithm returns a hpx::future<FwdIter2> if the execution policy is of type sequenced_task_policy or parallel_task_policy and returns FwdIter2 otherwise. The exclusive_scan algorithm returns the output iterator to the element in the destination range, one past the last element copied.
- Note
- GENERALIZED_NONCOMMUTATIVE_SUM(+, a1, …, aN) is defined as:
- a1 when N is 1
- GENERALIZED_NONCOMMUTATIVE_SUM(+, a1, …, aK)
- GENERALIZED_NONCOMMUTATIVE_SUM(+, aM, …, aN) where 1 < K+1 = M <= N.
-
template<typename
ExPolicy
, typenameFwdIter
, typenameT
>
util::detail::algorithm_result<ExPolicy>::typefill
(ExPolicy &&policy, FwdIter first, FwdIter last, T value)¶ Assigns the given value to the elements in the range [first, last).
The comparisons in the parallel
fill algorithm invoked with an execution policy object of type sequenced_policy execute in sequential order in the calling thread.- Note
- Complexity: Performs exactly last - first assignments.
- Template Parameters
ExPolicy
: The type of the execution policy to use (deduced). It describes the manner in which the execution of the algorithm may be parallelized and the manner in which it executes the assignments.FwdIter
: The type of the source iterators used (deduced). This iterator type must meet the requirements of an forward iterator.T
: The type of the value to be assigned (deduced).
- Parameters
policy
: The execution policy to use for the scheduling of the iterations.first
: Refers to the beginning of the sequence of elements the algorithm will be applied to.last
: Refers to the end of the sequence of elements the algorithm will be applied to.value
: The value to be assigned.
The comparisons in the parallel fill algorithm invoked with an execution policy object of type parallel_policy or parallel_task_policy are permitted to execute in an unordered fashion in unspecified threads, and indeterminately sequenced within each thread.
- Return
- The fill algorithm returns a hpx::future<void> if the execution policy is of type sequenced_task_policy or parallel_task_policy and returns difference_type otherwise (where difference_type is defined by void.
-
template<typename
ExPolicy
, typenameFwdIter
, typenameSize
, typenameT
>
util::detail::algorithm_result<ExPolicy, FwdIter>::typefill_n
(ExPolicy &&policy, FwdIter first, Size count, T value)¶ Assigns the given value value to the first count elements in the range beginning at first if count > 0. Does nothing otherwise.
The comparisons in the parallel
fill_n algorithm invoked with an execution policy object of type sequenced_policy execute in sequential order in the calling thread.- Note
- Complexity: Performs exactly count assignments, for count > 0.
- Template Parameters
ExPolicy
: The type of the execution policy to use (deduced). It describes the manner in which the execution of the algorithm may be parallelized and the manner in which it executes the assignments.FwdIter
: The type of the source iterators used (deduced). This iterator type must meet the requirements of an output iterator.Size
: The type of the argument specifying the number of elements to apply f to.T
: The type of the value to be assigned (deduced).
- Parameters
policy
: The execution policy to use for the scheduling of the iterations.first
: Refers to the beginning of the sequence of elements the algorithm will be applied to.count
: Refers to the number of elements starting at first the algorithm will be applied to.value
: The value to be assigned.
The comparisons in the parallel fill_n algorithm invoked with an execution policy object of type parallel_policy or parallel_task_policy are permitted to execute in an unordered fashion in unspecified threads, and indeterminately sequenced within each thread.
- Return
- The fill_n algorithm returns a hpx::future<void> if the execution policy is of type sequenced_task_policy or parallel_task_policy and returns difference_type otherwise (where difference_type is defined by void.
-
template<typename
ExPolicy
, typenameFwdIter
, typenameT
>
std::enable_if<execution::is_execution_policy<ExPolicy>::value, typename util::detail::algorithm_result<ExPolicy, FwdIter>::type>::typefind
(ExPolicy &&policy, FwdIter first, FwdIter last, T const &val)¶ Returns the first element in the range [first, last) that is equal to value
The comparison operations in the parallel
find algorithm invoked with an execution policy object of type sequenced_policy execute in sequential order in the calling thread.- Note
- Complexity: At most last - first applications of the operator==().
- Template Parameters
ExPolicy
: The type of the execution policy to use (deduced). It describes the manner in which the execution of the algorithm may be parallelized and the manner in which it executes the assignments.FwdIter
: The type of the source iterators used for the first range (deduced). This iterator type must meet the requirements of an forward iterator.T
: The type of the value to find (deduced).
- Parameters
policy
: The execution policy to use for the scheduling of the iterations.first
: Refers to the beginning of the sequence of elements of the first range the algorithm will be applied to.last
: Refers to the end of the sequence of elements of the first range the algorithm will be applied to.val
: the value to compare the elements to
The comparison operations in the parallel find algorithm invoked with an execution policy object of type parallel_policy or parallel_task_policy are permitted to execute in an unordered fashion in unspecified threads, and indeterminately sequenced within each thread.
- Return
- The find algorithm returns a hpx::future<FwdIter> if the execution policy is of type sequenced_task_policy or parallel_task_policy and returns FwdIter otherwise. The find algorithm returns the first element in the range [first,last) that is equal to val. If no such element in the range of [first,last) is equal to val, then the algorithm returns last.
-
template<typename
ExPolicy
, typenameFwdIter
, typenameF
>
std::enable_if<execution::is_execution_policy<ExPolicy>::value, typename util::detail::algorithm_result<ExPolicy, FwdIter>::type>::typefind_if
(ExPolicy &&policy, FwdIter first, FwdIter last, F &&f)¶ Returns the first element in the range [first, last) for which predicate f returns true
The comparison operations in the parallel
find_if algorithm invoked with an execution policy object of type sequenced_policy execute in sequential order in the calling thread.- Note
- Complexity: At most last - first applications of the predicate.
- Template Parameters
ExPolicy
: The type of the execution policy to use (deduced). It describes the manner in which the execution of the algorithm may be parallelized and the manner in which it executes the assignments.FwdIter
: The type of the source iterators used for the first range (deduced). This iterator type must meet the requirements of a forward iterator.F
: The type of the function/function object to use (deduced). Unlike its sequential form, the parallel overload of equal requires F to meet the requirements of CopyConstructible.
- Parameters
policy
: The execution policy to use for the scheduling of the iterations.first
: Refers to the beginning of the sequence of elements of the first range the algorithm will be applied to.last
: Refers to the end of the sequence of elements of the first range the algorithm will be applied to.f
: The unary predicate which returns true for the required element. The signature of the predicate should be equivalent to:bool pred(const Type &a);
The signature does not need to have const &, but the function must not modify the objects passed to it. The type
Type must be such that objects of type FwdIter can be dereferenced and then implicitly converted to Type.
The comparison operations in the parallel find_if algorithm invoked with an execution policy object of type parallel_policy or parallel_task_policy are permitted to execute in an unordered fashion in unspecified threads, and indeterminately sequenced within each thread.
- Return
- The find_if algorithm returns a hpx::future<FwdIter> if the execution policy is of type sequenced_task_policy or parallel_task_policy and returns FwdIter otherwise. The find_if algorithm returns the first element in the range [first,last) that satisfies the predicate f. If no such element exists that satisfies the predicate f, the algorithm returns last.
-
template<typename
ExPolicy
, typenameFwdIter
, typenameF
>
std::enable_if<execution::is_execution_policy<ExPolicy>::value, typename util::detail::algorithm_result<ExPolicy, FwdIter>::type>::typefind_if_not
(ExPolicy &&policy, FwdIter first, FwdIter last, F &&f)¶ Returns the first element in the range [first, last) for which predicate f returns false
The comparison operations in the parallel
find_if_not algorithm invoked with an execution policy object of type sequenced_policy execute in sequential order in the calling thread.- Note
- Complexity: At most last - first applications of the predicate.
- Template Parameters
ExPolicy
: The type of the execution policy to use (deduced). It describes the manner in which the execution of the algorithm may be parallelized and the manner in which it executes the assignments.FwdIter
: The type of the source iterators used for the first range (deduced). This iterator type must meet the requirements of a forward iterator.F
: The type of the function/function object to use (deduced). Unlike its sequential form, the parallel overload of equal requires F to meet the requirements of CopyConstructible.
- Parameters
policy
: The execution policy to use for the scheduling of the iterations.first
: Refers to the beginning of the sequence of elements of the first range the algorithm will be applied to.last
: Refers to the end of the sequence of elements of the first range the algorithm will be applied to.f
: The unary predicate which returns false for the required element. The signature of the predicate should be equivalent to:bool pred(const Type &a);
The signature does not need to have const &, but the function must not modify the objects passed to it. The type
Type must be such that objects of type FwdIter can be dereferenced and then implicitly converted to Type.
The comparison operations in the parallel find_if_not algorithm invoked with an execution policy object of type parallel_policy or parallel_task_policy are permitted to execute in an unordered fashion in unspecified threads, and indeterminately sequenced within each thread.
- Return
- The find_if_not algorithm returns a hpx::future<FwdIter> if the execution policy is of type sequenced_task_policy or parallel_task_policy and returns FwdIter otherwise. The find_if_not algorithm returns the first element in the range [first, last) that does not satisfy the predicate f. If no such element exists that does not satisfy the predicate f, the algorithm returns last.
-
template<typename
ExPolicy
, typenameFwdIter1
, typenameFwdIter2
, typenamePred
= detail::equal_to, typenameProj
= util::projection_identity>
std::enable_if<execution::is_execution_policy<ExPolicy>::value, typename util::detail::algorithm_result<ExPolicy, FwdIter1>::type>::typefind_end
(ExPolicy &&policy, FwdIter1 first1, FwdIter1 last1, FwdIter2 first2, FwdIter2 last2, Pred &&op = Pred(), Proj &&proj = Proj())¶ Returns the last subsequence of elements [first2, last2) found in the range [first, last) using the given predicate f to compare elements.
The comparison operations in the parallel
find_end algorithm invoked with an execution policy object of type sequenced_policy execute in sequential order in the calling thread.- Note
- Complexity: at most S*(N-S+1) comparisons where S = distance(first2, last2) and N = distance(first1, last1).
- Template Parameters
ExPolicy
: The type of the execution policy to use (deduced). It describes the manner in which the execution of the algorithm may be parallelized and the manner in which it executes the assignments.FwdIter1
: The type of the source iterators used for the first range (deduced). This iterator type must meet the requirements of an forward iterator.FwdIter2
: The type of the source iterators used for the second range (deduced). This iterator type must meet the requirements of an forward iterator.Pred
: The type of an optional function/function object to use. Unlike its sequential form, the parallel overload of replace requires Pred to meet the requirements of CopyConstructible. This defaults to std::equal_to<>Proj
: The type of an optional projection function. This defaults to util::projection_identity and is applied to the elements of type dereferenced FwdIter1 and dereferenced FwdIter2.
- Parameters
policy
: The execution policy to use for the scheduling of the iterations.first1
: Refers to the beginning of the sequence of elements of the first range the algorithm will be applied to.last1
: Refers to the end of the sequence of elements of the first range the algorithm will be applied to.first2
: Refers to the beginning of the sequence of elements the algorithm will be searching for.last2
: Refers to the end of the sequence of elements of the algorithm will be searching for.op
: The binary predicate which returns true if the elements should be treated as equal. The signature should be equivalent to the following:bool pred(const Type1 &a, const Type2 &b);
The signature does not need to have const &, but the function must not modify the objects passed to it. The types
Type1 and Type2 must be such that objects of types FwdIter1 and FwdIter2 can be dereferenced and then implicitly converted to Type1 and Type2 respectively.proj
: Specifies the function (or function object) which will be invoked for each of the elements of type dereferenced FwdIter1 and dereferenced FwdIter2 as a projection operation before the function f is invoked.
The comparison operations in the parallel find_end algorithm invoked with an execution policy object of type parallel_policy or parallel_task_policy are permitted to execute in an unordered fashion in unspecified threads, and indeterminately sequenced within each thread.
This overload of
find_end is available if the user decides to provide the algorithm their own predicate f.- Return
- The find_end algorithm returns a hpx::future<FwdIter> if the execution policy is of type sequenced_task_policy or parallel_task_policy and returns FwdIter otherwise. The find_end algorithm returns an iterator to the beginning of the last subsequence [first2, last2) in range [first, last). If the length of the subsequence [first2, last2) is greater than the length of the range [first1, last1), last1 is returned. Additionally if the size of the subsequence is empty or no subsequence is found, last1 is also returned.
-
template<typename
ExPolicy
, typenameFwdIter1
, typenameFwdIter2
, typenamePred
= detail::equal_to, typenameProj1
= util::projection_identity, typenameProj2
= util::projection_identity>
std::enable_if<execution::is_execution_policy<ExPolicy>::value, typename util::detail::algorithm_result<ExPolicy, FwdIter1>::type>::typefind_first_of
(ExPolicy &&policy, FwdIter1 first, FwdIter1 last, FwdIter2 s_first, FwdIter2 s_last, Pred &&op = Pred(), Proj1 &&proj1 = Proj1(), Proj2 &&proj2 = Proj2())¶ Searches the range [first, last) for any elements in the range [s_first, s_last). Uses binary predicate p to compare elements
The comparison operations in the parallel
find_first_of algorithm invoked with an execution policy object of type sequenced_policy execute in sequential order in the calling thread.- Note
- Complexity: at most (S*N) comparisons where S = distance(s_first, s_last) and N = distance(first, last).
- Template Parameters
ExPolicy
: The type of the execution policy to use (deduced). It describes the manner in which the execution of the algorithm may be parallelized and the manner in which it executes the assignments.FwdIter1
: The type of the source iterators used for the first range (deduced). This iterator type must meet the requirements of an forward iterator.FwdIter2
: The type of the source iterators used for the second range (deduced). This iterator type must meet the requirements of an forward iterator.Pred
: The type of an optional function/function object to use. Unlike its sequential form, the parallel overload of equal requires Pred to meet the requirements of CopyConstructible. This defaults to std::equal_to<>Proj1
: The type of an optional projection function. This defaults to util::projection_identity and is applied to the elements of type dereferenced FwdIter1.Proj2
: The type of an optional projection function. This defaults to util::projection_identity and is applied to the elements of type dereferenced FwdIter2.
- Parameters
policy
: The execution policy to use for the scheduling of the iterations.first
: Refers to the beginning of the sequence of elements of the first range the algorithm will be applied to.last
: Refers to the end of the sequence of elements of the first range the algorithm will be applied to.s_first
: Refers to the beginning of the sequence of elements the algorithm will be searching for.s_last
: Refers to the end of the sequence of elements of the algorithm will be searching for.op
: The binary predicate which returns true if the elements should be treated as equal. The signature should be equivalent to the following:bool pred(const Type1 &a, const Type2 &b);
The signature does not need to have const &, but the function must not modify the objects passed to it. The types
Type1 and Type2 must be such that objects of types FwdIter1 and FwdIter2 can be dereferenced and then implicitly converted to Type1 and Type2 respectively.proj1
: Specifies the function (or function object) which will be invoked for each of the elements of type dereferenced FwdIter1 as a projection operation before the function op is invoked.proj2
: Specifies the function (or function object) which will be invoked for each of the elements of type dereferenced FwdIter2 as a projection operation before the function op is invoked.
The comparison operations in the parallel find_first_of algorithm invoked with an execution policy object of type parallel_policy or parallel_task_policy are permitted to execute in an unordered fashion in unspecified threads, and indeterminately sequenced within each thread.
- Return
- The find_first_of algorithm returns a hpx::future<FwdIter1> if the execution policy is of type sequenced_task_policy or parallel_task_policy and returns FwdIter1 otherwise. The find_first_of algorithm returns an iterator to the first element in the range [first, last) that is equal to an element from the range [s_first, s_last). If the length of the subsequence [s_first, s_last) is greater than the length of the range [first, last), last is returned. Additionally if the size of the subsequence is empty or no subsequence is found, last is also returned. This overload of find_end is available if the user decides to provide the algorithm their own predicate f.
-
template<typename
ExPolicy
, typenameFwdIter
, typenameSize
, typenameF
, typenameProj
= util::projection_identity>
util::detail::algorithm_result<ExPolicy, FwdIter>::typefor_each_n
(ExPolicy &&policy, FwdIter first, Size count, F &&f, Proj &&proj = Proj())¶ Applies f to the result of dereferencing every iterator in the range [first, first + count), starting from first and proceeding to first + count - 1.
If
f returns a result, the result is ignored.- Note
- Complexity: Applies f exactly count times.
If the type of first satisfies the requirements of a mutable iterator, f may apply non-constant functions through the dereferenced iterator.
Unlike its sequential form, the parallel overload of for_each does not return a copy of its Function parameter, since parallelization may not permit efficient state accumulation.
The application of function objects in parallel algorithm invoked with an execution policy object of type
sequenced_policy execute in sequential order in the calling thread.- Template Parameters
ExPolicy
: The type of the execution policy to use (deduced). It describes the manner in which the execution of the algorithm may be parallelized and the manner in which it applies user-provided function objects.FwdIter
: The type of the source iterators used (deduced). This iterator type must meet the requirements of an forward iterator.Size
: The type of the argument specifying the number of elements to apply f to.F
: The type of the function/function object to use (deduced). Unlike its sequential form, the parallel overload of for_each requires F to meet the requirements of CopyConstructible.Proj
: The type of an optional projection function. This defaults to util::projection_identity
- Parameters
policy
: The execution policy to use for the scheduling of the iterations.first
: Refers to the beginning of the sequence of elements the algorithm will be applied to.count
: Refers to the number of elements starting at first the algorithm will be applied to.f
: Specifies the function (or function object) which will be invoked for each of the elements in the sequence specified by [first, last). The signature of this predicate should be equivalent to:<ignored> pred(const Type &a);
The signature does not need to have const&. The type
Type must be such that an object of type FwdIter can be dereferenced and then implicitly converted to Type.proj
: Specifies the function (or function object) which will be invoked for each of the elements as a projection operation before the actual predicate f is invoked.
The application of function objects in parallel algorithm invoked with an execution policy object of type parallel_policy or parallel_task_policy are permitted to execute in an unordered fashion in unspecified threads, and indeterminately sequenced within each thread.
- Return
- The for_each_n algorithm returns a hpx::future<FwdIter> if the execution policy is of type sequenced_task_policy or parallel_task_policy and returns FwdIter otherwise. It returns first + count for non-negative values of count and first for negative values.
-
template<typename
ExPolicy
, typenameFwdIter
, typenameF
, typenameProj
= util::projection_identity>
util::detail::algorithm_result<ExPolicy, FwdIter>::typefor_each
(ExPolicy &&policy, FwdIter first, FwdIter last, F &&f, Proj &&proj = Proj())¶ Applies f to the result of dereferencing every iterator in the range [first, last).
If
f returns a result, the result is ignored.- Note
- Complexity: Applies f exactly last - first times.
If the type of first satisfies the requirements of a mutable iterator, f may apply non-constant functions through the dereferenced iterator.
Unlike its sequential form, the parallel overload of for_each does not return a copy of its Function parameter, since parallelization may not permit efficient state accumulation.
The application of function objects in parallel algorithm invoked with an execution policy object of type
sequenced_policy execute in sequential order in the calling thread.- Template Parameters
ExPolicy
: The type of the execution policy to use (deduced). It describes the manner in which the execution of the algorithm may be parallelized and the manner in which it applies user-provided function objects.FwdIter
: The type of the source iterators used (deduced). This iterator type must meet the requirements of an forward iterator.F
: The type of the function/function object to use (deduced). Unlike its sequential form, the parallel overload of for_each requires F to meet the requirements of CopyConstructible.Proj
: The type of an optional projection function. This defaults to util::projection_identity
- Parameters
policy
: The execution policy to use for the scheduling of the iterations.first
: Refers to the beginning of the sequence of elements the algorithm will be applied to.last
: Refers to the end of the sequence of elements the algorithm will be applied to.f
: Specifies the function (or function object) which will be invoked for each of the elements in the sequence specified by [first, last). The signature of this predicate should be equivalent to:<ignored> pred(const Type &a);
The signature does not need to have const&. The type
Type must be such that an object of type FwdIter can be dereferenced and then implicitly converted to Type.proj
: Specifies the function (or function object) which will be invoked for each of the elements as a projection operation before the actual predicate f is invoked.
The application of function objects in parallel algorithm invoked with an execution policy object of type parallel_policy or parallel_task_policy are permitted to execute in an unordered fashion in unspecified threads, and indeterminately sequenced within each thread.
- Return
- The for_each algorithm returns a hpx::future<FwdIter> if the execution policy is of type sequenced_task_policy or parallel_task_policy and returns FwdIter otherwise. It returns last.
-
template<typename
ExPolicy
, typenameFwdIter
, typenameF
>
util::detail::algorithm_result<ExPolicy, FwdIter>::typegenerate
(ExPolicy &&policy, FwdIter first, FwdIter last, F &&f)¶ Assign each element in range [first, last) a value generated by the given function object f
The assignments in the parallel
generate algorithm invoked with an execution policy object of type sequenced_policy execute in sequential order in the calling thread.- Note
- Complexity: Exactly distance(first, last) invocations of f and assignments.
- Template Parameters
ExPolicy
: The type of the execution policy to use (deduced). It describes the manner in which the execution of the algorithm may be parallelized and the manner in which it executes the assignments.FwdIter
: The type of the source iterators used (deduced). This iterator type must meet the requirements of a forward iterator.F
: The type of the function/function object to use (deduced). Unlike its sequential form, the parallel overload of equal requires F to meet the requirements of CopyConstructible.
- Parameters
policy
: The execution policy to use for the scheduling of the iterations.first
: Refers to the beginning of the sequence of elements the algorithm will be applied to.last
: Refers to the end of the sequence of elements the algorithm will be applied to.f
: generator function that will be called. signature of function should be equivalent to the following:Ret fun();
The type
Ret must be such that an object of type FwdIter can be dereferenced and assigned a value of type Ret.
The assignments in the parallel generate algorithm invoked with an execution policy object of type parallel_policy or parallel_task_policy are permitted to execute in an unordered fashion in unspecified threads, and indeterminately sequenced within each thread.
- Return
- The replace_if algorithm returns a hpx::future<FwdIter> if the execution policy is of type sequenced_task_policy or parallel_task_policy and returns FwdIter otherwise. It returns last.
-
template<typename
ExPolicy
, typenameFwdIter
, typenameSize
, typenameF
>
util::detail::algorithm_result<ExPolicy, FwdIter>::typegenerate_n
(ExPolicy &&policy, FwdIter first, Size count, F &&f)¶ Assigns each element in range [first, first+count) a value generated by the given function object g.
The assignments in the parallel
generate_n algorithm invoked with an execution policy object of type sequenced_policy execute in sequential order in the calling thread.- Note
- Complexity: Exactly count invocations of f and assignments, for count > 0.
- Template Parameters
ExPolicy
: The type of the execution policy to use (deduced). It describes the manner in which the execution of the algorithm may be parallelized and the manner in which it executes the assignments.FwdIter
: The type of the source iterators used (deduced). This iterator type must meet the requirements of an forward iterator.F
: The type of the function/function object to use (deduced). Unlike its sequential form, the parallel overload of equal requires F to meet the requirements of CopyConstructible.
- Parameters
policy
: The execution policy to use for the scheduling of the iterations.first
: Refers to the beginning of the sequence of elements the algorithm will be applied to.count
: Refers to the number of elements in the sequence the algorithm will be applied to.f
: Refers to the generator function object that will be called. The signature of the function should be equivalent toRet fun();
The type
Ret must be such that an object of type OutputIt can be dereferenced and assigned a value of type Ret.
The assignments in the parallel generate_n algorithm invoked with an execution policy object of type parallel_policy or parallel_task_policy are permitted to execute in an unordered fashion in unspecified threads, and indeterminately sequenced within each thread.
- Return
- The replace_if algorithm returns a hpx::future<FwdIter> if the execution policy is of type sequenced_task_policy or parallel_task_policy and returns FwdIter otherwise. It returns last.
-
template<typename
ExPolicy
, typenameRandIter
, typenameComp
= detail::less, typenameProj
= util::projection_identity>
util::detail::algorithm_result<ExPolicy, bool>::typeis_heap
(ExPolicy &&policy, RandIter first, RandIter last, Comp &&comp = Comp(), Proj &&proj = Proj())¶ Returns whether the range is max heap. That is, true if the range is max heap, false otherwise. The function uses the given comparison function object comp (defaults to using operator<()).
comp has to induce a strict weak ordering on the values.
- Note
- Complexity: Performs at most N applications of the comparison comp, at most 2 * N applications of the projection proj, where N = last - first.
- Template Parameters
ExPolicy
: The type of the execution policy to use (deduced). It describes the manner in which the execution of the algorithm may be parallelized and the manner in which it executes the assignments.RandIter
: The type of the source iterators used (deduced). This iterator type must meet the requirements of a random access iterator.Comp
: The type of the function/function object to use (deduced).Proj
: The type of an optional projection function. This defaults to util::projection_identity
- Parameters
policy
: The execution policy to use for the scheduling of the iterations.first
: Refers to the beginning of the sequence of elements the algorithm will be applied to.last
: Refers to the end of the sequence of elements the algorithm will be applied to.comp
: comp is a callable object. The return value of the INVOKE operation applied to an object of type Comp, when contextually converted to bool, yields true if the first argument of the call is less than the second, and false otherwise. It is assumed that comp will not apply any non-constant function through the dereferenced iterator.proj
: Specifies the function (or function object) which will be invoked for each of the elements as a projection operation before the actual predicate is invoked.
The application of function objects in parallel algorithm invoked with an execution policy object of type sequenced_policy execute in sequential order in the calling thread.
The application of function objects in parallel algorithm invoked with an execution policy object of type parallel_policy or parallel_task_policy are permitted to execute in an unordered fashion in unspecified threads, and indeterminately sequenced within each thread.
- Return
- The is_heap algorithm returns a hpx::future<bool> if the execution policy is of type sequenced_task_policy or parallel_task_policy and returns bool otherwise. The is_heap algorithm returns whether the range is max heap. That is, true if the range is max heap, false otherwise.
-
template<typename
ExPolicy
, typenameRandIter
, typenameComp
= detail::less, typenameProj
= util::projection_identity>
util::detail::algorithm_result<ExPolicy, RandIter>::typeis_heap_until
(ExPolicy &&policy, RandIter first, RandIter last, Comp &&comp = Comp(), Proj &&proj = Proj())¶ Returns the upper bound of the largest range beginning at first which is a max heap. That is, the last iterator it for which range [first, it) is a max heap. The function uses the given comparison function object comp (defaults to using operator<()).
comp has to induce a strict weak ordering on the values.
- Note
- Complexity: Performs at most N applications of the comparison comp, at most 2 * N applications of the projection proj, where N = last - first.
- Template Parameters
ExPolicy
: The type of the execution policy to use (deduced). It describes the manner in which the execution of the algorithm may be parallelized and the manner in which it executes the assignments.RandIter
: The type of the source iterators used (deduced). This iterator type must meet the requirements of a random access iterator.Comp
: The type of the function/function object to use (deduced).Proj
: The type of an optional projection function. This defaults to util::projection_identity
- Parameters
policy
: The execution policy to use for the scheduling of the iterations.first
: Refers to the beginning of the sequence of elements the algorithm will be applied to.last
: Refers to the end of the sequence of elements the algorithm will be applied to.comp
: comp is a callable object. The return value of the INVOKE operation applied to an object of type Comp, when contextually converted to bool, yields true if the first argument of the call is less than the second, and false otherwise. It is assumed that comp will not apply any non-constant function through the dereferenced iterator.proj
: Specifies the function (or function object) which will be invoked for each of the elements as a projection operation before the actual predicate is invoked.
The application of function objects in parallel algorithm invoked with an execution policy object of type sequenced_policy execute in sequential order in the calling thread.
The application of function objects in parallel algorithm invoked with an execution policy object of type parallel_policy or parallel_task_policy are permitted to execute in an unordered fashion in unspecified threads, and indeterminately sequenced within each thread.
- Return
- The is_heap_until algorithm returns a hpx::future<RandIter> if the execution policy is of type sequenced_task_policy or parallel_task_policy and returns RandIter otherwise. The is_heap_until algorithm returns the upper bound of the largest range beginning at first which is a max heap. That is, the last iterator it for which range [first, it) is a max heap.
-
template<typename
ExPolicy
, typenameFwdIter1
, typenameFwdIter2
, typenamePred
= detail::less>
std::enable_if<execution::is_execution_policy<ExPolicy>::value, typename util::detail::algorithm_result<ExPolicy, bool>::type>::typeincludes
(ExPolicy &&policy, FwdIter1 first1, FwdIter1 last1, FwdIter2 first2, FwdIter2 last2, Pred &&op = Pred())¶ Returns true if every element from the sorted range [first2, last2) is found within the sorted range [first1, last1). Also returns true if [first2, last2) is empty. The version expects both ranges to be sorted with the user supplied binary predicate f.
The comparison operations in the parallel
includes algorithm invoked with an execution policy object of type sequenced_policy execute in sequential order in the calling thread.- Note
- At most 2*(N1+N2-1) comparisons, where N1 = std::distance(first1, last1) and N2 = std::distance(first2, last2).
- Template Parameters
ExPolicy
: The type of the execution policy to use (deduced). It describes the manner in which the execution of the algorithm may be parallelized and the manner in which it executes the assignments.FwdIter1
: The type of the source iterators used for the first range (deduced). This iterator type must meet the requirements of an forward iterator.FwdIter2
: The type of the source iterators used for the second range (deduced). This iterator type must meet the requirements of an forward iterator.Pred
: The type of an optional function/function object to use. Unlike its sequential form, the parallel overload of includes requires Pred to meet the requirements of CopyConstructible. This defaults to std::less<>
- Parameters
policy
: The execution policy to use for the scheduling of the iterations.first1
: Refers to the beginning of the sequence of elements of the first range the algorithm will be applied to.last1
: Refers to the end of the sequence of elements of the first range the algorithm will be applied to.first2
: Refers to the beginning of the sequence of elements of the second range the algorithm will be applied to.last2
: Refers to the end of the sequence of elements of the second range the algorithm will be applied to.op
: The binary predicate which returns true if the elements should be treated as includes. The signature of the predicate function should be equivalent to the following:bool pred(const Type1 &a, const Type2 &b);
The signature does not need to have const &, but the function must not modify the objects passed to it. The types
Type1 and Type2 must be such that objects of types FwdIter1 and FwdIter2 can be dereferenced and then implicitly converted to Type1 and Type2 respectively
The comparison operations in the parallel includes algorithm invoked with an execution policy object of type parallel_policy or parallel_task_policy are permitted to execute in an unordered fashion in unspecified threads, and indeterminately sequenced within each thread.
- Return
- The includes algorithm returns a hpx::future<bool> if the execution policy is of type sequenced_task_policy or parallel_task_policy and returns bool otherwise. The includes algorithm returns true every element from the sorted range [first2, last2) is found within the sorted range [first1, last1). Also returns true if [first2, last2) is empty.
-
template<typename
ExPolicy
, typenameFwdIter1
, typenameFwdIter2
, typenameOp
, typenameT
>
util::detail::algorithm_result<ExPolicy, FwdIter2>::typeinclusive_scan
(ExPolicy &&policy, FwdIter1 first, FwdIter1 last, FwdIter2 dest, Op &&op, T init)¶ Assigns through each iterator i in [result, result + (last - first)) the value of GENERALIZED_NONCOMMUTATIVE_SUM(op, init, *first, …, *(first + (i - result))).
The reduce operations in the parallel
inclusive_scan algorithm invoked with an execution policy object of type sequenced_policy execute in sequential order in the calling thread.- Note
- Complexity: O(last - first) applications of the predicate op.
- Template Parameters
ExPolicy
: The type of the execution policy to use (deduced). It describes the manner in which the execution of the algorithm may be parallelized and the manner in which it executes the assignments.FwdIter1
: The type of the source iterators used (deduced). This iterator type must meet the requirements of an forward iterator.FwdIter2
: The type of the iterator representing the destination range (deduced). This iterator type must meet the requirements of an forward iterator.T
: The type of the value to be used as initial (and intermediate) values (deduced).Op
: The type of the binary function object used for the reduction operation.
- Parameters
policy
: The execution policy to use for the scheduling of the iterations.first
: Refers to the beginning of the sequence of elements the algorithm will be applied to.last
: Refers to the end of the sequence of elements the algorithm will be applied to.dest
: Refers to the beginning of the destination range.init
: The initial value for the generalized sum.op
: Specifies the function (or function object) which will be invoked for each of the values of the input sequence. This is a binary predicate. The signature of this predicate should be equivalent to:Ret fun(const Type1 &a, const Type1 &b);
The signature does not need to have const&, but the function must not modify the objects passed to it. The types
Type1 and Ret must be such that an object of a type as given by the input sequence can be implicitly converted to any of those types.
The reduce operations in the parallel inclusive_scan algorithm invoked with an execution policy object of type parallel_policy or parallel_task_policy are permitted to execute in an unordered fashion in unspecified threads, and indeterminately sequenced within each thread.
The difference between
exclusive_scan and inclusive_scan is that inclusive_scan includes the ith input element in the ith sum. If op is not mathematically associative, the behavior of inclusive_scan may be non-deterministic.- Return
- The copy_n algorithm returns a hpx::future<FwdIter2> if the execution policy is of type sequenced_task_policy or parallel_task_policy and returns FwdIter2 otherwise. The inclusive_scan algorithm returns the output iterator to the element in the destination range, one past the last element copied.
- Note
- GENERALIZED_NONCOMMUTATIVE_SUM(op, a1, …, aN) is defined as:
- a1 when N is 1
- op(GENERALIZED_NONCOMMUTATIVE_SUM(op, a1, …, aK), GENERALIZED_NONCOMMUTATIVE_SUM(op, aM, …, aN)) where 1 < K+1 = M <= N.
-
template<typename
ExPolicy
, typenameFwdIter1
, typenameFwdIter2
, typenameOp
>
util::detail::algorithm_result<ExPolicy, FwdIter2>::typeinclusive_scan
(ExPolicy &&policy, FwdIter1 first, FwdIter1 last, FwdIter2 dest, Op &&op)¶ Assigns through each iterator i in [result, result + (last - first)) the value of GENERALIZED_NONCOMMUTATIVE_SUM(op, *first, …, *(first + (i - result))).
The reduce operations in the parallel
inclusive_scan algorithm invoked with an execution policy object of type sequenced_policy execute in sequential order in the calling thread.- Note
- Complexity: O(last - first) applications of the predicate op.
- Template Parameters
ExPolicy
: The type of the execution policy to use (deduced). It describes the manner in which the execution of the algorithm may be parallelized and the manner in which it executes the assignments.FwdIter1
: The type of the source iterators used (deduced). This iterator type must meet the requirements of an forward iterator.FwdIter2
: The type of the iterator representing the destination range (deduced). This iterator type must meet the requirements of an forward iterator.Op
: The type of the binary function object used for the reduction operation.
- Parameters
policy
: The execution policy to use for the scheduling of the iterations.first
: Refers to the beginning of the sequence of elements the algorithm will be applied to.last
: Refers to the end of the sequence of elements the algorithm will be applied to.dest
: Refers to the beginning of the destination range.op
: Specifies the function (or function object) which will be invoked for each of the values of the input sequence. This is a binary predicate. The signature of this predicate should be equivalent to:Ret fun(const Type1 &a, const Type1 &b);
The signature does not need to have const&, but the function must not modify the objects passed to it. The types
Type1 and Ret must be such that an object of a type as given by the input sequence can be implicitly converted to any of those types.
The reduce operations in the parallel inclusive_scan algorithm invoked with an execution policy object of type parallel_policy or parallel_task_policy are permitted to execute in an unordered fashion in unspecified threads, and indeterminately sequenced within each thread.
The difference between
exclusive_scan and inclusive_scan is that inclusive_scan includes the ith input element in the ith sum.- Return
- The copy_n algorithm returns a hpx::future<FwdIter2> if the execution policy is of type sequenced_task_policy or parallel_task_policy and returns FwdIter2 otherwise. The inclusive_scan algorithm returns the output iterator to the element in the destination range, one past the last element copied.
- Note
- GENERALIZED_NONCOMMUTATIVE_SUM(+, a1, …, aN) is defined as:
- a1 when N is 1
- GENERALIZED_NONCOMMUTATIVE_SUM(op, a1, …, aK)
- GENERALIZED_NONCOMMUTATIVE_SUM(+, aM, …, aN) where 1 < K+1 = M <= N.
-
template<typename
ExPolicy
, typenameFwdIter1
, typenameFwdIter2
>
std::enable_if<execution::is_execution_policy<ExPolicy>::value, typename util::detail::algorithm_result<ExPolicy, FwdIter2>::type>::typeinclusive_scan
(ExPolicy &&policy, FwdIter1 first, FwdIter1 last, FwdIter2 dest)¶ Assigns through each iterator i in [result, result + (last - first)) the value of gENERALIZED_NONCOMMUTATIVE_SUM(+, *first, …, *(first + (i - result))).
The reduce operations in the parallel
inclusive_scan algorithm invoked with an execution policy object of type sequenced_policy execute in sequential order in the calling thread.- Note
- Complexity: O(last - first) applications of the predicate op.
- Template Parameters
ExPolicy
: The type of the execution policy to use (deduced). It describes the manner in which the execution of the algorithm may be parallelized and the manner in which it executes the assignments.FwdIter1
: The type of the source iterators used (deduced). This iterator type must meet the requirements of an forward iterator.FwdIter2
: The type of the iterator representing the destination range (deduced). This iterator type must meet the requirements of an forward iterator.
- Parameters
policy
: The execution policy to use for the scheduling of the iterations.first
: Refers to the beginning of the sequence of elements the algorithm will be applied to.last
: Refers to the end of the sequence of elements the algorithm will be applied to.dest
: Refers to the beginning of the destination range.
The reduce operations in the parallel inclusive_scan algorithm invoked with an execution policy object of type parallel_policy or parallel_task_policy are permitted to execute in an unordered fashion in unspecified threads, and indeterminately sequenced within each thread.
The difference between
exclusive_scan and inclusive_scan is that inclusive_scan includes the ith input element in the ith sum.- Return
- The copy_n algorithm returns a hpx::future<FwdIter2> if the execution policy is of type sequenced_task_policy or parallel_task_policy and returns FwdIter2 otherwise. The inclusive_scan algorithm returns the output iterator to the element in the destination range, one past the last element copied.
- Note
- GENERALIZED_NONCOMMUTATIVE_SUM(+, a1, …, aN) is defined as:
- a1 when N is 1
- GENERALIZED_NONCOMMUTATIVE_SUM(+, a1, …, aK)
- GENERALIZED_NONCOMMUTATIVE_SUM(+, aM, …, aN) where 1 < K+1 = M <= N.
-
template<typename
ExPolicy
, typenameFwdIter
, typenamePred
>
std::enable_if<execution::is_execution_policy<ExPolicy>::value, typename util::detail::algorithm_result<ExPolicy, bool>::type>::typeis_partitioned
(ExPolicy &&policy, FwdIter first, FwdIter last, Pred &&pred)¶ Determines if the range [first, last) is partitioned.
The predicate operations in the parallel
is_partitioned algorithm invoked with an execution policy object of type sequenced_policy executes in sequential order in the calling thread.- Note
- Complexity: at most (N) predicate evaluations where N = distance(first, last).
- Template Parameters
ExPolicy
: The type of the execution policy to use (deduced). It describes the manner in which the execution of the algorithm may be parallelized and the manner in which it executes the assignments.FwdIter
: The type of the source iterators used for the This iterator type must meet the requirements of a forward iterator.
- Parameters
policy
: The execution policy to use for the scheduling of the iterations.first
: Refers to the beginning of the sequence of elements of that the algorithm will be applied to.last
: Refers to the end of the sequence of elements of that the algorithm will be applied to.pred
: Refers to the binary predicate which returns true if the first argument should be treated as less than the second argument. The signature of the function should be equivalent tobool pred(const Type &a, const Type &b);
The signature does not need to have const &, but the function must not modify the objects passed to it. The type
Type must be such that objects of types FwdIter can be dereferenced and then implicitly converted to Type.
The comparison operations in the parallel is_partitioned algorithm invoked with an execution policy object of type parallel_policy or parallel_task_policy are permitted to execute in an unordered fashion in unspecified threads, and indeterminately sequenced within each thread.
- Return
- The is_partitioned algorithm returns a hpx::future<bool> if the execution policy is of type task_execution_policy and returns bool otherwise. The is_partitioned algorithm returns true if each element in the sequence for which pred returns true precedes those for which pred returns false. Otherwise is_partitioned returns false. If the range [first, last) contains less than two elements, the function is always true.
-
template<typename
ExPolicy
, typenameFwdIter
, typenamePred
= detail::less>
std::enable_if<execution::is_execution_policy<ExPolicy>::value, typename util::detail::algorithm_result<ExPolicy, bool>::type>::typeis_sorted
(ExPolicy &&policy, FwdIter first, FwdIter last, Pred &&pred = Pred())¶ Determines if the range [first, last) is sorted. Uses pred to compare elements.
The comparison operations in the parallel
is_sorted algorithm invoked with an execution policy object of type sequenced_policy executes in sequential order in the calling thread.- Note
- Complexity: at most (N+S-1) comparisons where N = distance(first, last). S = number of partitions
- Template Parameters
ExPolicy
: The type of the execution policy to use (deduced). It describes the manner in which the execution of the algorithm may be parallelized and the manner in which it executes the assignments.FwdIter
: The type of the source iterators used for the This iterator type must meet the requirements of a forward iterator.Pred
: The type of an optional function/function object to use. Unlike its sequential form, the parallel overload of is_sorted requires Pred to meet the requirements of CopyConstructible. This defaults to std::less<>
- Parameters
policy
: The execution policy to use for the scheduling of the iterations.first
: Refers to the beginning of the sequence of elements of that the algorithm will be applied to.last
: Refers to the end of the sequence of elements of that the algorithm will be applied to.pred
: Refers to the binary predicate which returns true if the first argument should be treated as less than the second argument. The signature of the function should be equivalent tobool pred(const Type &a, const Type &b);
The signature does not need to have const &, but the function must not modify the objects passed to it. The type
Type must be such that objects of types FwdIter can be dereferenced and then implicitly converted to Type.
The comparison operations in the parallel is_sorted algorithm invoked with an execution policy object of type parallel_policy or parallel_task_policy are permitted to execute in an unordered fashion in unspecified threads, and indeterminately sequenced within each thread.
- Return
- The is_sorted algorithm returns a hpx::future<bool> if the execution policy is of type task_execution_policy and returns bool otherwise. The is_sorted algorithm returns a bool if each element in the sequence [first, last) satisfies the predicate passed. If the range [first, last) contains less than two elements, the function always returns true.
-
template<typename
ExPolicy
, typenameFwdIter
, typenamePred
= detail::less>
std::enable_if<execution::is_execution_policy<ExPolicy>::value, typename util::detail::algorithm_result<ExPolicy, FwdIter>::type>::typeis_sorted_until
(ExPolicy &&policy, FwdIter first, FwdIter last, Pred &&pred = Pred())¶ Returns the first element in the range [first, last) that is not sorted. Uses a predicate to compare elements or the less than operator.
The comparison operations in the parallel
is_sorted_until algorithm invoked with an execution policy object of type sequenced_policy executes in sequential order in the calling thread.- Note
- Complexity: at most (N+S-1) comparisons where N = distance(first, last). S = number of partitions
- Template Parameters
ExPolicy
: The type of the execution policy to use (deduced). It describes the manner in which the execution of the algorithm may be parallelized and the manner in which it executes the assignments.FwdIter
: The type of the source iterators used for the This iterator type must meet the requirements of a forward iterator.Pred
: The type of an optional function/function object to use. Unlike its sequential form, the parallel overload of is_sorted_until requires Pred to meet the requirements of CopyConstructible. This defaults to std::less<>
- Parameters
policy
: The execution policy to use for the scheduling of the iterations.first
: Refers to the beginning of the sequence of elements of that the algorithm will be applied to.last
: Refers to the end of the sequence of elements of that the algorithm will be applied to.pred
: Refers to the binary predicate which returns true if the first argument should be treated as less than the second argument. The signature of the function should be equivalent tobool pred(const Type &a, const Type &b);
The signature does not need to have const &, but the function must not modify the objects passed to it. The type
Type must be such that objects of types FwdIter can be dereferenced and then implicitly converted to Type.
The comparison operations in the parallel is_sorted_until algorithm invoked with an execution policy object of type parallel_policy or parallel_task_policy are permitted to execute in an unordered fashion in unspecified threads, and indeterminately sequenced within each thread.
- Return
- The is_sorted_until algorithm returns a hpx::future<FwdIter> if the execution policy is of type task_execution_policy and returns FwdIter otherwise. The is_sorted_until algorithm returns the first unsorted element. If the sequence has less than two elements or the sequence is sorted, last is returned.
-
template<typename
ExPolicy
, typenameFwdIter1
, typenameFwdIter2
, typenamePred
= detail::less>
std::enable_if<execution::is_execution_policy<ExPolicy>::value, typename util::detail::algorithm_result<ExPolicy, bool>::type>::typelexicographical_compare
(ExPolicy &&policy, FwdIter1 first1, FwdIter1 last1, FwdIter2 first2, FwdIter2 last2, Pred &&pred = Pred())¶ Checks if the first range [first1, last1) is lexicographically less than the second range [first2, last2). uses a provided predicate to compare elements.
The comparison operations in the parallel
lexicographical_compare algorithm invoked with an execution policy object of type sequenced_policy execute in sequential order in the calling thread.- Note
- Complexity: At most 2 * min(N1, N2) applications of the comparison operation, where N1 = std::distance(first1, last) and N2 = std::distance(first2, last2).
- Template Parameters
ExPolicy
: The type of the execution policy to use (deduced). It describes the manner in which the execution of the algorithm may be parallelized and the manner in which it executes the assignments.FwdIter1
: The type of the source iterators used for the first range (deduced). This iterator type must meet the requirements of an forward iterator.FwdIter2
: The type of the source iterators used for the second range (deduced). This iterator type must meet the requirements of an forward iterator.Pred
: The type of an optional function/function object to use. Unlike its sequential form, the parallel overload of lexicographical_compare requires Pred to meet the requirements of CopyConstructible. This defaults to std::less<>
- Parameters
policy
: The execution policy to use for the scheduling of the iterations.first1
: Refers to the beginning of the sequence of elements of the first range the algorithm will be applied to.last1
: Refers to the end of the sequence of elements of the first range the algorithm will be applied to.first2
: Refers to the beginning of the sequence of elements of the second range the algorithm will be applied to.last2
: Refers to the end of the sequence of elements of the second range the algorithm will be applied to.pred
: Refers to the comparison function that the first and second ranges will be applied to
The comparison operations in the parallel lexicographical_compare algorithm invoked with an execution policy object of type parallel_policy or parallel_task_policy are permitted to execute in an unordered fashion in unspecified threads, and indeterminately sequenced within each thread.
- Note
- Lexicographical comparison is an operation with the following properties
- Two ranges are compared element by element
- The first mismatching element defines which range is lexicographically less or greater than the other
- If one range is a prefix of another, the shorter range is lexicographically less than the other
- If two ranges have equivalent elements and are of the same length, then the ranges are lexicographically equal
- An empty range is lexicographically less than any non-empty range
- Two empty ranges are lexicographically equal
- Return
- The lexicographically_compare algorithm returns a hpx::future<bool> if the execution policy is of type sequenced_task_policy or parallel_task_policy and returns bool otherwise. The lexicographically_compare algorithm returns true if the first range is lexicographically less, otherwise it returns false. range [first2, last2), it returns false.
-
template<typename
ExPolicy
, typenameRandIter1
, typenameRandIter2
, typenameRandIter3
, typenameComp
= detail::less, typenameProj1
= util::projection_identity, typenameProj2
= util::projection_identity>
util::detail::algorithm_result<ExPolicy, hpx::util::tagged_tuple<tag::in1(RandIter1), tag::in2(RandIter2), tag::out(RandIter3)>>::typemerge
(ExPolicy &&policy, RandIter1 first1, RandIter1 last1, RandIter2 first2, RandIter2 last2, RandIter3 dest, Comp &&comp = Comp(), Proj1 &&proj1 = Proj1(), Proj2 &&proj2 = Proj2())¶ Merges two sorted ranges [first1, last1) and [first2, last2) into one sorted range beginning at dest. The order of equivalent elements in the each of original two ranges is preserved. For equivalent elements in the original two ranges, the elements from the first range precede the elements from the second range. The destination range cannot overlap with either of the input ranges.
The assignments in the parallel
merge algorithm invoked with an execution policy object of type sequenced_policy execute in sequential order in the calling thread.- Note
- Complexity: Performs O(std::distance(first1, last1) + std::distance(first2, last2)) applications of the comparison comp and the each projection.
- Template Parameters
ExPolicy
: The type of the execution policy to use (deduced). It describes the manner in which the execution of the algorithm may be parallelized and the manner in which it executes the assignments.RandIter1
: The type of the source iterators used (deduced) representing the first sorted range. This iterator type must meet the requirements of an random access iterator.RandIter2
: The type of the source iterators used (deduced) representing the second sorted range. This iterator type must meet the requirements of an random access iterator.RandIter3
: The type of the iterator representing the destination range (deduced). This iterator type must meet the requirements of an random access iterator.Comp
: The type of the function/function object to use (deduced). Unlike its sequential form, the parallel overload of merge requires Comp to meet the requirements of CopyConstructible. This defaults to std::less<>Proj1
: The type of an optional projection function to be used for elements of the first range. This defaults to util::projection_identityProj2
: The type of an optional projection function to be used for elements of the second range. This defaults to util::projection_identity
- Parameters
policy
: The execution policy to use for the scheduling of the iterations.first1
: Refers to the beginning of the first range of elements the algorithm will be applied to.last1
: Refers to the end of the first range of elements the algorithm will be applied to.first2
: Refers to the beginning of the second range of elements the algorithm will be applied to.last2
: Refers to the end of the second range of elements the algorithm will be applied to.dest
: Refers to the beginning of the destination range.comp
: comp is a callable object which returns true if the first argument is less than the second, and false otherwise. The signature of this comparison should be equivalent to:bool comp(const Type1 &a, const Type2 &b);
The signature does not need to have const&, but the function must not modify the objects passed to it. The types
Type1 and Type2 must be such that objects of types RandIter1 and RandIter2 can be dereferenced and then implicitly converted to both Type1 and Type2proj1
: Specifies the function (or function object) which will be invoked for each of the elements of the first range as a projection operation before the actual comparison comp is invoked.proj2
: Specifies the function (or function object) which will be invoked for each of the elements of the second range as a projection operation before the actual comparison comp is invoked.
The assignments in the parallel merge algorithm invoked with an execution policy object of type parallel_policy or parallel_task_policy are permitted to execute in an unordered fashion in unspecified threads, and indeterminately sequenced within each thread.
- Return
- The merge algorithm returns a hpx::future<tagged_tuple<tag::in1(RandIter1), tag::in2(RandIter2), tag::out(RandIter3)> > if the execution policy is of type sequenced_task_policy or parallel_task_policy and returns tagged_tuple<tag::in1(RandIter1), tag::in2(RandIter2), tag::out(RandIter3)> otherwise. The merge algorithm returns the tuple of the source iterator last1, the source iterator last2, the destination iterator to the end of the dest range.
-
template<typename
ExPolicy
, typenameRandIter
, typenameComp
= detail::less, typenameProj
= util::projection_identity>
util::detail::algorithm_result<ExPolicy, RandIter>::typeinplace_merge
(ExPolicy &&policy, RandIter first, RandIter middle, RandIter last, Comp &&comp = Comp(), Proj &&proj = Proj())¶ Merges two consecutive sorted ranges [first, middle) and [middle, last) into one sorted range [first, last). The order of equivalent elements in the each of original two ranges is preserved. For equivalent elements in the original two ranges, the elements from the first range precede the elements from the second range.
The assignments in the parallel
inplace_merge algorithm invoked with an execution policy object of type sequenced_policy execute in sequential order in the calling thread.- Note
- Complexity: Performs O(std::distance(first, last)) applications of the comparison comp and the each projection.
- Template Parameters
ExPolicy
: The type of the execution policy to use (deduced). It describes the manner in which the execution of the algorithm may be parallelized and the manner in which it executes the assignments.RandIter
: The type of the source iterators used (deduced). This iterator type must meet the requirements of an random access iterator.Comp
: The type of the function/function object to use (deduced). Unlike its sequential form, the parallel overload of inplace_merge requires Comp to meet the requirements of CopyConstructible. This defaults to std::less<>Proj
: The type of an optional projection function. This defaults to util::projection_identity
- Parameters
policy
: The execution policy to use for the scheduling of the iterations.first
: Refers to the beginning of the first sorted range the algorithm will be applied to.middle
: Refers to the end of the first sorted range and the beginning of the second sorted range the algorithm will be applied to.last
: Refers to the end of the second sorted range the algorithm will be applied to.comp
: comp is a callable object which returns true if the first argument is less than the second, and false otherwise. The signature of this comparison should be equivalent to:bool comp(const Type1 &a, const Type2 &b);
The signature does not need to have const&, but the function must not modify the objects passed to it. The types
Type1 and Type2 must be such that objects of types RandIter can be dereferenced and then implicitly converted to both Type1 and Type2proj
: Specifies the function (or function object) which will be invoked for each of the elements as a projection operation before the actual predicate is invoked.
The assignments in the parallel inplace_merge algorithm invoked with an execution policy object of type parallel_policy or parallel_task_policy are permitted to execute in an unordered fashion in unspecified threads, and indeterminately sequenced within each thread.
- Return
- The inplace_merge algorithm returns a hpx::future<RandIter> if the execution policy is of type sequenced_task_policy or parallel_task_policy and returns RandIter otherwise. The inplace_merge algorithm returns the source iterator last
-
template<typename
ExPolicy
, typenameFwdIter
, typenameProj
= util::projection_identity, typenameF
= detail::less>
util::detail::algorithm_result<ExPolicy, FwdIter>::typemin_element
(ExPolicy &&policy, FwdIter first, FwdIter last, F &&f = F(), Proj &&proj = Proj())¶ Finds the smallest element in the range [first, last) using the given comparison function f.
The comparisons in the parallel
min_element algorithm invoked with an execution policy object of type sequenced_policy execute in sequential order in the calling thread.- Note
- Complexity: Exactly max(N-1, 0) comparisons, where N = std::distance(first, last).
- Template Parameters
ExPolicy
: The type of the execution policy to use (deduced). It describes the manner in which the execution of the algorithm may be parallelized and the manner in which it executes the assignments.FwdIter
: The type of the source iterators used (deduced). This iterator type must meet the requirements of a forward iterator.F
: The type of the function/function object to use (deduced). Unlike its sequential form, the parallel overload of min_element requires F to meet the requirements of CopyConstructible.Proj
: The type of an optional projection function. This defaults to util::projection_identity
- Parameters
policy
: The execution policy to use for the scheduling of the iterations.first
: Refers to the beginning of the sequence of elements the algorithm will be applied to.last
: Refers to the end of the sequence of elements the algorithm will be applied to.f
: The binary predicate which returns true if the the left argument is less than the right element. The signature of the predicate function should be equivalent to the following:bool pred(const Type1 &a, const Type1 &b);
The signature does not need to have const &, but the function must not modify the objects passed to it. The type
Type1 must be such that objects of type FwdIter can be dereferenced and then implicitly converted to Type1.proj
: Specifies the function (or function object) which will be invoked for each of the elements as a projection operation before the actual predicate is invoked.
The comparisons in the parallel min_element algorithm invoked with an execution policy object of type parallel_policy or parallel_task_policy are permitted to execute in an unordered fashion in unspecified threads, and indeterminately sequenced within each thread.
- Return
- The min_element algorithm returns a hpx::future<FwdIter> if the execution policy is of type sequenced_task_policy or parallel_task_policy and returns FwdIter otherwise. The min_element algorithm returns the iterator to the smallest element in the range [first, last). If several elements in the range are equivalent to the smallest element, returns the iterator to the first such element. Returns last if the range is empty.
-
template<typename
ExPolicy
, typenameFwdIter
, typenameProj
= util::projection_identity, typenameF
= detail::less>
util::detail::algorithm_result<ExPolicy, FwdIter>::typemax_element
(ExPolicy &&policy, FwdIter first, FwdIter last, F &&f = F(), Proj &&proj = Proj())¶ Finds the greatest element in the range [first, last) using the given comparison function f.
The comparisons in the parallel
max_element algorithm invoked with an execution policy object of type sequenced_policy execute in sequential order in the calling thread.- Note
- Complexity: Exactly max(N-1, 0) comparisons, where N = std::distance(first, last).
- Template Parameters
ExPolicy
: The type of the execution policy to use (deduced). It describes the manner in which the execution of the algorithm may be parallelized and the manner in which it executes the assignments.FwdIter
: The type of the source iterators used (deduced). This iterator type must meet the requirements of a forward iterator.F
: The type of the function/function object to use (deduced). Unlike its sequential form, the parallel overload of max_element requires F to meet the requirements of CopyConstructible.Proj
: The type of an optional projection function. This defaults to util::projection_identity
- Parameters
policy
: The execution policy to use for the scheduling of the iterations.first
: Refers to the beginning of the sequence of elements the algorithm will be applied to.last
: Refers to the end of the sequence of elements the algorithm will be applied to.f
: The binary predicate which returns true if the This argument is optional and defaults to std::less. the left argument is less than the right element. The signature of the predicate function should be equivalent to the following:bool pred(const Type1 &a, const Type1 &b);
The signature does not need to have const &, but the function must not modify the objects passed to it. The type
Type1 must be such that objects of type FwdIter can be dereferenced and then implicitly converted to Type1.proj
: Specifies the function (or function object) which will be invoked for each of the elements as a projection operation before the actual predicate is invoked.
The comparisons in the parallel max_element algorithm invoked with an execution policy object of type parallel_policy or parallel_task_policy are permitted to execute in an unordered fashion in unspecified threads, and indeterminately sequenced within each thread.
- Return
- The max_element algorithm returns a hpx::future<FwdIter> if the execution policy is of type sequenced_task_policy or parallel_task_policy and returns FwdIter otherwise. The max_element algorithm returns the iterator to the smallest element in the range [first, last). If several elements in the range are equivalent to the smallest element, returns the iterator to the first such element. Returns last if the range is empty.
-
template<typename
ExPolicy
, typenameFwdIter
, typenameProj
= util::projection_identity, typenameF
= detail::less>
util::detail::algorithm_result<ExPolicy, hpx::util::tagged_pair<tag::min(FwdIter), tag::max(FwdIter)>>::typeminmax_element
(ExPolicy &&policy, FwdIter first, FwdIter last, F &&f = F(), Proj &&proj = Proj())¶ Finds the greatest element in the range [first, last) using the given comparison function f.
The comparisons in the parallel
minmax_element algorithm invoked with an execution policy object of type sequenced_policy execute in sequential order in the calling thread.- Note
- Complexity: At most max(floor(3/2*(N-1)), 0) applications of the predicate, where N = std::distance(first, last).
- Template Parameters
ExPolicy
: The type of the execution policy to use (deduced). It describes the manner in which the execution of the algorithm may be parallelized and the manner in which it executes the assignments.FwdIter
: The type of the source iterators used (deduced). This iterator type must meet the requirements of a forward iterator.F
: The type of the function/function object to use (deduced). Unlike its sequential form, the parallel overload of minmax_element requires F to meet the requirements of CopyConstructible.Proj
: The type of an optional projection function. This defaults to util::projection_identity
- Parameters
policy
: The execution policy to use for the scheduling of the iterations.first
: Refers to the beginning of the sequence of elements the algorithm will be applied to.last
: Refers to the end of the sequence of elements the algorithm will be applied to.f
: The binary predicate which returns true if the the left argument is less than the right element. This argument is optional and defaults to std::less. The signature of the predicate function should be equivalent to the following:bool pred(const Type1 &a, const Type1 &b);
The signature does not need to have const &, but the function must not modify the objects passed to it. The type
Type1 must be such that objects of type FwdIter can be dereferenced and then implicitly converted to Type1.proj
: Specifies the function (or function object) which will be invoked for each of the elements as a projection operation before the actual predicate is invoked.
The comparisons in the parallel minmax_element algorithm invoked with an execution policy object of type parallel_policy or parallel_task_policy are permitted to execute in an unordered fashion in unspecified threads, and indeterminately sequenced within each thread.
- Return
- The minmax_element algorithm returns a hpx::future<tagged_pair<tag::min(FwdIter), tag::max(FwdIter)> > if the execution policy is of type sequenced_task_policy or parallel_task_policy and returns tagged_pair<tag::min(FwdIter), tag::max(FwdIter)> otherwise. The minmax_element algorithm returns a pair consisting of an iterator to the smallest element as the first element and an iterator to the greatest element as the second. Returns std::make_pair(first, first) if the range is empty. If several elements are equivalent to the smallest element, the iterator to the first such element is returned. If several elements are equivalent to the largest element, the iterator to the last such element is returned.
-
template<typename
ExPolicy
, typenameFwdIter1
, typenameFwdIter2
, typenamePred
= detail::equal_to>
std::enable_if<execution::is_execution_policy<ExPolicy>::value, typename util::detail::algorithm_result<ExPolicy, std::pair<FwdIter1, FwdIter2>>::type>::typemismatch
(ExPolicy &&policy, FwdIter1 first1, FwdIter1 last1, FwdIter2 first2, FwdIter2 last2, Pred &&op = Pred())¶ Returns true if the range [first1, last1) is mismatch to the range [first2, last2), and false otherwise.
The comparison operations in the parallel
mismatch algorithm invoked with an execution policy object of type sequenced_policy execute in sequential order in the calling thread.- Note
- Complexity: At most min(last1 - first1, last2 - first2) applications of the predicate f. If FwdIter1 and FwdIter2 meet the requirements of RandomAccessIterator and (last1 - first1) != (last2 - first2) then no applications of the predicate f are made.
- Template Parameters
ExPolicy
: The type of the execution policy to use (deduced). It describes the manner in which the execution of the algorithm may be parallelized and the manner in which it executes the assignments.FwdIter1
: The type of the source iterators used for the first range (deduced). This iterator type must meet the requirements of an forward iterator.FwdIter2
: The type of the source iterators used for the second range (deduced). This iterator type must meet the requirements of an forward iterator.Pred
: The type of an optional function/function object to use. Unlike its sequential form, the parallel overload of mismatch requires Pred to meet the requirements of CopyConstructible. This defaults to std::equal_to<>
- Parameters
policy
: The execution policy to use for the scheduling of the iterations.first1
: Refers to the beginning of the sequence of elements of the first range the algorithm will be applied to.last1
: Refers to the end of the sequence of elements of the first range the algorithm will be applied to.first2
: Refers to the beginning of the sequence of elements of the second range the algorithm will be applied to.last2
: Refers to the end of the sequence of elements of the second range the algorithm will be applied to.op
: The binary predicate which returns true if the elements should be treated as mismatch. The signature of the predicate function should be equivalent to the following:bool pred(const Type1 &a, const Type2 &b);
The signature does not need to have const &, but the function must not modify the objects passed to it. The types
Type1 and Type2 must be such that objects of types FwdIter1 and FwdIter2 can be dereferenced and then implicitly converted to Type1 and Type2 respectively
The comparison operations in the parallel mismatch algorithm invoked with an execution policy object of type parallel_policy or parallel_task_policy are permitted to execute in an unordered fashion in unspecified threads, and indeterminately sequenced within each thread.
- Note
- The two ranges are considered mismatch if, for every iterator i in the range [first1,last1), *i mismatchs *(first2 + (i - first1)). This overload of mismatch uses operator== to determine if two elements are mismatch.
- Return
- The mismatch algorithm returns a hpx::future<bool> if the execution policy is of type sequenced_task_policy or parallel_task_policy and returns bool otherwise. The mismatch algorithm returns true if the elements in the two ranges are mismatch, otherwise it returns false. If the length of the range [first1, last1) does not mismatch the length of the range [first2, last2), it returns false.
-
template<typename
ExPolicy
, typenameFwdIter1
, typenameFwdIter2
, typenamePred
= detail::equal_to>
std::enable_if<execution::is_execution_policy<ExPolicy>::value, typename util::detail::algorithm_result<ExPolicy, std::pair<FwdIter1, FwdIter2>>::type>::typemismatch
(ExPolicy &&policy, FwdIter1 first1, FwdIter1 last1, FwdIter2 first2, Pred &&op = Pred())¶ Returns std::pair with iterators to the first two non-equivalent elements.
The comparison operations in the parallel
mismatch algorithm invoked with an execution policy object of type sequenced_policy execute in sequential order in the calling thread.- Note
- Complexity: At most last1 - first1 applications of the predicate f.
- Template Parameters
ExPolicy
: The type of the execution policy to use (deduced). It describes the manner in which the execution of the algorithm may be parallelized and the manner in which it executes the assignments.FwdIter1
: The type of the source iterators used for the first range (deduced). This iterator type must meet the requirements of an forward iterator.FwdIter2
: The type of the source iterators used for the second range (deduced). This iterator type must meet the requirements of an forward iterator.Pred
: The type of an optional function/function object to use. Unlike its sequential form, the parallel overload of mismatch requires Pred to meet the requirements of CopyConstructible. This defaults to std::equal_to<>
- Parameters
policy
: The execution policy to use for the scheduling of the iterations.first1
: Refers to the beginning of the sequence of elements of the first range the algorithm will be applied to.last1
: Refers to the end of the sequence of elements of the first range the algorithm will be applied to.first2
: Refers to the beginning of the sequence of elements of the second range the algorithm will be applied to.op
: The binary predicate which returns true if the elements should be treated as mismatch. The signature of the predicate function should be equivalent to the following:bool pred(const Type1 &a, const Type2 &b);
The signature does not need to have const &, but the function must not modify the objects passed to it. The types
Type1 and Type2 must be such that objects of types FwdIter1 and FwdIter2 can be dereferenced and then implicitly converted to Type1 and Type2 respectively
The comparison operations in the parallel mismatch algorithm invoked with an execution policy object of type parallel_policy or parallel_task_policy are permitted to execute in an unordered fashion in unspecified threads, and indeterminately sequenced within each thread.
- Return
- The mismatch algorithm returns a hpx::future<std::pair<FwdIter1, FwdIter2> > if the execution policy is of type sequenced_task_policy or parallel_task_policy and returns std::pair<FwdIter1, FwdIter2> otherwise. The mismatch algorithm returns the first mismatching pair of elements from two ranges: one defined by [first1, last1) and another defined by [first2, last2).
-
template<typename
ExPolicy
, typenameFwdIter1
, typenameFwdIter2
>
util::detail::algorithm_result<ExPolicy, hpx::util::tagged_pair<tag::in(FwdIter1), tag::out(FwdIter2)>>::typemove
(ExPolicy &&policy, FwdIter1 first, FwdIter1 last, FwdIter2 dest)¶ Moves the elements in the range [first, last), to another range beginning at dest. After this operation the elements in the moved-from range will still contain valid values of the appropriate type, but not necessarily the same values as before the move.
The move assignments in the parallel
move algorithm invoked with an execution policy object of type sequenced_policy execute in sequential order in the calling thread.- Note
- Complexity: Performs exactly last - first move assignments.
- Template Parameters
ExPolicy
: The type of the execution policy to use (deduced). It describes the manner in which the execution of the algorithm may be parallelized and the manner in which it executes the move assignments.FwdIter1
: The type of the source iterators used (deduced). This iterator type must meet the requirements of an forward iterator.FwdIter2
: The type of the iterator representing the destination range (deduced). This iterator type must meet the requirements of an forward iterator.
- Parameters
policy
: The execution policy to use for the scheduling of the iterations.first
: Refers to the beginning of the sequence of elements the algorithm will be applied to.last
: Refers to the end of the sequence of elements the algorithm will be applied to.dest
: Refers to the beginning of the destination range.
The move assignments in the parallel move algorithm invoked with an execution policy object of type parallel_policy or parallel_task_policy are permitted to execute in an unordered fashion in unspecified threads, and indeterminately sequenced within each thread.
- Return
- The move algorithm returns a hpx::future<tagged_pair<tag::in(FwdIter1), tag::out(FwdIter2)> > if the execution policy is of type sequenced_task_policy or parallel_task_policy and returns tagged_pair<tag::in(FwdIter1), tag::out(FwdIter2)> otherwise. The move algorithm returns the pair of the input iterator last and the output iterator to the element in the destination range, one past the last element moved.
-
template<typename
ExPolicy
, typenameBidirIter
, typenameF
, typenameProj
= util::projection_identity>
util::detail::algorithm_result<ExPolicy, BidirIter>::typestable_partition
(ExPolicy &&policy, BidirIter first, BidirIter last, F &&f, Proj &&proj = Proj())¶ Permutes the elements in the range [first, last) such that there exists an iterator i such that for every iterator j in the range [first, i) INVOKE(f, INVOKE (proj, *j)) != false, and for every iterator k in the range [i, last), INVOKE(f, INVOKE (proj, *k)) == false
The invocations of
f in the parallel stable_partition algorithm invoked with an execution policy object of type sequenced_policy executes in sequential order in the calling thread.- Note
- Complexity: At most (last - first) * log(last - first) swaps, but only linear number of swaps if there is enough extra memory. Exactly last - first applications of the predicate and projection.
- Template Parameters
ExPolicy
: The type of the execution policy to use (deduced). It describes the manner in which the execution of the algorithm may be parallelized and the manner in which it executes the invocations of f.BidirIter
: The type of the source iterators used (deduced). This iterator type must meet the requirements of an input iterator.F
: The type of the function/function object to use (deduced). Unlike its sequential form, the parallel overload of transform requires F to meet the requirements of CopyConstructible.Proj
: The type of an optional projection function. This defaults to util::projection_identity
- Parameters
policy
: The execution policy to use for the scheduling of the iterations.first
: Refers to the beginning of the sequence of elements the algorithm will be applied to.last
: Refers to the end of the sequence of elements the algorithm will be applied to.f
: Unary predicate which returns true if the element should be ordered before other elements. Specifies the function (or function object) which will be invoked for each of the elements in the sequence specified by [first, last). The signature of this predicate should be equivalent to:bool fun(const Type &a);
The signature does not need to have const&. The type
Type must be such that an object of type BidirIter can be dereferenced and then implicitly converted to Type.proj
: Specifies the function (or function object) which will be invoked for each of the elements as a projection operation before the actual predicate f is invoked.
The invocations of f in the parallel stable_partition algorithm invoked with an execution policy object of type parallel_policy or parallel_task_policy are permitted to execute in an unordered fashion in unspecified threads, and indeterminately sequenced within each thread.
- Return
- The stable_partition algorithm returns an iterator i such that for every iterator j in the range [first, i), f(*j) != false INVOKE(f, INVOKE(proj, *j)) != false, and for every iterator k in the range [i, last), f(*k) == false INVOKE(f, INVOKE (proj, *k)) == false. The relative order of the elements in both groups is preserved. If the execution policy is of type parallel_task_policy the algorithm returns a future<> referring to this iterator.
-
template<typename
ExPolicy
, typenameFwdIter
, typenamePred
, typenameProj
= util::projection_identity>
util::detail::algorithm_result<ExPolicy, FwdIter>::typepartition
(ExPolicy &&policy, FwdIter first, FwdIter last, Pred &&pred, Proj &&proj = Proj())¶ Reorders the elements in the range [first, last) in such a way that all elements for which the predicate pred returns true precede the elements for which the predicate pred returns false. Relative order of the elements is not preserved.
The assignments in the parallel
partition algorithm invoked with an execution policy object of type sequenced_policy execute in sequential order in the calling thread.- Note
- Complexity: At most 2 * (last - first) swaps. Exactly last - first applications of the predicate and projection.
- Template Parameters
ExPolicy
: The type of the execution policy to use (deduced). It describes the manner in which the execution of the algorithm may be parallelized and the manner in which it executes the assignments.FwdIter
: The type of the source iterators used (deduced). This iterator type must meet the requirements of an forward iterator.Pred
: The type of the function/function object to use (deduced). Unlike its sequential form, the parallel overload of partition requires Pred to meet the requirements of CopyConstructible.Proj
: The type of an optional projection function. This defaults to util::projection_identity
- Parameters
policy
: The execution policy to use for the scheduling of the iterations.first
: Refers to the beginning of the sequence of elements the algorithm will be applied to.last
: Refers to the end of the sequence of elements the algorithm will be applied to.pred
: Specifies the function (or function object) which will be invoked for each of the elements in the sequence specified by [first, last). This is an unary predicate for partitioning the source iterators. The signature of this predicate should be equivalent to:bool pred(const Type &a);
The signature does not need to have const&, but the function must not modify the objects passed to it. The type
Type must be such that an object of type InIter can be dereferenced and then implicitly converted to Type.proj
: Specifies the function (or function object) which will be invoked for each of the elements as a projection operation before the actual predicate is invoked.
The assignments in the parallel partition algorithm invoked with an execution policy object of type parallel_policy or parallel_task_policy are permitted to execute in an unordered fashion in unspecified threads, and indeterminately sequenced within each thread.
- Return
- The partition algorithm returns a hpx::future<FwdIter> if the execution policy is of type parallel_task_policy and returns FwdIter otherwise. The partition algorithm returns the iterator to the first element of the second group.
-
template<typename
ExPolicy
, typenameFwdIter1
, typenameFwdIter2
, typenameFwdIter3
, typenamePred
, typenameProj
= util::projection_identity>
util::detail::algorithm_result<ExPolicy, hpx::util::tagged_tuple<tag::in(FwdIter1), tag::out1(FwdIter2), tag::out2(FwdIter3)>>::typepartition_copy
(ExPolicy &&policy, FwdIter1 first, FwdIter1 last, FwdIter2 dest_true, FwdIter3 dest_false, Pred &&pred, Proj &&proj = Proj())¶ Copies the elements in the range, defined by [first, last), to two different ranges depending on the value returned by the predicate pred. The elements, that satisfy the predicate pred, are copied to the range beginning at dest_true. The rest of the elements are copied to the range beginning at dest_false. The order of the elements is preserved.
The assignments in the parallel
partition_copy algorithm invoked with an execution policy object of type sequenced_policy execute in sequential order in the calling thread.- Note
- Complexity: Performs not more than last - first assignments, exactly last - first applications of the predicate f.
- Template Parameters
ExPolicy
: The type of the execution policy to use (deduced). It describes the manner in which the execution of the algorithm may be parallelized and the manner in which it executes the assignments.FwdIter1
: The type of the source iterators used (deduced). This iterator type must meet the requirements of an forward iterator.FwdIter2
: The type of the iterator representing the destination range for the elements that satisfy the predicate pred (deduced). This iterator type must meet the requirements of an forward iterator.FwdIter3
: The type of the iterator representing the destination range for the elements that don’t satisfy the predicate pred (deduced). This iterator type must meet the requirements of an forward iterator.Pred
: The type of the function/function object to use (deduced). Unlike its sequential form, the parallel overload of partition_copy requires Pred to meet the requirements of CopyConstructible.Proj
: The type of an optional projection function. This defaults to util::projection_identity
- Parameters
policy
: The execution policy to use for the scheduling of the iterations.first
: Refers to the beginning of the sequence of elements the algorithm will be applied to.last
: Refers to the end of the sequence of elements the algorithm will be applied to.dest_true
: Refers to the beginning of the destination range for the elements that satisfy the predicate pred.dest_false
: Refers to the beginning of the destination range for the elements that don’t satisfy the predicate pred.pred
: Specifies the function (or function object) which will be invoked for each of the elements in the sequence specified by [first, last). This is an unary predicate for partitioning the source iterators. The signature of this predicate should be equivalent to:bool pred(const Type &a);
The signature does not need to have const&, but the function must not modify the objects passed to it. The type
Type must be such that an object of type FwdIter1 can be dereferenced and then implicitly converted to Type.proj
: Specifies the function (or function object) which will be invoked for each of the elements as a projection operation before the actual predicate is invoked.
The assignments in the parallel partition_copy algorithm invoked with an execution policy object of type parallel_policy or parallel_task_policy are permitted to execute in an unordered fashion in unspecified threads, and indeterminately sequenced within each thread.
- Return
- The partition_copy algorithm returns a hpx::future<tagged_tuple<tag::in(InIter), tag::out1(OutIter1), tag::out2(OutIter2)> > if the execution policy is of type parallel_task_policy and returns tagged_tuple<tag::in(InIter), tag::out1(OutIter1), tag::out2(OutIter2)> otherwise. The partition_copy algorithm returns the tuple of the source iterator last, the destination iterator to the end of the dest_true range, and the destination iterator to the end of the dest_false range.
-
template<typename
ExPolicy
, typenameFwdIterB
, typenameFwdIterE
, typenameT
, typenameF
>
std::enable_if<execution::is_execution_policy<ExPolicy>::value, typename util::detail::algorithm_result<ExPolicy, T>::type>::typereduce
(ExPolicy &&policy, FwdIterB first, FwdIterE last, T init, F &&f)¶ Returns GENERALIZED_SUM(f, init, *first, …, *(first + (last - first) - 1)).
The reduce operations in the parallel
reduce algorithm invoked with an execution policy object of type sequenced_policy execute in sequential order in the calling thread.- Note
- Complexity: O(last - first) applications of the predicate f.
- Template Parameters
ExPolicy
: The type of the execution policy to use (deduced). It describes the manner in which the execution of the algorithm may be parallelized and the manner in which it executes the assignments.FwdIterB
: The type of the source begin iterator used (deduced). This iterator type must meet the requirements of an forward iterator.FwdIterE
: The type of the source end iterator used (deduced). This iterator type must meet the requirements of an forward iterator.F
: The type of the function/function object to use (deduced). Unlike its sequential form, the parallel overload of copy_if requires F to meet the requirements of CopyConstructible.T
: The type of the value to be used as initial (and intermediate) values (deduced).
- Parameters
policy
: The execution policy to use for the scheduling of the iterations.first
: Refers to the beginning of the sequence of elements the algorithm will be applied to.last
: Refers to the end of the sequence of elements the algorithm will be applied to.f
: Specifies the function (or function object) which will be invoked for each of the elements in the sequence specified by [first, last). This is a binary predicate. The signature of this predicate should be equivalent to:Ret fun(const Type1 &a, const Type1 &b);
The signature does not need to have const&. The types
Type1 Ret must be such that an object of type FwdIterB can be dereferenced and then implicitly converted to any of those types.init
: The initial value for the generalized sum.
The reduce operations in the parallel copy_if algorithm invoked with an execution policy object of type parallel_policy or parallel_task_policy are permitted to execute in an unordered fashion in unspecified threads, and indeterminately sequenced within each thread.
The difference between
reduce and accumulate is that the behavior of reduce may be non-deterministic for non-associative or non-commutative binary predicate.- Return
- The reduce algorithm returns a hpx::future<T> if the execution policy is of type sequenced_task_policy or parallel_task_policy and returns T otherwise. The reduce algorithm returns the result of the generalized sum over the elements given by the input range [first, last).
- Note
- GENERALIZED_SUM(op, a1, …, aN) is defined as follows:
- a1 when N is 1
- op(GENERALIZED_SUM(op, b1, …, bK), GENERALIZED_SUM(op, bM, …, bN)), where:
- b1, …, bN may be any permutation of a1, …, aN and
- 1 < K+1 = M <= N.
-
template<typename
ExPolicy
, typenameFwdIterB
, typenameFwdIterE
, typenameT
>
std::enable_if<execution::is_execution_policy<ExPolicy>::value, typename util::detail::algorithm_result<ExPolicy, T>::type>::typereduce
(ExPolicy &&policy, FwdIterB first, FwdIterE last, T init)¶ Returns GENERALIZED_SUM(+, init, *first, …, *(first + (last - first) - 1)).
The reduce operations in the parallel
reduce algorithm invoked with an execution policy object of type sequenced_policy execute in sequential order in the calling thread.- Note
- Complexity: O(last - first) applications of the operator+().
- Template Parameters
ExPolicy
: The type of the execution policy to use (deduced). It describes the manner in which the execution of the algorithm may be parallelized and the manner in which it executes the assignments.FwdIterB
: The type of the source begin iterator used (deduced). This iterator type must meet the requirements of an forward iterator.FwdIterE
: The type of the source end iterator used (deduced). This iterator type must meet the requirements of an forward iterator.T
: The type of the value to be used as initial (and intermediate) values (deduced).
- Parameters
policy
: The execution policy to use for the scheduling of the iterations.first
: Refers to the beginning of the sequence of elements the algorithm will be applied to.last
: Refers to the end of the sequence of elements the algorithm will be applied to.init
: The initial value for the generalized sum.
The reduce operations in the parallel copy_if algorithm invoked with an execution policy object of type parallel_policy or parallel_task_policy are permitted to execute in an unordered fashion in unspecified threads, and indeterminately sequenced within each thread.
The difference between
reduce and accumulate is that the behavior of reduce may be non-deterministic for non-associative or non-commutative binary predicate.- Return
- The reduce algorithm returns a hpx::future<T> if the execution policy is of type sequenced_task_policy or parallel_task_policy and returns T otherwise. The reduce algorithm returns the result of the generalized sum (applying operator+()) over the elements given by the input range [first, last).
- Note
- GENERALIZED_SUM(+, a1, …, aN) is defined as follows:
- a1 when N is 1
- op(GENERALIZED_SUM(+, b1, …, bK), GENERALIZED_SUM(+, bM, …, bN)), where:
- b1, …, bN may be any permutation of a1, …, aN and
- 1 < K+1 = M <= N.
-
template<typename
ExPolicy
, typenameFwdIterB
, typenameFwdIterE
>
std::enable_if<execution::is_execution_policy<ExPolicy>::value, typename util::detail::algorithm_result<ExPolicy, typename std::iterator_traits<FwdIterB>::value_type>::type>::typereduce
(ExPolicy &&policy, FwdIterB first, FwdIterE last)¶ Returns GENERALIZED_SUM(+, T(), *first, …, *(first + (last - first) - 1)).
The reduce operations in the parallel
reduce algorithm invoked with an execution policy object of type sequenced_policy execute in sequential order in the calling thread.- Note
- Complexity: O(last - first) applications of the operator+().
- Template Parameters
ExPolicy
: The type of the execution policy to use (deduced). It describes the manner in which the execution of the algorithm may be parallelized and the manner in which it executes the assignments.FwdIterB
: The type of the source begin iterator used (deduced). This iterator type must meet the requirements of an forward iterator.FwdIterE
: The type of the source end iterator used (deduced). This iterator type must meet the requirements of an forward iterator.
- Parameters
policy
: The execution policy to use for the scheduling of the iterations.first
: Refers to the beginning of the sequence of elements the algorithm will be applied to.last
: Refers to the end of the sequence of elements the algorithm will be applied to.
The reduce operations in the parallel copy_if algorithm invoked with an execution policy object of type parallel_policy or parallel_task_policy are permitted to execute in an unordered fashion in unspecified threads, and indeterminately sequenced within each thread.
The difference between
reduce and accumulate is that the behavior of reduce may be non-deterministic for non-associative or non-commutative binary predicate.- Return
- The reduce algorithm returns a hpx::future<T> if the execution policy is of type sequenced_task_policy or parallel_task_policy and returns T otherwise (where T is the value_type of FwdIterB). The reduce algorithm returns the result of the generalized sum (applying operator+()) over the elements given by the input range [first, last).
- Note
- The type of the initial value (and the result type) T is determined from the value_type of the used FwdIterB.
- Note
- GENERALIZED_SUM(+, a1, …, aN) is defined as follows:
- a1 when N is 1
- op(GENERALIZED_SUM(+, b1, …, bK), GENERALIZED_SUM(+, bM, …, bN)), where:
- b1, …, bN may be any permutation of a1, …, aN and
- 1 < K+1 = M <= N.
-
template<typename
ExPolicy
, typenameRanIter
, typenameRanIter2
, typenameFwdIter1
, typenameFwdIter2
, typenameCompare
= std::equal_to<typename std::iterator_traits<RanIter>::value_type>, typenameFunc
= std::plus<typename std::iterator_traits<RanIter2>::value_type>>
util::detail::algorithm_result<ExPolicy, std::pair<FwdIter1, FwdIter2>>::typereduce_by_key
(ExPolicy &&policy, RanIter key_first, RanIter key_last, RanIter2 values_first, FwdIter1 keys_output, FwdIter2 values_output, Compare &&comp = Compare(), Func &&func = Func())¶ Reduce by Key performs an inclusive scan reduction operation on elements supplied in key/value pairs. The algorithm produces a single output value for each set of equal consecutive keys in [key_first, key_last). the value being the GENERALIZED_NONCOMMUTATIVE_SUM(op, init, *first, …, *(first + (i - result))). for the run of consecutive matching keys. The number of keys supplied must match the number of values.
comp has to induce a strict weak ordering on the values.
- Note
- Complexity: O(last - first) applications of the predicate op.
- Template Parameters
ExPolicy
: The type of the execution policy to use (deduced). It describes the manner in which the execution of the algorithm may be parallelized and the manner in which it applies user-provided function objects.RanIter
: The type of the key iterators used (deduced). This iterator type must meet the requirements of a random access iterator.RanIter2
: The type of the value iterators used (deduced). This iterator type must meet the requirements of a random access iterator.FwdIter1
: The type of the iterator representing the destination key range (deduced). This iterator type must meet the requirements of an forward iterator.FwdIter2
: The type of the iterator representing the destination value range (deduced). This iterator type must meet the requirements of an forward iterator.Compare
: The type of the optional function/function object to use to compare keys (deduced). Assumed to be std::equal_to otherwise.Func
: The type of the function/function object to use (deduced). Unlike its sequential form, the parallel overload of copy_if requires F to meet the requirements of CopyConstructible.
- Parameters
policy
: The execution policy to use for the scheduling of the iterations.key_first
: Refers to the beginning of the sequence of key elements the algorithm will be applied to.key_last
: Refers to the end of the sequence of key elements the algorithm will be applied to.values_first
: Refers to the beginning of the sequence of value elements the algorithm will be applied to.keys_output
: Refers to the start output location for the keys produced by the algorithm.values_output
: Refers to the start output location for the values produced by the algorithm.comp
: comp is a callable object. The return value of the INVOKE operation applied to an object of type Comp, when contextually converted to bool, yields true if the first argument of the call is less than the second, and false otherwise. It is assumed that comp will not apply any non-constant function through the dereferenced iterator.func
: Specifies the function (or function object) which will be invoked for each of the elements in the sequence specified by [first, last). This is a binary predicate. The signature of this predicate should be equivalent to:Ret fun(const Type1 &a, const Type1 &b);
The signature does not need to have const&. The types
Type1 Ret must be such that an object of type FwdIter can be dereferenced and then implicitly converted to any of those types.
The application of function objects in parallel algorithm invoked with an execution policy object of type sequenced_policy execute in sequential order in the calling thread.
The application of function objects in parallel algorithm invoked with an execution policy object of type parallel_policy or parallel_task_policy are permitted to execute in an unordered fashion in unspecified threads, and indeterminately sequenced within each thread.
- Return
- The reduce_by_key algorithm returns a hpx::future<pair<Iter1,Iter2>> if the execution policy is of type sequenced_task_policy or parallel_task_policy and returns pair<Iter1,Iter2> otherwise.
-
template<typename
ExPolicy
, typenameFwdIter
, typenamePred
, typenameProj
= util::projection_identity>
util::detail::algorithm_result<ExPolicy, FwdIter>::typeremove_if
(ExPolicy &&policy, FwdIter first, FwdIter last, Pred &&pred, Proj &&proj = Proj())¶ Removes all elements satisfying specific criteria from the range [first, last) and returns a past-the-end iterator for the new end of the range. This version removes all elements for which predicate pred returns true.
The assignments in the parallel
remove_if algorithm invoked with an execution policy object of type sequenced_policy execute in sequential order in the calling thread.- Note
- Complexity: Performs not more than last - first assignments, exactly last - first applications of the predicate pred and the projection proj.
- Template Parameters
ExPolicy
: The type of the execution policy to use (deduced). It describes the manner in which the execution of the algorithm may be parallelized and the manner in which it executes the assignments.FwdIter
: The type of the source iterators used (deduced). This iterator type must meet the requirements of an forward iterator.Pred
: The type of the function/function object to use (deduced). Unlike its sequential form, the parallel overload of remove_if requires Pred to meet the requirements of CopyConstructible.Proj
: The type of an optional projection function. This defaults to util::projection_identity
- Parameters
policy
: The execution policy to use for the scheduling of the iterations.first
: Refers to the beginning of the sequence of elements the algorithm will be applied to.last
: Refers to the end of the sequence of elements the algorithm will be applied to.pred
: Specifies the function (or function object) which will be invoked for each of the elements in the sequence specified by [first, last).This is an unary predicate which returns true for the required elements. The signature of this predicate should be equivalent to:bool pred(const Type &a);
The signature does not need to have const&, but the function must not modify the objects passed to it. The type
Type must be such that an object of type FwdIter can be dereferenced and then implicitly converted to Type.proj
: Specifies the function (or function object) which will be invoked for each of the elements as a projection operation before the actual predicate is invoked.
The assignments in the parallel remove_if algorithm invoked with an execution policy object of type parallel_policy or parallel_task_policy are permitted to execute in an unordered fashion in unspecified threads, and indeterminately sequenced within each thread.
- Return
- The remove_if algorithm returns a hpx::future<FwdIter> if the execution policy is of type sequenced_task_policy or parallel_task_policy and returns FwdIter otherwise. The remove_if algorithm returns the iterator to the new end of the range.
-
template<typename
ExPolicy
, typenameFwdIter
, typenameT
, typenameProj
= util::projection_identity>
util::detail::algorithm_result<ExPolicy, FwdIter>::typeremove
(ExPolicy &&policy, FwdIter first, FwdIter last, T const &value, Proj &&proj = Proj())¶ Removes all elements satisfying specific criteria from the range [first, last) and returns a past-the-end iterator for the new end of the range. This version removes all elements that are equal to value.
The assignments in the parallel
remove algorithm invoked with an execution policy object of type sequenced_policy execute in sequential order in the calling thread.- Note
- Complexity: Performs not more than last - first assignments, exactly last - first applications of the operator==() and the projection proj.
- Template Parameters
ExPolicy
: The type of the execution policy to use (deduced). It describes the manner in which the execution of the algorithm may be parallelized and the manner in which it executes the assignments.FwdIter
: The type of the source iterators used (deduced). This iterator type must meet the requirements of an forward iterator.T
: The type of the value to remove (deduced). This value type must meet the requirements of CopyConstructible.Proj
: The type of an optional projection function. This defaults to util::projection_identity
- Parameters
policy
: The execution policy to use for the scheduling of the iterations.first
: Refers to the beginning of the sequence of elements the algorithm will be applied to.last
: Refers to the end of the sequence of elements the algorithm will be applied to.value
: Specifies the value of elements to remove.proj
: Specifies the function (or function object) which will be invoked for each of the elements as a projection operation before the actual predicate is invoked.
The assignments in the parallel remove algorithm invoked with an execution policy object of type parallel_policy or parallel_task_policy are permitted to execute in an unordered fashion in unspecified threads, and indeterminately sequenced within each thread.
- Return
- The remove algorithm returns a hpx::future<FwdIter> if the execution policy is of type sequenced_task_policy or parallel_task_policy and returns FwdIter otherwise. The remove algorithm returns the iterator to the new end of the range.
-
template<typename
ExPolicy
, typenameFwdIter1
, typenameFwdIter2
, typenameT
, typenameProj
= util::projection_identity>
util::detail::algorithm_result<ExPolicy, hpx::util::tagged_pair<tag::in(FwdIter1), tag::out(FwdIter2)>>::typeremove_copy
(ExPolicy &&policy, FwdIter1 first, FwdIter1 last, FwdIter2 dest, T const &val, Proj &&proj = Proj())¶ Copies the elements in the range, defined by [first, last), to another range beginning at dest. Copies only the elements for which the comparison operator returns false when compare to val. The order of the elements that are not removed is preserved.
Effects: Copies all the elements referred to by the iterator it in the range [first,last) for which the following corresponding conditions do not hold: INVOKE(proj, *it) == value
The assignments in the parallel
remove_copy algorithm invoked with an execution policy object of type sequenced_policy execute in sequential order in the calling thread.- Note
- Complexity: Performs not more than last - first assignments, exactly last - first applications of the predicate f.
- Template Parameters
ExPolicy
: The type of the execution policy to use (deduced). It describes the manner in which the execution of the algorithm may be parallelized and the manner in which it executes the assignments.FwdIter1
: The type of the source iterators used (deduced). This iterator type must meet the requirements of an forward iterator.FwdIter2
: The type of the iterator representing the destination range (deduced). This iterator type must meet the requirements of an forward iterator.T
: The type that the result of dereferencing FwdIter1 is compared to.Proj
: The type of an optional projection function. This defaults to util::projection_identity
- Parameters
policy
: The execution policy to use for the scheduling of the iterations.first
: Refers to the beginning of the sequence of elements the algorithm will be applied to.last
: Refers to the end of the sequence of elements the algorithm will be applied to.dest
: Refers to the beginning of the destination range.val
: Value to be removed.proj
: Specifies the function (or function object) which will be invoked for each of the elements as a projection operation before the actual predicate is invoked.
The assignments in the parallel remove_copy algorithm invoked with an execution policy object of type parallel_policy or parallel_task_policy are permitted to execute in an unordered fashion in unspecified threads, and indeterminately sequenced within each thread.
- Return
- The remove_copy algorithm returns a hpx::future<tagged_pair<tag::in(FwdIter1), tag::out(FwdIter2)> > if the execution policy is of type sequenced_task_policy or parallel_task_policy and returns tagged_pair<tag::in(FwdIter1), tag::out(FwdIter2)> otherwise. The copy algorithm returns the pair of the input iterator forwarded to the first element after the last in the input sequence and the output iterator to the element in the destination range, one past the last element copied.
-
template<typename
ExPolicy
, typenameFwdIter1
, typenameFwdIter2
, typenameF
, typenameProj
= util::projection_identity>
util::detail::algorithm_result<ExPolicy, hpx::util::tagged_pair<tag::in(FwdIter1), tag::out(FwdIter2)>>::typeremove_copy_if
(ExPolicy &&policy, FwdIter1 first, FwdIter1 last, FwdIter2 dest, F &&f, Proj &&proj = Proj())¶ Copies the elements in the range, defined by [first, last), to another range beginning at dest. Copies only the elements for which the predicate f returns false. The order of the elements that are not removed is preserved.
Effects: Copies all the elements referred to by the iterator it in the range [first,last) for which the following corresponding conditions do not hold: INVOKE(pred, INVOKE(proj, *it)) != false.
The assignments in the parallel
remove_copy_if algorithm invoked with an execution policy object of type sequenced_policy execute in sequential order in the calling thread.- Note
- Complexity: Performs not more than last - first assignments, exactly last - first applications of the predicate f.
- Template Parameters
ExPolicy
: The type of the execution policy to use (deduced). It describes the manner in which the execution of the algorithm may be parallelized and the manner in which it executes the assignments.FwdIter1
: The type of the source iterators used (deduced). This iterator type must meet the requirements of an forward iterator.FwdIter2
: The type of the iterator representing the destination range (deduced). This iterator type must meet the requirements of an forward iterator.F
: The type of the function/function object to use (deduced). Unlike its sequential form, the parallel overload of copy_if requires F to meet the requirements of CopyConstructible.Proj
: The type of an optional projection function. This defaults to util::projection_identity
- Parameters
policy
: The execution policy to use for the scheduling of the iterations.first
: Refers to the beginning of the sequence of elements the algorithm will be applied to.last
: Refers to the end of the sequence of elements the algorithm will be applied to.dest
: Refers to the beginning of the destination range.f
: Specifies the function (or function object) which will be invoked for each of the elements in the sequence specified by [first, last).This is an unary predicate which returns true for the elements to be removed. The signature of this predicate should be equivalent to:bool pred(const Type &a);
The signature does not need to have const&, but the function must not modify the objects passed to it. The type
Type must be such that an object of type FwdIter1 can be dereferenced and then implicitly converted to Type.proj
: Specifies the function (or function object) which will be invoked for each of the elements as a projection operation before the actual predicate is invoked.
The assignments in the parallel remove_copy_if algorithm invoked with an execution policy object of type parallel_policy or parallel_task_policy are permitted to execute in an unordered fashion in unspecified threads, and indeterminately sequenced within each thread.
- Return
- The remove_copy_if algorithm returns a hpx::future<tagged_pair<tag::in(FwdIter1), tag::out(FwdIter2)> > if the execution policy is of type sequenced_task_policy or parallel_task_policy and returns tagged_pair<tag::in(FwdIter1), tag::out(FwdIter2)> otherwise. The copy algorithm returns the pair of the input iterator forwarded to the first element after the last in the input sequence and the output iterator to the element in the destination range, one past the last element copied.
-
template<typename
ExPolicy
, typenameFwdIter
, typenameT1
, typenameT2
, typenameProj
= util::projection_identity>
util::detail::algorithm_result<ExPolicy, FwdIter>::typereplace
(ExPolicy &&policy, FwdIter first, FwdIter last, T1 const &old_value, T2 const &new_value, Proj &&proj = Proj())¶ Replaces all elements satisfying specific criteria with new_value in the range [first, last).
Effects: Substitutes elements referred by the iterator it in the range [first, last) with new_value, when the following corresponding conditions hold: INVOKE(proj, *it) == old_value
The assignments in the parallel
replace algorithm invoked with an execution policy object of type sequenced_policy execute in sequential order in the calling thread.- Note
- Complexity: Performs exactly last - first assignments.
- Template Parameters
ExPolicy
: The type of the execution policy to use (deduced). It describes the manner in which the execution of the algorithm may be parallelized and the manner in which it executes the assignments.FwdIter
: The type of the source iterators used (deduced). This iterator type must meet the requirements of a forward iterator.T1
: The type of the old value to replace (deduced).T2
: The type of the new values to replace (deduced).Proj
: The type of an optional projection function. This defaults to util::projection_identity
- Parameters
policy
: The execution policy to use for the scheduling of the iterations.first
: Refers to the beginning of the sequence of elements the algorithm will be applied to.last
: Refers to the end of the sequence of elements the algorithm will be applied to.old_value
: Refers to the old value of the elements to replace.new_value
: Refers to the new value to use as the replacement.proj
: Specifies the function (or function object) which will be invoked for each of the elements as a projection operation before the actual predicate is invoked.
The assignments in the parallel replace algorithm invoked with an execution policy object of type parallel_policy or parallel_task_policy are permitted to execute in an unordered fashion in unspecified threads, and indeterminately sequenced within each thread.
- Return
- The replace algorithm returns a hpx::future<FwdIter> if the execution policy is of type sequenced_task_policy or parallel_task_policy and returns void otherwise. It returns last.
-
template<typename
ExPolicy
, typenameFwdIter
, typenameF
, typenameT
, typenameProj
= util::projection_identity>
util::detail::algorithm_result<ExPolicy, FwdIter>::typereplace_if
(ExPolicy &&policy, FwdIter first, FwdIter last, F &&f, T const &new_value, Proj &&proj = Proj())¶ Replaces all elements satisfying specific criteria (for which predicate f returns true) with new_value in the range [first, last).
Effects: Substitutes elements referred by the iterator it in the range [first, last) with new_value, when the following corresponding conditions hold: INVOKE(f, INVOKE(proj, *it)) != false
The assignments in the parallel
replace_if algorithm invoked with an execution policy object of type sequenced_policy execute in sequential order in the calling thread.- Note
- Complexity: Performs exactly last - first applications of the predicate.
- Template Parameters
ExPolicy
: The type of the execution policy to use (deduced). It describes the manner in which the execution of the algorithm may be parallelized and the manner in which it executes the assignments.FwdIter
: The type of the source iterators used (deduced). This iterator type must meet the requirements of a forward iterator.F
: The type of the function/function object to use (deduced). Unlike its sequential form, the parallel overload of equal requires F to meet the requirements of CopyConstructible. (deduced).T
: The type of the new values to replace (deduced).Proj
: The type of an optional projection function. This defaults to util::projection_identity
- Parameters
policy
: The execution policy to use for the scheduling of the iterations.first
: Refers to the beginning of the sequence of elements the algorithm will be applied to.last
: Refers to the end of the sequence of elements the algorithm will be applied to.f
: Specifies the function (or function object) which will be invoked for each of the elements in the sequence specified by [first, last).This is an unary predicate which returns true for the elements which need to replaced. The signature of this predicate should be equivalent to:bool pred(const Type &a);
The signature does not need to have const&, but the function must not modify the objects passed to it. The type
Type must be such that an object of type FwdIter can be dereferenced and then implicitly converted to Type.new_value
: Refers to the new value to use as the replacement.proj
: Specifies the function (or function object) which will be invoked for each of the elements as a projection operation before the actual predicate is invoked.
The assignments in the parallel replace_if algorithm invoked with an execution policy object of type parallel_policy or parallel_task_policy are permitted to execute in an unordered fashion in unspecified threads, and indeterminately sequenced within each thread.
- Return
- The replace_if algorithm returns a hpx::future<FwdIter> if the execution policy is of type sequenced_task_policy or parallel_task_policy and returns FwdIter otherwise. It returns last.
-
template<typename
ExPolicy
, typenameFwdIter1
, typenameFwdIter2
, typenameT1
, typenameT2
, typenameProj
= util::projection_identity>
util::detail::algorithm_result<ExPolicy, hpx::util::tagged_pair<tag::in(FwdIter1), tag::out(FwdIter2)>>::typereplace_copy
(ExPolicy &&policy, FwdIter1 first, FwdIter1 last, FwdIter2 dest, T1 const &old_value, T2 const &new_value, Proj &&proj = Proj())¶ Copies the all elements from the range [first, last) to another range beginning at dest replacing all elements satisfying a specific criteria with new_value.
Effects: Assigns to every iterator it in the range [result, result + (last - first)) either new_value or *(first + (it - result)) depending on whether the following corresponding condition holds: INVOKE(proj, *(first + (i - result))) == old_value
The assignments in the parallel
replace_copy algorithm invoked with an execution policy object of type sequenced_policy execute in sequential order in the calling thread.- Note
- Complexity: Performs exactly last - first applications of the predicate.
- Template Parameters
ExPolicy
: The type of the execution policy to use (deduced). It describes the manner in which the execution of the algorithm may be parallelized and the manner in which it executes the assignments.FwdIter1
: The type of the source iterators used (deduced). This iterator type must meet the requirements of an forward iterator.FwdIter2
: The type of the iterator representing the destination range (deduced). This iterator type must meet the requirements of an forward iterator.T1
: The type of the old value to replace (deduced).T2
: The type of the new values to replace (deduced).Proj
: The type of an optional projection function. This defaults to util::projection_identity
- Parameters
policy
: The execution policy to use for the scheduling of the iterations.first
: Refers to the beginning of the sequence of elements the algorithm will be applied to.last
: Refers to the end of the sequence of elements the algorithm will be applied to.dest
: Refers to the beginning of the destination range.old_value
: Refers to the old value of the elements to replace.new_value
: Refers to the new value to use as the replacement.proj
: Specifies the function (or function object) which will be invoked for each of the elements as a projection operation before the actual predicate is invoked.
The assignments in the parallel replace_copy algorithm invoked with an execution policy object of type parallel_policy or parallel_task_policy are permitted to execute in an unordered fashion in unspecified threads, and indeterminately sequenced within each thread.
- Return
- The replace_copy algorithm returns a hpx::future<tagged_pair<tag::in(FwdIter1), tag::out(FwdIter2)> > if the execution policy is of type sequenced_task_policy or parallel_task_policy and returns tagged_pair<tag::in(FwdIter1), tag::out(FwdIter2)> otherwise. The copy algorithm returns the pair of the input iterator last and the output iterator to the element in the destination range, one past the last element copied.
-
template<typename
ExPolicy
, typenameFwdIter1
, typenameFwdIter2
, typenameF
, typenameT
, typenameProj
= util::projection_identity>
util::detail::algorithm_result<ExPolicy, hpx::util::tagged_pair<tag::in(FwdIter1), tag::out(FwdIter2)>>::typereplace_copy_if
(ExPolicy &&policy, FwdIter1 first, FwdIter1 last, FwdIter2 dest, F &&f, T const &new_value, Proj &&proj = Proj())¶ Copies the all elements from the range [first, last) to another range beginning at dest replacing all elements satisfying a specific criteria with new_value.
Effects: Assigns to every iterator it in the range [result, result + (last - first)) either new_value or *(first + (it - result)) depending on whether the following corresponding condition holds: INVOKE(f, INVOKE(proj, *(first + (i - result)))) != false
The assignments in the parallel
replace_copy_if algorithm invoked with an execution policy object of type sequenced_policy execute in sequential order in the calling thread.- Note
- Complexity: Performs exactly last - first applications of the predicate.
- Template Parameters
ExPolicy
: The type of the execution policy to use (deduced). It describes the manner in which the execution of the algorithm may be parallelized and the manner in which it executes the assignments.FwdIter1
: The type of the source iterators used (deduced). This iterator type must meet the requirements of an forward iterator.FwdIter2
: The type of the iterator representing the destination range (deduced). This iterator type must meet the requirements of an forward iterator.F
: The type of the function/function object to use (deduced). Unlike its sequential form, the parallel overload of equal requires F to meet the requirements of CopyConstructible. (deduced).T
: The type of the new values to replace (deduced).Proj
: The type of an optional projection function. This defaults to util::projection_identity
- Parameters
policy
: The execution policy to use for the scheduling of the iterations.first
: Refers to the beginning of the sequence of elements the algorithm will be applied to.last
: Refers to the end of the sequence of elements the algorithm will be applied to.dest
: Refers to the beginning of the destination range.f
: Specifies the function (or function object) which will be invoked for each of the elements in the sequence specified by [first, last).This is an unary predicate which returns true for the elements which need to replaced. The signature of this predicate should be equivalent to:bool pred(const Type &a);
The signature does not need to have const&, but the function must not modify the objects passed to it. The type
Type must be such that an object of type FwdIter1 can be dereferenced and then implicitly converted to Type.new_value
: Refers to the new value to use as the replacement.proj
: Specifies the function (or function object) which will be invoked for each of the elements as a projection operation before the actual predicate is invoked.
The assignments in the parallel replace_copy_if algorithm invoked with an execution policy object of type parallel_policy or parallel_task_policy are permitted to execute in an unordered fashion in unspecified threads, and indeterminately sequenced within each thread.
- Return
- The replace_copy_if algorithm returns a hpx::future<tagged_pair<tag::in(FwdIter1), tag::out(FwdIter2)> > if the execution policy is of type sequenced_task_policy or parallel_task_policy and returns tagged_pair<tag::in(FwdIter1), tag::out(FwdIter2)> otherwise. The replace_copy_if algorithm returns the input iterator last and the output iterator to the element in the destination range, one past the last element copied.
-
template<typename
ExPolicy
, typenameBidirIter
>
util::detail::algorithm_result<ExPolicy, BidirIter>::typereverse
(ExPolicy &&policy, BidirIter first, BidirIter last)¶ Reverses the order of the elements in the range [first, last). Behaves as if applying std::iter_swap to every pair of iterators first+i, (last-i) - 1 for each non-negative i < (last-first)/2.
The assignments in the parallel
reverse algorithm invoked with an execution policy object of type sequenced_policy execute in sequential order in the calling thread.- Note
- Complexity: Linear in the distance between first and last.
- Template Parameters
ExPolicy
: The type of the execution policy to use (deduced). It describes the manner in which the execution of the algorithm may be parallelized and the manner in which it executes the assignments.BidirIter
: The type of the source iterators used (deduced). This iterator type must meet the requirements of an bidirectional iterator.
- Parameters
policy
: The execution policy to use for the scheduling of the iterations.first
: Refers to the beginning of the sequence of elements the algorithm will be applied to.last
: Refers to the end of the sequence of elements the algorithm will be applied to.
The assignments in the parallel reverse algorithm invoked with an execution policy object of type parallel_policy or parallel_task_policy are permitted to execute in an unordered fashion in unspecified threads, and indeterminately sequenced within each thread.
- Return
- The reverse algorithm returns a hpx::future<BidirIter> if the execution policy is of type sequenced_task_policy or parallel_task_policy and returns BidirIter otherwise. It returns last.
-
template<typename
ExPolicy
, typenameBidirIter
, typenameFwdIter
>
util::detail::algorithm_result<ExPolicy, hpx::util::tagged_pair<tag::in(BidirIter), tag::out(FwdIter)>>::typereverse_copy
(ExPolicy &&policy, BidirIter first, BidirIter last, FwdIter dest_first)¶ Copies the elements from the range [first, last) to another range beginning at dest_first in such a way that the elements in the new range are in reverse order. Behaves as if by executing the assignment *(dest_first + (last - first) - 1 - i) = *(first + i) once for each non-negative i < (last - first) If the source and destination ranges (that is, [first, last) and [dest_first, dest_first+(last-first)) respectively) overlap, the behavior is undefined.
The assignments in the parallel
reverse_copy algorithm invoked with an execution policy object of type sequenced_policy execute in sequential order in the calling thread.- Note
- Complexity: Performs exactly last - first assignments.
- Template Parameters
ExPolicy
: The type of the execution policy to use (deduced). It describes the manner in which the execution of the algorithm may be parallelized and the manner in which it executes the assignments.BidirIter
: The type of the source iterators used (deduced). This iterator type must meet the requirements of an bidirectional iterator.FwdIter
: The type of the iterator representing the destination range (deduced). This iterator type must meet the requirements of an forward iterator.
- Parameters
policy
: The execution policy to use for the scheduling of the iterations.first
: Refers to the beginning of the sequence of elements the algorithm will be applied to.last
: Refers to the end of the sequence of elements the algorithm will be applied to.dest_first
: Refers to the begin of the destination range.
The assignments in the parallel reverse_copy algorithm invoked with an execution policy object of type parallel_policy or parallel_task_policy are permitted to execute in an unordered fashion in unspecified threads, and indeterminately sequenced within each thread.
- Return
- The reverse_copy algorithm returns a hpx::future<tagged_pair<tag::in(BidirIter), tag::out(FwdIter)> > if the execution policy is of type sequenced_task_policy or parallel_task_policy and returns tagged_pair<tag::in(BidirIter), tag::out(FwdIter)> otherwise. The copy algorithm returns the pair of the input iterator forwarded to the first element after the last in the input sequence and the output iterator to the element in the destination range, one past the last element copied.
-
template<typename
ExPolicy
, typenameFwdIter
>
util::detail::algorithm_result<ExPolicy, hpx::util::tagged_pair<tag::begin(FwdIter), tag::end(FwdIter)>>::typerotate
(ExPolicy &&policy, FwdIter first, FwdIter new_first, FwdIter last)¶ Performs a left rotation on a range of elements. Specifically, rotate swaps the elements in the range [first, last) in such a way that the element new_first becomes the first element of the new range and new_first - 1 becomes the last element.
The assignments in the parallel
rotate algorithm invoked with an execution policy object of type sequenced_policy execute in sequential order in the calling thread.- Note
- Complexity: Linear in the distance between first and last.
- Template Parameters
ExPolicy
: The type of the execution policy to use (deduced). It describes the manner in which the execution of the algorithm may be parallelized and the manner in which it executes the assignments.FwdIter
: The type of the source iterators used (deduced). This iterator type must meet the requirements of an forward iterator.
- Parameters
policy
: The execution policy to use for the scheduling of the iterations.first
: Refers to the beginning of the sequence of elements the algorithm will be applied to.new_first
: Refers to the element that should appear at the beginning of the rotated range.last
: Refers to the end of the sequence of elements the algorithm will be applied to.
The assignments in the parallel rotate algorithm invoked with an execution policy object of type parallel_policy or parallel_task_policy are permitted to execute in an unordered fashion in unspecified threads, and indeterminately sequenced within each thread.
- Note
- The type of dereferenced FwdIter must meet the requirements of MoveAssignable and MoveConstructible.
- Return
- The rotate algorithm returns a hpx::future<tagged_pair<tag::begin(FwdIter), tag::end(FwdIter)> > if the execution policy is of type parallel_task_policy and returns tagged_pair<tag::begin(FwdIter), tag::end(FwdIter)> otherwise. The rotate algorithm returns the iterator equal to pair(first + (last - new_first), last).
-
template<typename
ExPolicy
, typenameFwdIter1
, typenameFwdIter2
>
util::detail::algorithm_result<ExPolicy, hpx::util::tagged_pair<tag::in(FwdIter1), tag::out(FwdIter2)>>::typerotate_copy
(ExPolicy &&policy, FwdIter1 first, FwdIter1 new_first, FwdIter1 last, FwdIter2 dest_first)¶ Copies the elements from the range [first, last), to another range beginning at dest_first in such a way, that the element new_first becomes the first element of the new range and new_first - 1 becomes the last element.
The assignments in the parallel
rotate_copy algorithm invoked with an execution policy object of type sequenced_policy execute in sequential order in the calling thread.- Note
- Complexity: Performs exactly last - first assignments.
- Template Parameters
ExPolicy
: The type of the execution policy to use (deduced). It describes the manner in which the execution of the algorithm may be parallelized and the manner in which it executes the assignments.FwdIter1
: The type of the source iterators used (deduced). This iterator type must meet the requirements of an bidirectional iterator.FwdIter2
: The type of the iterator representing the destination range (deduced). This iterator type must meet the requirements of an forward iterator.
- Parameters
policy
: The execution policy to use for the scheduling of the iterations.first
: Refers to the beginning of the sequence of elements the algorithm will be applied to.new_first
: Refers to the element that should appear at the beginning of the rotated range.last
: Refers to the end of the sequence of elements the algorithm will be applied to.dest_first
: Refers to the begin of the destination range.
The assignments in the parallel rotate_copy algorithm invoked with an execution policy object of type parallel_policy or parallel_task_policy are permitted to execute in an unordered fashion in unspecified threads, and indeterminately sequenced within each thread.
- Return
- The rotate_copy algorithm returns a hpx::future<tagged_pair<tag::in(FwdIter1), tag::out(FwdIter2)> > if the execution policy is of type parallel_task_policy and returns tagged_pair<tag::in(FwdIter1), tag::out(FwdIter2)> otherwise. The rotate_copy algorithm returns the output iterator to the element past the last element copied.
-
template<typename
ExPolicy
, typenameFwdIter
, typenameFwdIter2
, typenamePred
= detail::equal_to, typenameProj1
= util::projection_identity, typenameProj2
= util::projection_identity>
util::detail::algorithm_result<ExPolicy, FwdIter>::typesearch
(ExPolicy &&policy, FwdIter first, FwdIter last, FwdIter2 s_first, FwdIter2 s_last, Pred &&op = Pred(), Proj1 &&proj1 = Proj1(), Proj2 &&proj2 = Proj2())¶ Searches the range [first, last) for any elements in the range [s_first, s_last). Uses a provided predicate to compare elements.
The comparison operations in the parallel
search algorithm invoked with an execution policy object of type sequenced_policy execute in sequential order in the calling thread.- Note
- Complexity: at most (S*N) comparisons where S = distance(s_first, s_last) and N = distance(first, last).
- Template Parameters
ExPolicy
: The type of the execution policy to use (deduced). It describes the manner in which the execution of the algorithm may be parallelized and the manner in which it executes the assignments.FwdIter
: The type of the source iterators used for the first range (deduced). This iterator type must meet the requirements of an input iterator.FwdIter2
: The type of the source iterators used for the second range (deduced). This iterator type must meet the requirements of an forward iterator.Pred
: The type of an optional function/function object to use. Unlike its sequential form, the parallel overload of adjacent_find requires Pred to meet the requirements of CopyConstructible. This defaults to std::equal_to<>Proj1
: The type of an optional projection function. This defaults to util::projection_identity and is applied to the elements of type dereferenced FwdIter.Proj2
: The type of an optional projection function. This defaults to util::projection_identity and is applied to the elements of type dereferenced FwdIter2.
- Parameters
policy
: The execution policy to use for the scheduling of the iterations.first
: Refers to the beginning of the sequence of elements of the first range the algorithm will be applied to.last
: Refers to the end of the sequence of elements of the first range the algorithm will be applied to.s_first
: Refers to the beginning of the sequence of elements the algorithm will be searching for.s_last
: Refers to the end of the sequence of elements of the algorithm will be searching for.op
: Refers to the binary predicate which returns true if the elements should be treated as equal. the signature of the function should be equivalent tobool pred(const Type1 &a, const Type2 &b);
The signature does not need to have const &, but the function must not modify the objects passed to it. The types
Type1 and Type2 must be such that objects of types FwdIter1 and FwdIter2 can be dereferenced and then implicitly converted to Type1 and Type2 respectivelyproj1
: Specifies the function (or function object) which will be invoked for each of the elements of type dereferenced FwdIter1 as a projection operation before the actual predicate is invoked.proj2
: Specifies the function (or function object) which will be invoked for each of the elements of type dereferenced FwdIter2 as a projection operation before the actual predicate is invoked.
The comparison operations in the parallel search algorithm invoked with an execution policy object of type parallel_policy or parallel_task_policy are permitted to execute in an unordered fashion in unspecified threads, and indeterminately sequenced within each thread.
- Return
- The search algorithm returns a hpx::future<FwdIter> if the execution policy is of type task_execution_policy and returns FwdIter otherwise. The search algorithm returns an iterator to the beginning of the first subsequence [s_first, s_last) in range [first, last). If the length of the subsequence [s_first, s_last) is greater than the length of the range [first, last), last is returned. Additionally if the size of the subsequence is empty first is returned. If no subsequence is found, last is returned.
-
template<typename
ExPolicy
, typenameFwdIter
, typenameFwdIter2
, typenamePred
= detail::equal_to, typenameProj1
= util::projection_identity, typenameProj2
= util::projection_identity>
util::detail::algorithm_result<ExPolicy, FwdIter>::typesearch_n
(ExPolicy &&policy, FwdIter first, std::size_t count, FwdIter2 s_first, FwdIter2 s_last, Pred &&op = Pred(), Proj1 &&proj1 = Proj1(), Proj2 &&proj2 = Proj2())¶ Searches the range [first, last) for any elements in the range [s_first, s_last). Uses a provided predicate to compare elements.
The comparison operations in the parallel
search_n algorithm invoked with an execution policy object of type sequenced_policy execute in sequential order in the calling thread.- Note
- Complexity: at most (S*N) comparisons where S = distance(s_first, s_last) and N = count.
- Template Parameters
ExPolicy
: The type of the execution policy to use (deduced). It describes the manner in which the execution of the algorithm may be parallelized and the manner in which it executes the assignments.FwdIter
: The type of the source iterators used for the first range (deduced). This iterator type must meet the requirements of an input iterator.FwdIter2
: The type of the source iterators used for the second range (deduced). This iterator type must meet the requirements of an forward iterator.Pred
: The type of an optional function/function object to use. Unlike its sequential form, the parallel overload of adjacent_find requires Pred to meet the requirements of CopyConstructible. This defaults to std::equal_to<>
- Parameters
policy
: The execution policy to use for the scheduling of the iterations.first
: Refers to the beginning of the sequence of elements of the first range the algorithm will be applied to.count
: Refers to the range of elements of the first range the algorithm will be applied to.s_first
: Refers to the beginning of the sequence of elements the algorithm will be searching for.s_last
: Refers to the end of the sequence of elements of the algorithm will be searching for.op
: Refers to the binary predicate which returns true if the elements should be treated as equal. the signature of the function should be equivalent tobool pred(const Type1 &a, const Type2 &b);
The signature does not need to have const &, but the function must not modify the objects passed to it. The types
Type1 and Type2 must be such that objects of types FwdIter1 and FwdIter2 can be dereferenced and then implicitly converted to Type1 and Type2 respectivelyproj1
: Specifies the function (or function object) which will be invoked for each of the elements of type dereferenced FwdIter1 as a projection operation before the actual predicate is invoked.proj2
: Specifies the function (or function object) which will be invoked for each of the elements of type dereferenced FwdIter2 as a projection operation before the actual predicate is invoked.
The comparison operations in the parallel search_n algorithm invoked with an execution policy object of type parallel_policy or parallel_task_policy are permitted to execute in an unordered fashion in unspecified threads, and indeterminately sequenced within each thread.
- Return
- The search_n algorithm returns a hpx::future<FwdIter> if the execution policy is of type task_execution_policy and returns FwdIter otherwise. The search_n algorithm returns an iterator to the beginning of the last subsequence [s_first, s_last) in range [first, first+count). If the length of the subsequence [s_first, s_last) is greater than the length of the range [first, first+count), first is returned. Additionally if the size of the subsequence is empty or no subsequence is found, first is also returned.
-
template<typename
ExPolicy
, typenameFwdIter1
, typenameFwdIter2
, typenameFwdIter3
, typenamePred
= detail::less>
std::enable_if<execution::is_execution_policy<ExPolicy>::value, typename util::detail::algorithm_result<ExPolicy, FwdIter3>::type>::typeset_difference
(ExPolicy &&policy, FwdIter1 first1, FwdIter1 last1, FwdIter2 first2, FwdIter2 last2, FwdIter3 dest, Pred &&op = Pred())¶ Constructs a sorted range beginning at dest consisting of all elements present in the range [first1, last1) and not present in the range [first2, last2). This algorithm expects both input ranges to be sorted with the given binary predicate f.
Equivalent elements are treated individually, that is, if some element is found
m times in [first1, last1) and n times in [first2, last2), it will be copied to dest exactly std::max(m-n, 0) times. The resulting range cannot overlap with either of the input ranges.- Note
- Complexity: At most 2*(N1 + N2 - 1) comparisons, where N1 is the length of the first sequence and N2 is the length of the second sequence.
The resulting range cannot overlap with either of the input ranges.
The application of function objects in parallel algorithm invoked with a sequential execution policy object execute in sequential order in the calling thread (
sequenced_policy) or in a single new thread spawned from the current thread (for sequenced_task_policy).- Template Parameters
ExPolicy
: The type of the execution policy to use (deduced). It describes the manner in which the execution of the algorithm may be parallelized and the manner in which it applies user-provided function objects.FwdIter1
: The type of the source iterators used (deduced) representing the first sequence. This iterator type must meet the requirements of an forward iterator.FwdIter2
: The type of the source iterators used (deduced) representing the first sequence. This iterator type must meet the requirements of an forward iterator.FwdIter3
: The type of the iterator representing the destination range (deduced). This iterator type must meet the requirements of an output iterator.Pred
: The type of an optional function/function object to use. Unlike its sequential form, the parallel overload of set_difference requires Pred to meet the requirements of CopyConstructible. This defaults to std::less<>
- Parameters
policy
: The execution policy to use for the scheduling of the iterations.first1
: Refers to the beginning of the sequence of elements of the first range the algorithm will be applied to.last1
: Refers to the end of the sequence of elements of the first range the algorithm will be applied to.first2
: Refers to the beginning of the sequence of elements of the second range the algorithm will be applied to.last2
: Refers to the end of the sequence of elements of the second range the algorithm will be applied to.dest
: Refers to the beginning of the destination range.op
: The binary predicate which returns true if the elements should be treated as equal. The signature of the predicate function should be equivalent to the following:bool pred(const Type1 &a, const Type1 &b);
The signature does not need to have const &, but the function must not modify the objects passed to it. The type
Type1 must be such that objects of type InIter can be dereferenced and then implicitly converted to Type1
The application of function objects in parallel algorithm invoked with an execution policy object of type parallel_policy or parallel_task_policy are permitted to execute in an unordered fashion in unspecified threads, and indeterminately sequenced within each thread.
- Return
- The set_difference algorithm returns a hpx::future<FwdIter3> if the execution policy is of type sequenced_task_policy or parallel_task_policy and returns FwdIter3 otherwise. The set_difference algorithm returns the output iterator to the element in the destination range, one past the last element copied.
-
template<typename
ExPolicy
, typenameFwdIter1
, typenameFwdIter2
, typenameFwdIter3
, typenamePred
= detail::less>
std::enable_if<execution::is_execution_policy<ExPolicy>::value, typename util::detail::algorithm_result<ExPolicy, FwdIter3>::type>::typeset_intersection
(ExPolicy &&policy, FwdIter1 first1, FwdIter1 last1, FwdIter2 first2, FwdIter2 last2, FwdIter3 dest, Pred &&op = Pred())¶ Constructs a sorted range beginning at dest consisting of all elements present in both sorted ranges [first1, last1) and [first2, last2). This algorithm expects both input ranges to be sorted with the given binary predicate f.
If some element is found
m times in [first1, last1) and n times in [first2, last2), the first std::min(m, n) elements will be copied from the first range to the destination range. The order of equivalent elements is preserved. The resulting range cannot overlap with either of the input ranges.- Note
- Complexity: At most 2*(N1 + N2 - 1) comparisons, where N1 is the length of the first sequence and N2 is the length of the second sequence.
The resulting range cannot overlap with either of the input ranges.
The application of function objects in parallel algorithm invoked with a sequential execution policy object execute in sequential order in the calling thread (
sequenced_policy) or in a single new thread spawned from the current thread (for sequenced_task_policy).- Template Parameters
ExPolicy
: The type of the execution policy to use (deduced). It describes the manner in which the execution of the algorithm may be parallelized and the manner in which it applies user-provided function objects.FwdIter1
: The type of the source iterators used (deduced) representing the first sequence. This iterator type must meet the requirements of an forward iterator.FwdIter2
: The type of the source iterators used (deduced) representing the first sequence. This iterator type must meet the requirements of an forward iterator.FwdIter3
: The type of the iterator representing the destination range (deduced). This iterator type must meet the requirements of an output iterator.Pred
: The type of an optional function/function object to use. Unlike its sequential form, the parallel overload of set_intersection requires Pred to meet the requirements of CopyConstructible. This defaults to std::less<>
- Parameters
policy
: The execution policy to use for the scheduling of the iterations.first1
: Refers to the beginning of the sequence of elements of the first range the algorithm will be applied to.last1
: Refers to the end of the sequence of elements of the first range the algorithm will be applied to.first2
: Refers to the beginning of the sequence of elements of the second range the algorithm will be applied to.last2
: Refers to the end of the sequence of elements of the second range the algorithm will be applied to.dest
: Refers to the beginning of the destination range.op
: The binary predicate which returns true if the elements should be treated as equal. The signature of the predicate function should be equivalent to the following:bool pred(const Type1 &a, const Type1 &b);
The signature does not need to have const &, but the function must not modify the objects passed to it. The type
Type1 must be such that objects of type InIter can be dereferenced and then implicitly converted to Type1
The application of function objects in parallel algorithm invoked with an execution policy object of type parallel_policy or parallel_task_policy are permitted to execute in an unordered fashion in unspecified threads, and indeterminately sequenced within each thread.
- Return
- The set_intersection algorithm returns a hpx::future<FwdIter3> if the execution policy is of type sequenced_task_policy or parallel_task_policy and returns FwdIter3 otherwise. The set_intersection algorithm returns the output iterator to the element in the destination range, one past the last element copied.
-
template<typename
ExPolicy
, typenameFwdIter1
, typenameFwdIter2
, typenameFwdIter3
, typenamePred
= detail::less>
std::enable_if<execution::is_execution_policy<ExPolicy>::value, typename util::detail::algorithm_result<ExPolicy, FwdIter3>::type>::typeset_symmetric_difference
(ExPolicy &&policy, FwdIter1 first1, FwdIter1 last1, FwdIter2 first2, FwdIter2 last2, FwdIter3 dest, Pred &&op = Pred())¶ Constructs a sorted range beginning at dest consisting of all elements present in either of the sorted ranges [first1, last1) and [first2, last2), but not in both of them are copied to the range beginning at dest. The resulting range is also sorted. This algorithm expects both input ranges to be sorted with the given binary predicate f.
If some element is found
m times in [first1, last1) and n times in [first2, last2), it will be copied to dest exactly std::abs(m-n) times. If m>n, then the last m-n of those elements are copied from [first1,last1), otherwise the last n-m elements are copied from [first2,last2). The resulting range cannot overlap with either of the input ranges.- Note
- Complexity: At most 2*(N1 + N2 - 1) comparisons, where N1 is the length of the first sequence and N2 is the length of the second sequence.
The resulting range cannot overlap with either of the input ranges.
The application of function objects in parallel algorithm invoked with a sequential execution policy object execute in sequential order in the calling thread (
sequenced_policy) or in a single new thread spawned from the current thread (for sequenced_task_policy).- Template Parameters
ExPolicy
: The type of the execution policy to use (deduced). It describes the manner in which the execution of the algorithm may be parallelized and the manner in which it applies user-provided function objects.FwdIter1
: The type of the source iterators used (deduced) representing the first sequence. This iterator type must meet the requirements of an forward iterator.FwdIter2
: The type of the source iterators used (deduced) representing the first sequence. This iterator type must meet the requirements of an forward iterator.FwdIter3
: The type of the iterator representing the destination range (deduced). This iterator type must meet the requirements of an output iterator.Pred
: The type of an optional function/function object to use. Unlike its sequential form, the parallel overload of set_symmetric_difference requires Pred to meet the requirements of CopyConstructible. This defaults to std::less<>
- Parameters
policy
: The execution policy to use for the scheduling of the iterations.first1
: Refers to the beginning of the sequence of elements of the first range the algorithm will be applied to.last1
: Refers to the end of the sequence of elements of the first range the algorithm will be applied to.first2
: Refers to the beginning of the sequence of elements of the second range the algorithm will be applied to.last2
: Refers to the end of the sequence of elements of the second range the algorithm will be applied to.dest
: Refers to the beginning of the destination range.op
: The binary predicate which returns true if the elements should be treated as equal. The signature of the predicate function should be equivalent to the following:bool pred(const Type1 &a, const Type1 &b);
The signature does not need to have const &, but the function must not modify the objects passed to it. The type
Type1 must be such that objects of type InIter can be dereferenced and then implicitly converted to Type1
The application of function objects in parallel algorithm invoked with an execution policy object of type parallel_policy or parallel_task_policy are permitted to execute in an unordered fashion in unspecified threads, and indeterminately sequenced within each thread.
- Return
- The set_symmetric_difference algorithm returns a hpx::future<FwdIter3> if the execution policy is of type sequenced_task_policy or parallel_task_policy and returns FwdIter3 otherwise. The set_symmetric_difference algorithm returns the output iterator to the element in the destination range, one past the last element copied.
-
template<typename
ExPolicy
, typenameFwdIter1
, typenameFwdIter2
, typenameFwdIter3
, typenamePred
= detail::less>
std::enable_if<execution::is_execution_policy<ExPolicy>::value, typename util::detail::algorithm_result<ExPolicy, FwdIter3>::type>::typeset_union
(ExPolicy &&policy, FwdIter1 first1, FwdIter1 last1, FwdIter2 first2, FwdIter2 last2, FwdIter3 dest, Pred &&op = Pred())¶ Constructs a sorted range beginning at dest consisting of all elements present in one or both sorted ranges [first1, last1) and [first2, last2). This algorithm expects both input ranges to be sorted with the given binary predicate f.
If some element is found
m times in [first1, last1) and n times in [first2, last2), then all m elements will be copied from [first1, last1) to dest, preserving order, and then exactly std::max(n-m, 0) elements will be copied from [first2, last2) to dest, also preserving order.- Note
- Complexity: At most 2*(N1 + N2 - 1) comparisons, where N1 is the length of the first sequence and N2 is the length of the second sequence.
The resulting range cannot overlap with either of the input ranges.
The application of function objects in parallel algorithm invoked with a sequential execution policy object execute in sequential order in the calling thread (
sequenced_policy) or in a single new thread spawned from the current thread (for sequenced_task_policy).- Template Parameters
ExPolicy
: The type of the execution policy to use (deduced). It describes the manner in which the execution of the algorithm may be parallelized and the manner in which it applies user-provided function objects.FwdIter1
: The type of the source iterators used (deduced) representing the first sequence. This iterator type must meet the requirements of an forward iterator.FwdIter2
: The type of the source iterators used (deduced) representing the first sequence. This iterator type must meet the requirements of an forward iterator.FwdIter3
: The type of the iterator representing the destination range (deduced). This iterator type must meet the requirements of an output iterator.Op
: The type of an optional function/function object to use. Unlike its sequential form, the parallel overload of set_union requires Pred to meet the requirements of CopyConstructible. This defaults to std::less<>
- Parameters
policy
: The execution policy to use for the scheduling of the iterations.first1
: Refers to the beginning of the sequence of elements of the first range the algorithm will be applied to.last1
: Refers to the end of the sequence of elements of the first range the algorithm will be applied to.first2
: Refers to the beginning of the sequence of elements of the second range the algorithm will be applied to.last2
: Refers to the end of the sequence of elements of the second range the algorithm will be applied to.dest
: Refers to the beginning of the destination range.op
: The binary predicate which returns true if the elements should be treated as equal. The signature of the predicate function should be equivalent to the following:bool pred(const Type1 &a, const Type1 &b);
The signature does not need to have const &, but the function must not modify the objects passed to it. The type
Type1 must be such that objects of type InIter can be dereferenced and then implicitly converted to Type1
The application of function objects in parallel algorithm invoked with an execution policy object of type parallel_policy or parallel_task_policy are permitted to execute in an unordered fashion in unspecified threads, and indeterminately sequenced within each thread.
- Return
- The set_union algorithm returns a hpx::future<FwdIter3> if the execution policy is of type sequenced_task_policy or parallel_task_policy and returns FwdIter3 otherwise. The set_union algorithm returns the output iterator to the element in the destination range, one past the last element copied.
-
template<typename
ExPolicy
, typenameRandomIt
, typenameProj
= util::projection_identity, typenameCompare
= detail::less>
util::detail::algorithm_result<ExPolicy, RandomIt>::typesort
(ExPolicy &&policy, RandomIt first, RandomIt last, Compare &&comp = Compare(), Proj &&proj = Proj())¶ Sorts the elements in the range [first, last) in ascending order. The order of equal elements is not guaranteed to be preserved. The function uses the given comparison function object comp (defaults to using operator<()).
A sequence is sorted with respect to a comparator
comp and a projection proj if for every iterator i pointing to the sequence and every non-negative integer n such that i + n is a valid iterator pointing to an element of the sequence, and INVOKE(comp, INVOKE(proj, *(i + n)), INVOKE(proj, *i)) == false.- Note
- Complexity: O(Nlog(N)), where N = std::distance(first, last) comparisons.
comp has to induce a strict weak ordering on the values.
- Template Parameters
ExPolicy
: The type of the execution policy to use (deduced). It describes the manner in which the execution of the algorithm may be parallelized and the manner in which it applies user-provided function objects.Iter
: The type of the source iterators used (deduced). This iterator type must meet the requirements of a random access iterator.Comp
: The type of the function/function object to use (deduced).Proj
: The type of an optional projection function. This defaults to util::projection_identity
- Parameters
policy
: The execution policy to use for the scheduling of the iterations.first
: Refers to the beginning of the sequence of elements the algorithm will be applied to.last
: Refers to the end of the sequence of elements the algorithm will be applied to.comp
: comp is a callable object. The return value of the INVOKE operation applied to an object of type Comp, when contextually converted to bool, yields true if the first argument of the call is less than the second, and false otherwise. It is assumed that comp will not apply any non-constant function through the dereferenced iterator.proj
: Specifies the function (or function object) which will be invoked for each pair of elements as a projection operation before the actual predicate comp is invoked.
The application of function objects in parallel algorithm invoked with an execution policy object of type sequenced_policy execute in sequential order in the calling thread.
The application of function objects in parallel algorithm invoked with an execution policy object of type parallel_policy or parallel_task_policy are permitted to execute in an unordered fashion in unspecified threads, and indeterminately sequenced within each thread.
- Return
- The sort algorithm returns a hpx::future<RandomIt> if the execution policy is of type sequenced_task_policy or parallel_task_policy and returns RandomIt otherwise. The algorithm returns an iterator pointing to the first element after the last element in the input sequence.
-
template<typename
ExPolicy
, typenameKeyIter
, typenameValueIter
, typenameCompare
= detail::less>
util::detail::algorithm_result<ExPolicy, hpx::util::tagged_pair<tag::in1(KeyIter), tag::in2(ValueIter)>>::typesort_by_key
(ExPolicy &&policy, KeyIter key_first, KeyIter key_last, ValueIter value_first, Compare &&comp = Compare())¶ Sorts one range of data using keys supplied in another range. The key elements in the range [key_first, key_last) are sorted in ascending order with the corresponding elements in the value range moved to follow the sorted order. The algorithm is not stable, the order of equal elements is not guaranteed to be preserved. The function uses the given comparison function object comp (defaults to using operator<()).
A sequence is sorted with respect to a comparator
comp and a projection proj if for every iterator i pointing to the sequence and every non-negative integer n such that i + n is a valid iterator pointing to an element of the sequence, and INVOKE(comp, INVOKE(proj, *(i + n)), INVOKE(proj, *i)) == false.- Note
- Complexity: O(Nlog(N)), where N = std::distance(first, last) comparisons.
comp has to induce a strict weak ordering on the values.
- Template Parameters
ExPolicy
: The type of the execution policy to use (deduced). It describes the manner in which the execution of the algorithm may be parallelized and the manner in which it applies user-provided function objects.KeyIter
: The type of the key iterators used (deduced). This iterator type must meet the requirements of a random access iterator.ValueIter
: The type of the value iterators used (deduced). This iterator type must meet the requirements of a random access iterator.Comp
: The type of the function/function object to use (deduced).
- Parameters
policy
: The execution policy to use for the scheduling of the iterations.key_first
: Refers to the beginning of the sequence of key elements the algorithm will be applied to.key_last
: Refers to the end of the sequence of key elements the algorithm will be applied to.value_first
: Refers to the beginning of the sequence of value elements the algorithm will be applied to, the range of elements must match [key_first, key_last)comp
: comp is a callable object. The return value of the INVOKE operation applied to an object of type Comp, when contextually converted to bool, yields true if the first argument of the call is less than the second, and false otherwise. It is assumed that comp will not apply any non-constant function through the dereferenced iterator.
The application of function objects in parallel algorithm invoked with an execution policy object of type sequenced_policy execute in sequential order in the calling thread.
The application of function objects in parallel algorithm invoked with an execution policy object of type parallel_policy or parallel_task_policy are permitted to execute in an unordered fashion in unspecified threads, and indeterminately sequenced within each thread.
- Return
- The sort_by-key algorithm returns a hpx::future<tagged_pair<tag::in1(KeyIter>, tag::in2(ValueIter)> > if the execution policy is of type sequenced_task_policy or parallel_task_policy and returns otherwise. The algorithm returns a pair holding an iterator pointing to the first element after the last element in the input key sequence and an iterator pointing to the first element after the last element in the input value sequence.
-
template<typename
ExPolicy
, typenameFwdIter1
, typenameFwdIter2
>
std::enable_if<execution::is_execution_policy<ExPolicy>::value, typename util::detail::algorithm_result<ExPolicy, FwdIter2>::type>::typeswap_ranges
(ExPolicy &&policy, FwdIter1 first1, FwdIter1 last1, FwdIter2 first2)¶ Exchanges elements between range [first1, last1) and another range starting at first2.
The swap operations in the parallel
swap_ranges algorithm invoked with an execution policy object of type sequenced_policy execute in sequential order in the calling thread.- Note
- Complexity: Linear in the distance between first1 and last1
- Template Parameters
ExPolicy
: The type of the execution policy to use (deduced). It describes the manner in which the execution of the algorithm may be parallelized and the manner in which it executes the swap operations.FwdIter1
: The type of the first range of iterators to swap (deduced). This iterator type must meet the requirements of an forward iterator.FwdIter2
: The type of the second range of iterators to swap (deduced). This iterator type must meet the requirements of an forward iterator.
- Parameters
policy
: The execution policy to use for the scheduling of the iterations.first1
: Refers to the beginning of the first sequence of elements the algorithm will be applied to.last1
: Refers to the end of the first sequence of elements the algorithm will be applied to.first2
: Refers to the beginning of the second sequence of elements the algorithm will be applied to.
The swap operations in the parallel swap_ranges algorithm invoked with an execution policy object of type parallel_policy or parallel_task_policy are permitted to execute in an unordered fashion in unspecified threads, and indeterminately sequenced within each thread.
- Return
- The swap_ranges algorithm returns a hpx::future<FwdIter2> if the execution policy is of type parallel_task_policy and returns FwdIter2 otherwise. The swap_ranges algorithm returns iterator to the element past the last element exchanged in the range beginning with first2.
-
template<typename
ExPolicy
, typenameFwdIter1
, typenameFwdIter2
, typenameF
, typenameProj
= util::projection_identity>
util::detail::algorithm_result<ExPolicy, hpx::util::tagged_pair<tag::in(FwdIter1), tag::out(FwdIter2)>>::typetransform
(ExPolicy &&policy, FwdIter1 first, FwdIter1 last, FwdIter2 dest, F &&f, Proj &&proj = Proj())¶ Applies the given function f to the range [first, last) and stores the result in another range, beginning at dest.
The invocations of
f in the parallel transform algorithm invoked with an execution policy object of type sequenced_policy execute in sequential order in the calling thread.- Note
- Complexity: Exactly last - first applications of f
- Template Parameters
ExPolicy
: The type of the execution policy to use (deduced). It describes the manner in which the execution of the algorithm may be parallelized and the manner in which it executes the invocations of f.FwdIter1
: The type of the source iterators used (deduced). This iterator type must meet the requirements of an forward iterator.FwdIter2
: The type of the iterator representing the destination range (deduced). This iterator type must meet the requirements of an forward iterator.F
: The type of the function/function object to use (deduced). Unlike its sequential form, the parallel overload of transform requires F to meet the requirements of CopyConstructible.Proj
: The type of an optional projection function. This defaults to util::projection_identity
- Parameters
policy
: The execution policy to use for the scheduling of the iterations.first
: Refers to the beginning of the sequence of elements the algorithm will be applied to.last
: Refers to the end of the sequence of elements the algorithm will be applied to.dest
: Refers to the beginning of the destination range.f
: Specifies the function (or function object) which will be invoked for each of the elements in the sequence specified by [first, last).This is an unary predicate. The signature of this predicate should be equivalent to:Ret fun(const Type &a);
The signature does not need to have const&. The type
Type must be such that an object of type FwdIter can be dereferenced and then implicitly converted to Type. The type Ret must be such that an object of type FwdIter2 can be dereferenced and assigned a value of type Ret.proj
: Specifies the function (or function object) which will be invoked for each of the elements as a projection operation before the actual predicate f is invoked.
The invocations of f in the parallel transform algorithm invoked with an execution policy object of type parallel_policy or parallel_task_policy are permitted to execute in an unordered fashion in unspecified threads, and indeterminately sequenced within each thread.
- Return
- The transform algorithm returns a hpx::future<tagged_pair<tag::in(FwdIter1), tag::out(FwdIter2)> > if the execution policy is of type parallel_task_policy and returns tagged_pair<tag::in(FwdIter1), tag::out(FwdIter2)> otherwise. The transform algorithm returns a tuple holding an iterator referring to the first element after the input sequence and the output iterator to the element in the destination range, one past the last element copied.
-
template<typename
ExPolicy
, typenameFwdIter1
, typenameFwdIter2
, typenameFwdIter3
, typenameF
, typenameProj1
= util::projection_identity, typenameProj2
= util::projection_identity>
util::detail::algorithm_result<ExPolicy, hpx::util::tagged_tuple<tag::in1(FwdIter1), tag::in2(FwdIter2), tag::out(FwdIter3)>>::typetransform
(ExPolicy &&policy, FwdIter1 first1, FwdIter1 last1, FwdIter2 first2, FwdIter3 dest, F &&f, Proj1 &&proj1 = Proj1(), Proj2 &&proj2 = Proj2())¶ Applies the given function f to pairs of elements from two ranges: one defined by [first1, last1) and the other beginning at first2, and stores the result in another range, beginning at dest.
The invocations of
f in the parallel transform algorithm invoked with an execution policy object of type sequenced_policy execute in sequential order in the calling thread.- Note
- Complexity: Exactly last - first applications of f
- Template Parameters
ExPolicy
: The type of the execution policy to use (deduced). It describes the manner in which the execution of the algorithm may be parallelized and the manner in which it executes the invocations of f.FwdIter1
: The type of the source iterators for the first range used (deduced). This iterator type must meet the requirements of an forward iterator.FwdIter2
: The type of the source iterators for the second range used (deduced). This iterator type must meet the requirements of an forward iterator.FwdIter3
: The type of the iterator representing the destination range (deduced). This iterator type must meet the requirements of an forward iterator.F
: The type of the function/function object to use (deduced). Unlike its sequential form, the parallel overload of transform requires F to meet the requirements of CopyConstructible.Proj1
: The type of an optional projection function to be used for elements of the first sequence. This defaults to util::projection_identityProj2
: The type of an optional projection function to be used for elements of the second sequence. This defaults to util::projection_identity
- Parameters
policy
: The execution policy to use for the scheduling of the iterations.first1
: Refers to the beginning of the first sequence of elements the algorithm will be applied to.last1
: Refers to the end of the first sequence of elements the algorithm will be applied to.first2
: Refers to the beginning of the second sequence of elements the algorithm will be applied to.dest
: Refers to the beginning of the destination range.f
: Specifies the function (or function object) which will be invoked for each of the elements in the sequence specified by [first, last).This is a binary predicate. The signature of this predicate should be equivalent to:Ret fun(const Type1 &a, const Type2 &b);
The signature does not need to have const&. The types
Type1 and Type2 must be such that objects of types FwdIter1 and FwdIter2 can be dereferenced and then implicitly converted to Type1 and Type2 respectively. The type Ret must be such that an object of type FwdIter3 can be dereferenced and assigned a value of type Ret.proj1
: Specifies the function (or function object) which will be invoked for each of the elements of the first sequence as a projection operation before the actual predicate f is invoked.proj2
: Specifies the function (or function object) which will be invoked for each of the elements of the second sequence as a projection operation before the actual predicate f is invoked.
The invocations of f in the parallel transform algorithm invoked with an execution policy object of type parallel_policy or parallel_task_policy are permitted to execute in an unordered fashion in unspecified threads, and indeterminately sequenced within each thread.
- Return
- The transform algorithm returns a hpx::future<tagged_tuple<tag::in1(FwdIter1), tag::in2(FwdIter2), tag::out(FwdIter3)> > if the execution policy is of type parallel_task_policy and returns tagged_tuple<tag::in1(FwdIter1), tag::in2(FwdIter2), tag::out(FwdIter3)> otherwise. The transform algorithm returns a tuple holding an iterator referring to the first element after the first input sequence, an iterator referring to the first element after the second input sequence, and the output iterator referring to the element in the destination range, one past the last element copied.
-
template<typename
ExPolicy
, typenameFwdIter1
, typenameFwdIter2
, typenameFwdIter3
, typenameF
, typenameProj1
= util::projection_identity, typenameProj2
= util::projection_identity>
util::detail::algorithm_result<ExPolicy, hpx::util::tagged_tuple<tag::in1(FwdIter1), tag::in2(FwdIter2), tag::out(FwdIter3)>>::typetransform
(ExPolicy &&policy, FwdIter1 first1, FwdIter1 last1, FwdIter2 first2, FwdIter2 last2, FwdIter3 dest, F &&f, Proj1 &&proj1 = Proj1(), Proj2 &&proj2 = Proj2())¶ Applies the given function f to pairs of elements from two ranges: one defined by [first1, last1) and the other beginning at first2, and stores the result in another range, beginning at dest.
The invocations of
f in the parallel transform algorithm invoked with an execution policy object of type sequenced_policy execute in sequential order in the calling thread.- Note
- Complexity: Exactly min(last2-first2, last1-first1) applications of f
- Template Parameters
ExPolicy
: The type of the execution policy to use (deduced). It describes the manner in which the execution of the algorithm may be parallelized and the manner in which it executes the invocations of f.FwdIter1
: The type of the source iterators for the first range used (deduced). This iterator type must meet the requirements of an forward iterator.FwdIter2
: The type of the source iterators for the second range used (deduced). This iterator type must meet the requirements of an forward iterator.FwdIter3
: The type of the iterator representing the destination range (deduced). This iterator type must meet the requirements of an forward iterator.F
: The type of the function/function object to use (deduced). Unlike its sequential form, the parallel overload of transform requires F to meet the requirements of CopyConstructible.Proj1
: The type of an optional projection function to be used for elements of the first sequence. This defaults to util::projection_identityProj2
: The type of an optional projection function to be used for elements of the second sequence. This defaults to util::projection_identity
- Parameters
policy
: The execution policy to use for the scheduling of the iterations.first1
: Refers to the beginning of the first sequence of elements the algorithm will be applied to.last1
: Refers to the end of the first sequence of elements the algorithm will be applied to.first2
: Refers to the beginning of the second sequence of elements the algorithm will be applied to.last2
: Refers to the end of the second sequence of elements the algorithm will be applied to.dest
: Refers to the beginning of the destination range.f
: Specifies the function (or function object) which will be invoked for each of the elements in the sequence specified by [first, last).This is a binary predicate. The signature of this predicate should be equivalent to:Ret fun(const Type1 &a, const Type2 &b);
The signature does not need to have const&. The types
Type1 and Type2 must be such that objects of types FwdIter1 and FwdIter2 can be dereferenced and then implicitly converted to Type1 and Type2 respectively. The type Ret must be such that an object of type FwdIter3 can be dereferenced and assigned a value of type Ret.proj1
: Specifies the function (or function object) which will be invoked for each of the elements of the first sequence as a projection operation before the actual predicate f is invoked.proj2
: Specifies the function (or function object) which will be invoked for each of the elements of the second sequence as a projection operation before the actual predicate f is invoked.
The invocations of f in the parallel transform algorithm invoked with an execution policy object of type parallel_policy or parallel_task_policy are permitted to execute in an unordered fashion in unspecified threads, and indeterminately sequenced within each thread.
- Note
- The algorithm will invoke the binary predicate until it reaches the end of the shorter of the two given input sequences
- Return
- The transform algorithm returns a hpx::future<tagged_tuple<tag::in1(FwdIter1), tag::in2(FwdIter2), tag::out(FwdIter3)> > if the execution policy is of type parallel_task_policy and returns tagged_tuple<tag::in1(FwdIter1), tag::in2(FwdIter2), tag::out(FwdIter3)> otherwise. The transform algorithm returns a tuple holding an iterator referring to the first element after the first input sequence, an iterator referring to the first element after the second input sequence, and the output iterator referring to the element in the destination range, one past the last element copied.
-
template<typename
ExPolicy
, typenameFwdIter1
, typenameFwdIter2
, typenameT
, typenameOp
, typenameConv
>
util::detail::algorithm_result<ExPolicy, FwdIter2>::typetransform_exclusive_scan
(ExPolicy &&policy, FwdIter1 first, FwdIter1 last, FwdIter2 dest, T init, Op &&op, Conv &&conv)¶ Assigns through each iterator i in [result, result + (last - first)) the value of GENERALIZED_NONCOMMUTATIVE_SUM(binary_op, init, conv(*first), …, conv(*(first + (i - result) - 1))).
The reduce operations in the parallel
transform_exclusive_scan algorithm invoked with an execution policy object of type sequenced_policy execute in sequential order in the calling thread.- Note
- Complexity: O(last - first) applications of the predicates op and conv.
- Template Parameters
ExPolicy
: The type of the execution policy to use (deduced). It describes the manner in which the execution of the algorithm may be parallelized and the manner in which it executes the assignments.FwdIter1
: The type of the source iterators used (deduced). This iterator type must meet the requirements of an forward iterator.FwdIter2
: The type of the iterator representing the destination range (deduced). This iterator type must meet the requirements of an forward iterator.Conv
: The type of the unary function object used for the conversion operation.T
: The type of the value to be used as initial (and intermediate) values (deduced).Op
: The type of the binary function object used for the reduction operation.
- Parameters
policy
: The execution policy to use for the scheduling of the iterations.first
: Refers to the beginning of the sequence of elements the algorithm will be applied to.last
: Refers to the end of the sequence of elements the algorithm will be applied to.dest
: Refers to the beginning of the destination range.conv
: Specifies the function (or function object) which will be invoked for each of the elements in the sequence specified by [first, last). This is a unary predicate. The signature of this predicate should be equivalent to:R fun(const Type &a);
The signature does not need to have const&, but the function must not modify the objects passed to it. The type
Type must be such that an object of type FwdIter1 can be dereferenced and then implicitly converted to Type. The type R must be such that an object of this type can be implicitly converted to T.init
: The initial value for the generalized sum.op
: Specifies the function (or function object) which will be invoked for each of the values of the input sequence. This is a binary predicate. The signature of this predicate should be equivalent to:Ret fun(const Type1 &a, const Type1 &b);
The signature does not need to have const&, but the function must not modify the objects passed to it. The types
Type1 and Ret must be such that an object of a type as given by the input sequence can be implicitly converted to any of those types.
The reduce operations in the parallel transform_exclusive_scan algorithm invoked with an execution policy object of type parallel_policy or parallel_task_policy are permitted to execute in an unordered fashion in unspecified threads, and indeterminately sequenced within each thread.
Neither
conv nor op shall invalidate iterators or subranges, or modify elements in the ranges [first,last) or [result,result + (last - first)).- Return
- The copy_n algorithm returns a hpx::future<FwdIter2> if the execution policy is of type sequenced_task_policy or parallel_task_policy and returns FwdIter2 otherwise. The transform_exclusive_scan algorithm returns the output iterator to the element in the destination range, one past the last element copied.
- Note
- GENERALIZED_NONCOMMUTATIVE_SUM(op, a1, …, aN) is defined as:
- a1 when N is 1
- op(GENERALIZED_NONCOMMUTATIVE_SUM(op, a1, …, aK), GENERALIZED_NONCOMMUTATIVE_SUM(op, aM, …, aN) where 1 < K+1 = M <= N.
The behavior of transform_exclusive_scan may be non-deterministic for a non-associative predicate.
-
template<typename
ExPolicy
, typenameFwdIter1
, typenameFwdIter2
, typenameOp
, typenameConv
, typenameT
>
util::detail::algorithm_result<ExPolicy, FwdIter2>::typetransform_inclusive_scan
(ExPolicy &&policy, FwdIter1 first, FwdIter1 last, FwdIter2 dest, Op &&op, Conv &&conv, T init)¶ Assigns through each iterator i in [result, result + (last - first)) the value of GENERALIZED_NONCOMMUTATIVE_SUM(op, init, conv(*first), …, conv(*(first + (i - result)))).
The reduce operations in the parallel
transform_inclusive_scan algorithm invoked with an execution policy object of type sequenced_policy execute in sequential order in the calling thread.- Note
- Complexity: O(last - first) applications of the predicate op.
- Template Parameters
ExPolicy
: The type of the execution policy to use (deduced). It describes the manner in which the execution of the algorithm may be parallelized and the manner in which it executes the assignments.FwdIter1
: The type of the source iterators used (deduced). This iterator type must meet the requirements of an forward iterator.FwdIter2
: The type of the iterator representing the destination range (deduced). This iterator type must meet the requirements of an forward iterator.Conv
: The type of the unary function object used for the conversion operation.T
: The type of the value to be used as initial (and intermediate) values (deduced).Op
: The type of the binary function object used for the reduction operation.
- Parameters
policy
: The execution policy to use for the scheduling of the iterations.first
: Refers to the beginning of the sequence of elements the algorithm will be applied to.last
: Refers to the end of the sequence of elements the algorithm will be applied to.dest
: Refers to the beginning of the destination range.conv
: Specifies the function (or function object) which will be invoked for each of the elements in the sequence specified by [first, last). This is a unary predicate. The signature of this predicate should be equivalent to:R fun(const Type &a);
The signature does not need to have const&, but the function must not modify the objects passed to it. The type
Type must be such that an object of type FwdIter1 can be dereferenced and then implicitly converted to Type. The type R must be such that an object of this type can be implicitly converted to T.init
: The initial value for the generalized sum.op
: Specifies the function (or function object) which will be invoked for each of the values of the input sequence. This is a binary predicate. The signature of this predicate should be equivalent to:Ret fun(const Type1 &a, const Type1 &b);
The signature does not need to have const&, but the function must not modify the objects passed to it. The types
Type1 and Ret must be such that an object of a type as given by the input sequence can be implicitly converted to any of those types.
The reduce operations in the parallel transform_inclusive_scan algorithm invoked with an execution policy object of type parallel_policy or parallel_task_policy are permitted to execute in an unordered fashion in unspecified threads, and indeterminately sequenced within each thread.
Neither
conv nor op shall invalidate iterators or subranges, or modify elements in the ranges [first,last) or [result,result + (last - first)).- Return
- The copy_n algorithm returns a hpx::future<FwdIter2> if the execution policy is of type sequenced_task_policy or parallel_task_policy and returns FwdIter2 otherwise. The transform_inclusive_scan algorithm returns the output iterator to the element in the destination range, one past the last element copied.
- Note
- GENERALIZED_NONCOMMUTATIVE_SUM(op, a1, …, aN) is defined as:
- a1 when N is 1
- op(GENERALIZED_NONCOMMUTATIVE_SUM(op, a1, …, aK), GENERALIZED_NONCOMMUTATIVE_SUM(op, aM, …, aN)) where 1 < K+1 = M <= N.
The difference between exclusive_scan and transform_inclusive_scan is that transform_inclusive_scan includes the ith input element in the ith sum. If op is not mathematically associative, the behavior of transform_inclusive_scan may be non-deterministic.
-
template<typename
ExPolicy
, typenameFwdIter1
, typenameFwdIter2
, typenameConv
, typenameOp
>
util::detail::algorithm_result<ExPolicy, FwdIter2>::typetransform_inclusive_scan
(ExPolicy &&policy, FwdIter1 first, FwdIter1 last, FwdIter2 dest, Op &&op, Conv &&conv)¶ Assigns through each iterator i in [result, result + (last - first)) the value of GENERALIZED_NONCOMMUTATIVE_SUM(op, conv(*first), …, conv(*(first + (i - result)))).
The reduce operations in the parallel
transform_inclusive_scan algorithm invoked with an execution policy object of type sequenced_policy execute in sequential order in the calling thread.- Note
- Complexity: O(last - first) applications of the predicate op.
- Template Parameters
ExPolicy
: The type of the execution policy to use (deduced). It describes the manner in which the execution of the algorithm may be parallelized and the manner in which it executes the assignments.FwdIter1
: The type of the source iterators used (deduced). This iterator type must meet the requirements of an forward iterator.FwdIter2
: The type of the iterator representing the destination range (deduced). This iterator type must meet the requirements of an forward iterator.Conv
: The type of the unary function object used for the conversion operation.T
: The type of the value to be used as initial (and intermediate) values (deduced).Op
: The type of the binary function object used for the reduction operation.
- Parameters
policy
: The execution policy to use for the scheduling of the iterations.first
: Refers to the beginning of the sequence of elements the algorithm will be applied to.last
: Refers to the end of the sequence of elements the algorithm will be applied to.dest
: Refers to the beginning of the destination range.conv
: Specifies the function (or function object) which will be invoked for each of the elements in the sequence specified by [first, last). This is a unary predicate. The signature of this predicate should be equivalent to:R fun(const Type &a);
The signature does not need to have const&, but the function must not modify the objects passed to it. The type
Type must be such that an object of type FwdIter1 can be dereferenced and then implicitly converted to Type. The type R must be such that an object of this type can be implicitly converted to T.op
: Specifies the function (or function object) which will be invoked for each of the values of the input sequence. This is a binary predicate. The signature of this predicate should be equivalent to:Ret fun(const Type1 &a, const Type1 &b);
The signature does not need to have const&, but the function must not modify the objects passed to it. The types
Type1 and Ret must be such that an object of a type as given by the input sequence can be implicitly converted to any of those types.
The reduce operations in the parallel transform_inclusive_scan algorithm invoked with an execution policy object of type parallel_policy or parallel_task_policy are permitted to execute in an unordered fashion in unspecified threads, and indeterminately sequenced within each thread.
Neither
conv nor op shall invalidate iterators or subranges, or modify elements in the ranges [first,last) or [result,result + (last - first)).- Return
- The copy_n algorithm returns a hpx::future<FwdIter2> if the execution policy is of type sequenced_task_policy or parallel_task_policy and returns FwdIter2 otherwise. The transform_inclusive_scan algorithm returns the output iterator to the element in the destination range, one past the last element copied.
- Note
- GENERALIZED_NONCOMMUTATIVE_SUM(op, a1, …, aN) is defined as:
- a1 when N is 1
- op(GENERALIZED_NONCOMMUTATIVE_SUM(op, a1, …, aK), GENERALIZED_NONCOMMUTATIVE_SUM(op, aM, …, aN)) where 1 < K+1 = M <= N.
The difference between exclusive_scan and transform_inclusive_scan is that transform_inclusive_scan includes the ith input element in the ith sum.
-
template<typename
ExPolicy
, typenameFwdIter
, typenameT
, typenameReduce
, typenameConvert
>
util::detail::algorithm_result<ExPolicy, T>::typetransform_reduce
(ExPolicy &&policy, FwdIter first, FwdIter last, T init, Reduce &&red_op, Convert &&conv_op)¶ Returns GENERALIZED_SUM(red_op, init, conv_op(*first), …, conv_op(*(first + (last - first) - 1))).
The reduce operations in the parallel
transform_reduce algorithm invoked with an execution policy object of type sequenced_policy execute in sequential order in the calling thread.- Note
- Complexity: O(last - first) applications of the predicates red_op and conv_op.
- Template Parameters
ExPolicy
: The type of the execution policy to use (deduced). It describes the manner in which the execution of the algorithm may be parallelized and the manner in which it executes the assignments.FwdIter
: The type of the source iterators used (deduced). This iterator type must meet the requirements of an input iterator.F
: The type of the function/function object to use (deduced). Unlike its sequential form, the parallel overload of copy_if requires F to meet the requirements of CopyConstructible.T
: The type of the value to be used as initial (and intermediate) values (deduced).Reduce
: The type of the binary function object used for the reduction operation.Convert
: The type of the unary function object used to transform the elements of the input sequence before invoking the reduce function.
- Parameters
policy
: The execution policy to use for the scheduling of the iterations.first
: Refers to the beginning of the sequence of elements the algorithm will be applied to.last
: Refers to the end of the sequence of elements the algorithm will be applied to.conv_op
: Specifies the function (or function object) which will be invoked for each of the elements in the sequence specified by [first, last). This is a unary predicate. The signature of this predicate should be equivalent to:R fun(const Type &a);
The signature does not need to have const&, but the function must not modify the objects passed to it. The type
Type must be such that an object of type FwdIter can be dereferenced and then implicitly converted to Type. The type R must be such that an object of this type can be implicitly converted to T.init
: The initial value for the generalized sum.red_op
: Specifies the function (or function object) which will be invoked for each of the values returned from the invocation of conv_op. This is a binary predicate. The signature of this predicate should be equivalent to:Ret fun(const Type1 &a, const Type2 &b);
The signature does not need to have const&, but the function must not modify the objects passed to it. The types
Type1, Type2, and Ret must be such that an object of a type as returned from conv_op can be implicitly converted to any of those types.
The reduce operations in the parallel transform_reduce algorithm invoked with an execution policy object of type parallel_policy or parallel_task_policy are permitted to execute in an unordered fashion in unspecified threads, and indeterminately sequenced within each thread.
The difference between
transform_reduce and accumulate is that the behavior of transform_reduce may be non-deterministic for non-associative or non-commutative binary predicate.- Return
- The transform_reduce algorithm returns a hpx::future<T> if the execution policy is of type parallel_task_policy and returns T otherwise. The transform_reduce algorithm returns the result of the generalized sum over the values returned from conv_op when applied to the elements given by the input range [first, last).
- Note
- GENERALIZED_SUM(op, a1, …, aN) is defined as follows:
- a1 when N is 1
- op(GENERALIZED_SUM(op, b1, …, bK), GENERALIZED_SUM(op, bM, …, bN)), where:
- b1, …, bN may be any permutation of a1, …, aN and
- 1 < K+1 = M <= N.
-
template<typename
ExPolicy
, typenameFwdIter1
, typenameFwdIter2
, typenameT
>
util::detail::algorithm_result<ExPolicy, T>::typetransform_reduce
(ExPolicy &&policy, FwdIter1 first1, FwdIter1 last1, FwdIter2 first2, T init)¶ Returns the result of accumulating init with the inner products of the pairs formed by the elements of two ranges starting at first1 and first2.
The operations in the parallel
transform_reduce algorithm invoked with an execution policy object of type sequenced_policy execute in sequential order in the calling thread.- Note
- Complexity: O(last - first) applications of the predicate op2.
- Template Parameters
ExPolicy
: The type of the execution policy to use (deduced). It describes the manner in which the execution of the algorithm may be parallelized and the manner in which it executes the assignments.FwdIter1
: The type of the first source iterators used (deduced). This iterator type must meet the requirements of an forward iterator.FwdIter2
: The type of the second source iterators used (deduced). This iterator type must meet the requirements of an forward iterator.T
: The type of the value to be used as return) values (deduced).
- Parameters
policy
: The execution policy to use for the scheduling of the iterations.first1
: Refers to the beginning of the first sequence of elements the result will be calculated with.last1
: Refers to the end of the first sequence of elements the algorithm will be applied to.first2
: Refers to the beginning of the second sequence of elements the result will be calculated with.init
: The initial value for the sum.
The operations in the parallel transform_reduce algorithm invoked with an execution policy object of type parallel_policy or parallel_task_policy are permitted to execute in an unordered fashion in unspecified threads, and indeterminately sequenced within each thread.
- Return
- The transform_reduce algorithm returns a hpx::future<T> if the execution policy is of type sequenced_task_policy or parallel_task_policy and returns T otherwise.
-
template<typename
ExPolicy
, typenameFwdIter1
, typenameFwdIter2
, typenameT
, typenameReduce
, typenameConvert
>
util::detail::algorithm_result<ExPolicy, T>::typetransform_reduce
(ExPolicy &&policy, FwdIter1 first1, FwdIter1 last1, FwdIter2 first2, T init, Reduce &&red_op, Convert &&conv_op)¶ Returns the result of accumulating init with the inner products of the pairs formed by the elements of two ranges starting at first1 and first2.
The operations in the parallel
transform_reduce algorithm invoked with an execution policy object of type sequenced_policy execute in sequential order in the calling thread.- Note
- Complexity: O(last - first) applications of the predicate op2.
- Template Parameters
ExPolicy
: The type of the execution policy to use (deduced). It describes the manner in which the execution of the algorithm may be parallelized and the manner in which it executes the assignments.FwdIter1
: The type of the first source iterators used (deduced). This iterator type must meet the requirements of an forward iterator.FwdIter2
: The type of the second source iterators used (deduced). This iterator type must meet the requirements of an forward iterator.T
: The type of the value to be used as return) values (deduced).Reduce
: The type of the binary function object used for the multiplication operation.Convert
: The type of the unary function object used to transform the elements of the input sequence before invoking the reduce function.
- Parameters
policy
: The execution policy to use for the scheduling of the iterations.first1
: Refers to the beginning of the first sequence of elements the result will be calculated with.last1
: Refers to the end of the first sequence of elements the algorithm will be applied to.first2
: Refers to the beginning of the second sequence of elements the result will be calculated with.init
: The initial value for the sum.red_op
: Specifies the function (or function object) which will be invoked for the initial value and each of the return values of op2. This is a binary predicate. The signature of this predicate should be equivalent to should be equivalent to:Ret fun(const Type1 &a, const Type1 &b);
The signature does not need to have const&, but the function must not modify the objects passed to it. The type
Ret must be such that it can be implicitly converted to a type of T.conv_op
: Specifies the function (or function object) which will be invoked for each of the input values of the sequence. This is a binary predicate. The signature of this predicate should be equivalent toRet fun(const Type1 &a, const Type2 &b);
The signature does not need to have const&, but the function must not modify the objects passed to it. The type
Ret must be such that it can be implicitly converted to an object for the second argument type of op1.
The operations in the parallel transform_reduce algorithm invoked with an execution policy object of type parallel_policy or parallel_task_policy are permitted to execute in an unordered fashion in unspecified threads, and indeterminately sequenced within each thread.
- Return
- The transform_reduce algorithm returns a hpx::future<T> if the execution policy is of type sequenced_task_policy or parallel_task_policy and returns T otherwise.
-
template<typename
ExPolicy
, typenameFwdIter1
, typenameFwdIter2
>
std::enable_if<execution::is_execution_policy<ExPolicy>::value, typename util::detail::algorithm_result<ExPolicy, FwdIter2>::type>::typeuninitialized_copy
(ExPolicy &&policy, FwdIter1 first, FwdIter1 last, FwdIter2 dest)¶ Copies the elements in the range, defined by [first, last), to an uninitialized memory area beginning at dest. If an exception is thrown during the copy operation, the function has no effects.
The assignments in the parallel
uninitialized_copy algorithm invoked with an execution policy object of type sequenced_policy execute in sequential order in the calling thread.- Note
- Complexity: Performs exactly last - first assignments.
- Template Parameters
ExPolicy
: The type of the execution policy to use (deduced). It describes the manner in which the execution of the algorithm may be parallelized and the manner in which it executes the assignments.FwdIter1
: The type of the source iterators used (deduced). This iterator type must meet the requirements of an forward iterator.FwdIter2
: The type of the iterator representing the destination range (deduced). This iterator type must meet the requirements of a forward iterator.
- Parameters
policy
: The execution policy to use for the scheduling of the iterations.first
: Refers to the beginning of the sequence of elements the algorithm will be applied to.last
: Refers to the end of the sequence of elements the algorithm will be applied to.dest
: Refers to the beginning of the destination range.
The assignments in the parallel uninitialized_copy algorithm invoked with an execution policy object of type parallel_policy or parallel_task_policy are permitted to execute in an unordered fashion in unspecified threads, and indeterminately sequenced within each thread.
- Return
- The uninitialized_copy algorithm returns a hpx::future<FwdIter2>, if the execution policy is of type sequenced_task_policy or parallel_task_policy and returns FwdIter2 otherwise. The uninitialized_copy algorithm returns the output iterator to the element in the destination range, one past the last element copied.
-
template<typename
ExPolicy
, typenameFwdIter1
, typenameSize
, typenameFwdIter2
>
std::enable_if<execution::is_execution_policy<ExPolicy>::value, typename util::detail::algorithm_result<ExPolicy, FwdIter2>::type>::typeuninitialized_copy_n
(ExPolicy &&policy, FwdIter1 first, Size count, FwdIter2 dest)¶ Copies the elements in the range [first, first + count), starting from first and proceeding to first + count - 1., to another range beginning at dest. If an exception is thrown during the copy operation, the function has no effects.
The assignments in the parallel
uninitialized_copy_n algorithm invoked with an execution policy object of type sequenced_policy execute in sequential order in the calling thread.- Note
- Complexity: Performs exactly count assignments, if count > 0, no assignments otherwise.
- Template Parameters
ExPolicy
: The type of the execution policy to use (deduced). It describes the manner in which the execution of the algorithm may be parallelized and the manner in which it executes the assignments.FwdIter1
: The type of the source iterators used (deduced). This iterator type must meet the requirements of an input iterator.Size
: The type of the argument specifying the number of elements to apply f to.FwdIter2
: The type of the iterator representing the destination range (deduced). This iterator type must meet the requirements of a forward iterator.
- Parameters
policy
: The execution policy to use for the scheduling of the iterations.first
: Refers to the beginning of the sequence of elements the algorithm will be applied to.count
: Refers to the number of elements starting at first the algorithm will be applied to.dest
: Refers to the beginning of the destination range.
The assignments in the parallel uninitialized_copy_n algorithm invoked with an execution policy object of type parallel_policy or parallel_task_policy are permitted to execute in an unordered fashion in unspecified threads, and indeterminately sequenced within each thread.
- Return
- The uninitialized_copy_n algorithm returns a hpx::future<FwdIter2> if the execution policy is of type sequenced_task_policy or parallel_task_policy and returns FwdIter2 otherwise. The uninitialized_copy_n algorithm returns the output iterator to the element in the destination range, one past the last element copied.
-
template<typename
ExPolicy
, typenameFwdIter
>
util::detail::algorithm_result<ExPolicy>::typeuninitialized_default_construct
(ExPolicy &&policy, FwdIter first, FwdIter last)¶ Constructs objects of type typename iterator_traits<ForwardIt>::value_type in the uninitialized storage designated by the range [first, last) by default-initialization. If an exception is thrown during the initialization, the function has no effects.
The assignments in the parallel
uninitialized_default_construct algorithm invoked with an execution policy object of type sequenced_policy execute in sequential order in the calling thread.- Note
- Complexity: Performs exactly last - first assignments.
- Template Parameters
ExPolicy
: The type of the execution policy to use (deduced). It describes the manner in which the execution of the algorithm may be parallelized and the manner in which it executes the assignments.FwdIter
: The type of the source iterators used (deduced). This iterator type must meet the requirements of an forward iterator.
- Parameters
policy
: The execution policy to use for the scheduling of the iterations.first
: Refers to the beginning of the sequence of elements the algorithm will be applied to.last
: Refers to the end of the sequence of elements the algorithm will be applied to.
The assignments in the parallel uninitialized_default_construct algorithm invoked with an execution policy object of type parallel_policy or parallel_task_policy are permitted to execute in an unordered fashion in unspecified threads, and indeterminately sequenced within each thread.
- Return
- The uninitialized_default_construct algorithm returns a hpx::future<void>, if the execution policy is of type sequenced_task_policy or parallel_task_policy and returns void otherwise.
-
template<typename
ExPolicy
, typenameFwdIter
, typenameSize
>
util::detail::algorithm_result<ExPolicy, FwdIter>::typeuninitialized_default_construct_n
(ExPolicy &&policy, FwdIter first, Size count)¶ Constructs objects of type typename iterator_traits<ForwardIt>::value_type in the uninitialized storage designated by the range [first, first + count) by default-initialization. If an exception is thrown during the initialization, the function has no effects.
The assignments in the parallel
uninitialized_default_construct_n algorithm invoked with an execution policy object of type sequenced_policy execute in sequential order in the calling thread.- Note
- Complexity: Performs exactly count assignments, if count > 0, no assignments otherwise.
- Template Parameters
ExPolicy
: The type of the execution policy to use (deduced). It describes the manner in which the execution of the algorithm may be parallelized and the manner in which it executes the assignments.FwdIter
: The type of the source iterators used (deduced). This iterator type must meet the requirements of an forward iterator.Size
: The type of the argument specifying the number of elements to apply f to.
- Parameters
policy
: The execution policy to use for the scheduling of the iterations.first
: Refers to the beginning of the sequence of elements the algorithm will be applied to.count
: Refers to the number of elements starting at first the algorithm will be applied to.
The assignments in the parallel uninitialized_default_construct_n algorithm invoked with an execution policy object of type parallel_policy or parallel_task_policy are permitted to execute in an unordered fashion in unspecified threads, and indeterminately sequenced within each thread.
- Return
- The uninitialized_default_construct_n algorithm returns a hpx::future<FwdIter> if the execution policy is of type sequenced_task_policy or parallel_task_policy and returns FwdIter otherwise. The uninitialized_default_construct_n algorithm returns the iterator to the element in the source range, one past the last element constructed.
-
template<typename
ExPolicy
, typenameFwdIter
, typenameT
>
std::enable_if<execution::is_execution_policy<ExPolicy>::value, typename util::detail::algorithm_result<ExPolicy>::type>::typeuninitialized_fill
(ExPolicy &&policy, FwdIter first, FwdIter last, T const &value)¶ Copies the given value to an uninitialized memory area, defined by the range [first, last). If an exception is thrown during the initialization, the function has no effects.
The initializations in the parallel
uninitialized_fill algorithm invoked with an execution policy object of type sequenced_policy execute in sequential order in the calling thread.- Note
- Complexity: Linear in the distance between first and last
- Template Parameters
ExPolicy
: The type of the execution policy to use (deduced). It describes the manner in which the execution of the algorithm may be parallelized and the manner in which it executes the assignments.FwdIter
: The type of the source iterators used (deduced). This iterator type must meet the requirements of an forward iterator.T
: The type of the value to be assigned (deduced).
- Parameters
policy
: The execution policy to use for the scheduling of the iterations.first
: Refers to the beginning of the sequence of elements the algorithm will be applied to.last
: Refers to the end of the sequence of elements the algorithm will be applied to.value
: The value to be assigned.
The initializations in the parallel uninitialized_fill algorithm invoked with an execution policy object of type parallel_policy or parallel_task_policy are permitted to execute in an unordered fashion in unspecified threads, and indeterminately sequenced within each thread.
- Return
- The uninitialized_fill algorithm returns a hpx::future<void>, if the execution policy is of type sequenced_task_policy or parallel_task_policy and returns nothing otherwise.
-
template<typename
ExPolicy
, typenameFwdIter
, typenameSize
, typenameT
>
std::enable_if<execution::is_execution_policy<ExPolicy>::value, typename util::detail::algorithm_result<ExPolicy>::type>::typeuninitialized_fill_n
(ExPolicy &&policy, FwdIter first, Size count, T const &value)¶ Copies the given value value to the first count elements in an uninitialized memory area beginning at first. If an exception is thrown during the initialization, the function has no effects.
The initializations in the parallel
uninitialized_fill_n algorithm invoked with an execution policy object of type sequenced_policy execute in sequential order in the calling thread.- Note
- Complexity: Performs exactly count assignments, if count > 0, no assignments otherwise.
- Template Parameters
ExPolicy
: The type of the execution policy to use (deduced). It describes the manner in which the execution of the algorithm may be parallelized and the manner in which it executes the assignments.FwdIter
: The type of the source iterators used (deduced). This iterator type must meet the requirements of a forward iterator.Size
: The type of the argument specifying the number of elements to apply f to.T
: The type of the value to be assigned (deduced).
- Parameters
policy
: The execution policy to use for the scheduling of the iterations.first
: Refers to the beginning of the sequence of elements the algorithm will be applied to.count
: Refers to the number of elements starting at first the algorithm will be applied to.value
: The value to be assigned.
The initializations in the parallel uninitialized_fill_n algorithm invoked with an execution policy object of type parallel_policy or parallel_task_policy are permitted to execute in an unordered fashion in unspecified threads, and indeterminately sequenced within each thread.
- Return
- The uninitialized_fill_n algorithm returns a hpx::future<void>, if the execution policy is of type sequenced_task_policy or parallel_task_policy and returns nothing otherwise.
-
template<typename
ExPolicy
, typenameFwdIter1
, typenameFwdIter2
>
util::detail::algorithm_result<ExPolicy, FwdIter2>::typeuninitialized_move
(ExPolicy &&policy, FwdIter1 first, FwdIter1 last, FwdIter2 dest)¶ Moves the elements in the range, defined by [first, last), to an uninitialized memory area beginning at dest. If an exception is thrown during the initialization, some objects in [first, last) are left in a valid but unspecified state.
The assignments in the parallel
uninitialized_move algorithm invoked with an execution policy object of type sequenced_policy execute in sequential order in the calling thread.- Note
- Complexity: Performs exactly last - first move operations.
- Template Parameters
ExPolicy
: The type of the execution policy to use (deduced). It describes the manner in which the execution of the algorithm may be parallelized and the manner in which it executes the assignments.FwdIter1
: The type of the source iterators used (deduced). This iterator type must meet the requirements of an forward iterator.FwdIter2
: The type of the iterator representing the destination range (deduced). This iterator type must meet the requirements of a forward iterator.
- Parameters
policy
: The execution policy to use for the scheduling of the iterations.first
: Refers to the beginning of the sequence of elements the algorithm will be applied to.last
: Refers to the end of the sequence of elements the algorithm will be applied to.dest
: Refers to the beginning of the destination range.
The assignments in the parallel uninitialized_move algorithm invoked with an execution policy object of type parallel_policy or parallel_task_policy are permitted to execute in an unordered fashion in unspecified threads, and indeterminately sequenced within each thread.
- Return
- The uninitialized_move algorithm returns a hpx::future<FwdIter2>, if the execution policy is of type sequenced_task_policy or parallel_task_policy and returns FwdIter2 otherwise. The uninitialized_move algorithm returns the output iterator to the element in the destination range, one past the last element moved.
-
template<typename
ExPolicy
, typenameFwdIter1
, typenameSize
, typenameFwdIter2
>
util::detail::algorithm_result<ExPolicy, hpx::util::tagged_pair<tag::in(FwdIter1), tag::out(FwdIter2)>>::typeuninitialized_move_n
(ExPolicy &&policy, FwdIter1 first, Size count, FwdIter2 dest)¶ Moves the elements in the range [first, first + count), starting from first and proceeding to first + count - 1., to another range beginning at dest. If an exception is thrown during the initialization, some objects in [first, first + count) are left in a valid but unspecified state.
The assignments in the parallel
uninitialized_move_n algorithm invoked with an execution policy object of type sequenced_policy execute in sequential order in the calling thread.- Note
- Complexity: Performs exactly count movements, if count > 0, no move operations otherwise.
- Template Parameters
ExPolicy
: The type of the execution policy to use (deduced). It describes the manner in which the execution of the algorithm may be parallelized and the manner in which it executes the assignments.FwdIter1
: The type of the source iterators used (deduced). This iterator type must meet the requirements of an forward iterator.Size
: The type of the argument specifying the number of elements to apply f to.FwdIter2
: The type of the iterator representing the destination range (deduced). This iterator type must meet the requirements of a forward iterator.
- Parameters
policy
: The execution policy to use for the scheduling of the iterations.first
: Refers to the beginning of the sequence of elements the algorithm will be applied to.count
: Refers to the number of elements starting at first the algorithm will be applied to.dest
: Refers to the beginning of the destination range.
The assignments in the parallel uninitialized_move_n algorithm invoked with an execution policy object of type parallel_policy or parallel_task_policy are permitted to execute in an unordered fashion in unspecified threads, and indeterminately sequenced within each thread.
- Return
- The uninitialized_move_n algorithm returns a hpx::future<std::pair<FwdIter1, FwdIter2>> if the execution policy is of type sequenced_task_policy or parallel_task_policy and returns std::pair<FwdIter1, FwdIter2> otherwise. The uninitialized_move_n algorithm returns the pair of the input iterator to the element past in the source range and an output iterator to the element in the destination range, one past the last element moved.
-
template<typename
ExPolicy
, typenameFwdIter
>
util::detail::algorithm_result<ExPolicy>::typeuninitialized_value_construct
(ExPolicy &&policy, FwdIter first, FwdIter last)¶ Constructs objects of type typename iterator_traits<ForwardIt>::value_type in the uninitialized storage designated by the range [first, last) by default-initialization. If an exception is thrown during the initialization, the function has no effects.
The assignments in the parallel
uninitialized_value_construct algorithm invoked with an execution policy object of type sequenced_policy execute in sequential order in the calling thread.- Note
- Complexity: Performs exactly last - first assignments.
- Template Parameters
ExPolicy
: The type of the execution policy to use (deduced). It describes the manner in which the execution of the algorithm may be parallelized and the manner in which it executes the assignments.FwdIter
: The type of the source iterators used (deduced). This iterator type must meet the requirements of an forward iterator.
- Parameters
policy
: The execution policy to use for the scheduling of the iterations.first
: Refers to the beginning of the sequence of elements the algorithm will be applied to.last
: Refers to the end of the sequence of elements the algorithm will be applied to.
The assignments in the parallel uninitialized_value_construct algorithm invoked with an execution policy object of type parallel_policy or parallel_task_policy are permitted to execute in an unordered fashion in unspecified threads, and indeterminately sequenced within each thread.
- Return
- The uninitialized_value_construct algorithm returns a hpx::future<void>, if the execution policy is of type sequenced_task_policy or parallel_task_policy and returns void otherwise.
-
template<typename
ExPolicy
, typenameFwdIter
, typenameSize
>
util::detail::algorithm_result<ExPolicy, FwdIter>::typeuninitialized_value_construct_n
(ExPolicy &&policy, FwdIter first, Size count)¶ Constructs objects of type typename iterator_traits<ForwardIt>::value_type in the uninitialized storage designated by the range [first, first + count) by default-initialization. If an exception is thrown during the initialization, the function has no effects.
The assignments in the parallel
uninitialized_value_construct_n algorithm invoked with an execution policy object of type sequenced_policy execute in sequential order in the calling thread.- Note
- Complexity: Performs exactly count assignments, if count > 0, no assignments otherwise.
- Template Parameters
ExPolicy
: The type of the execution policy to use (deduced). It describes the manner in which the execution of the algorithm may be parallelized and the manner in which it executes the assignments.FwdIter
: The type of the source iterators used (deduced). This iterator type must meet the requirements of an forward iterator.Size
: The type of the argument specifying the number of elements to apply f to.
- Parameters
policy
: The execution policy to use for the scheduling of the iterations.first
: Refers to the beginning of the sequence of elements the algorithm will be applied to.count
: Refers to the number of elements starting at first the algorithm will be applied to.
The assignments in the parallel uninitialized_value_construct_n algorithm invoked with an execution policy object of type parallel_policy or parallel_task_policy are permitted to execute in an unordered fashion in unspecified threads, and indeterminately sequenced within each thread.
- Return
- The uninitialized_value_construct_n algorithm returns a hpx::future<FwdIter> if the execution policy is of type sequenced_task_policy or parallel_task_policy and returns FwdIter otherwise. The uninitialized_value_construct_n algorithm returns the iterator to the element in the source range, one past the last element constructed.
-
template<typename
ExPolicy
, typenameFwdIter
, typenamePred
= detail::equal_to, typenameProj
= util::projection_identity>
util::detail::algorithm_result<ExPolicy, FwdIter>::typeunique
(ExPolicy &&policy, FwdIter first, FwdIter last, Pred &&pred = Pred(), Proj &&proj = Proj())¶ Eliminates all but the first element from every consecutive group of equivalent elements from the range [first, last) and returns a past-the-end iterator for the new logical end of the range.
The assignments in the parallel
unique algorithm invoked with an execution policy object of type sequenced_policy execute in sequential order in the calling thread.- Note
- Complexity: Performs not more than last - first assignments, exactly last - first - 1 applications of the predicate pred and no more than twice as many applications of the projection proj.
- Template Parameters
ExPolicy
: The type of the execution policy to use (deduced). It describes the manner in which the execution of the algorithm may be parallelized and the manner in which it executes the assignments.FwdIter
: The type of the source iterators used (deduced). This iterator type must meet the requirements of an forward iterator.Pred
: The type of the function/function object to use (deduced). Unlike its sequential form, the parallel overload of unique requires Pred to meet the requirements of CopyConstructible. This defaults to std::equal_to<>Proj
: The type of an optional projection function. This defaults to util::projection_identity
- Parameters
policy
: The execution policy to use for the scheduling of the iterations.first
: Refers to the beginning of the sequence of elements the algorithm will be applied to.last
: Refers to the end of the sequence of elements the algorithm will be applied to.pred
: Specifies the function (or function object) which will be invoked for each of the elements in the sequence specified by [first, last). This is an binary predicate which returns true for the required elements. The signature of this predicate should be equivalent to:bool pred(const Type1 &a, const Type2 &b);
The signature does not need to have const&, but the function must not modify the objects passed to it. The types
Type1 and Type2 must be such that objects of types FwdIter can be dereferenced and then implicitly converted to both Type1 and Type2proj
: Specifies the function (or function object) which will be invoked for each of the elements as a projection operation before the actual predicate is invoked.
The assignments in the parallel unique algorithm invoked with an execution policy object of type parallel_policy or parallel_task_policy are permitted to execute in an unordered fashion in unspecified threads, and indeterminately sequenced within each thread.
- Return
- The unique algorithm returns a hpx::future<FwdIter> if the execution policy is of type sequenced_task_policy or parallel_task_policy and returns FwdIter otherwise. The unique algorithm returns the iterator to the new end of the range.
-
template<typename
ExPolicy
, typenameFwdIter1
, typenameFwdIter2
, typenamePred
= detail::equal_to, typenameProj
= util::projection_identity>
util::detail::algorithm_result<ExPolicy, hpx::util::tagged_pair<tag::in(FwdIter1), tag::out(FwdIter2)>>::typeunique_copy
(ExPolicy &&policy, FwdIter1 first, FwdIter1 last, FwdIter2 dest, Pred &&pred = Pred(), Proj &&proj = Proj())¶ Copies the elements from the range [first, last), to another range beginning at dest in such a way that there are no consecutive equal elements. Only the first element of each group of equal elements is copied.
The assignments in the parallel
unique_copy algorithm invoked with an execution policy object of type sequenced_policy execute in sequential order in the calling thread.- Note
- Complexity: Performs not more than last - first assignments, exactly last - first - 1 applications of the predicate pred and no more than twice as many applications of the projection proj
- Template Parameters
ExPolicy
: The type of the execution policy to use (deduced). It describes the manner in which the execution of the algorithm may be parallelized and the manner in which it executes the assignments.FwdIter1
: The type of the source iterators used (deduced). This iterator type must meet the requirements of an forward iterator.FwdIter2
: The type of the iterator representing the destination range (deduced). This iterator type must meet the requirements of an forward iterator.Pred
: The type of the function/function object to use (deduced). Unlike its sequential form, the parallel overload of unique_copy requires Pred to meet the requirements of CopyConstructible. This defaults to std::equal_to<>Proj
: The type of an optional projection function. This defaults to util::projection_identity
- Parameters
policy
: The execution policy to use for the scheduling of the iterations.first
: Refers to the beginning of the sequence of elements the algorithm will be applied to.last
: Refers to the end of the sequence of elements the algorithm will be applied to.dest
: Refers to the beginning of the destination range.pred
: Specifies the function (or function object) which will be invoked for each of the elements in the sequence specified by [first, last). This is an binary predicate which returns true for the required elements. The signature of this predicate should be equivalent to:bool pred(const Type &a, const Type &b);
The signature does not need to have const&, but the function must not modify the objects passed to it. The type
Type must be such that an object of type FwdIter1 can be dereferenced and then implicitly converted to Type.proj
: Specifies the function (or function object) which will be invoked for each of the elements as a projection operation before the actual predicate is invoked.
The assignments in the parallel unique_copy algorithm invoked with an execution policy object of type parallel_policy or parallel_task_policy are permitted to execute in an unordered fashion in unspecified threads, and indeterminately sequenced within each thread.
- Return
- The unique_copy algorithm returns a hpx::future<tagged_pair<tag::in(FwdIter1), tag::out(FwdIter2)> > if the execution policy is of type sequenced_task_policy or parallel_task_policy and returns tagged_pair<tag::in(FwdIter1), tag::out(FwdIter2)> otherwise. The unique_copy algorithm returns the pair of the source iterator to last, and the destination iterator to the end of the dest range.
-
template<typename
ExPolicy
, typenameRng
, typenameF
, typenameProj
= util::projection_identity>
util::detail::algorithm_result<ExPolicy, bool>::typenone_of
(ExPolicy &&policy, Rng &&rng, F &&f, Proj &&proj = Proj())¶ Checks if unary predicate f returns true for no elements in the range rng.
The application of function objects in parallel algorithm invoked with an execution policy object of type
sequenced_policy execute in sequential order in the calling thread.- Note
- Complexity: At most std::distance(begin(rng), end(rng)) applications of the predicate f
- Template Parameters
ExPolicy
: The type of the execution policy to use (deduced). It describes the manner in which the execution of the algorithm may be parallelized and the manner in which it applies user-provided function objects.Rng
: The type of the source range used (deduced). The iterators extracted from this range type must meet the requirements of an input iterator.F
: The type of the function/function object to use (deduced). Unlike its sequential form, the parallel overload of none_of requires F to meet the requirements of CopyConstructible.Proj
: The type of an optional projection function. This defaults to util::projection_identity
- Parameters
policy
: The execution policy to use for the scheduling of the iterations.rng
: Refers to the sequence of elements the algorithm will be applied to.f
: Specifies the function (or function object) which will be invoked for each of the elements in the sequence specified by [first, last). The signature of this predicate should be equivalent to:bool pred(const Type &a);
The signature does not need to have const&, but the function must not modify the objects passed to it. The type
Type must be such that an object of type FwdIter can be dereferenced and then implicitly converted to Type.proj
: Specifies the function (or function object) which will be invoked for each of the elements as a projection operation before the actual predicate is invoked.
The application of function objects in parallel algorithm invoked with an execution policy object of type parallel_policy or parallel_task_policy are permitted to execute in an unordered fashion in unspecified threads, and indeterminately sequenced within each thread.
- Return
- The none_of algorithm returns a hpx::future<bool> if the execution policy is of type sequenced_task_policy or parallel_task_policy and returns bool otherwise. The none_of algorithm returns true if the unary predicate f returns true for no elements in the range, false otherwise. It returns true if the range is empty.
-
template<typename
ExPolicy
, typenameRng
, typenameF
, typenameProj
= util::projection_identity>
util::detail::algorithm_result<ExPolicy, bool>::typeany_of
(ExPolicy &&policy, Rng &&rng, F &&f, Proj &&proj = Proj())¶ Checks if unary predicate f returns true for at least one element in the range rng.
The application of function objects in parallel algorithm invoked with an execution policy object of type
sequenced_policy execute in sequential order in the calling thread.- Note
- Complexity: At most std::distance(begin(rng), end(rng)) applications of the predicate f
- Template Parameters
ExPolicy
: The type of the execution policy to use (deduced). It describes the manner in which the execution of the algorithm may be parallelized and the manner in which it applies user-provided function objects.Rng
: The type of the source range used (deduced). The iterators extracted from this range type must meet the requirements of an input iterator.F
: The type of the function/function object to use (deduced). Unlike its sequential form, the parallel overload of none_of requires F to meet the requirements of CopyConstructible.Proj
: The type of an optional projection function. This defaults to util::projection_identity
- Parameters
policy
: The execution policy to use for the scheduling of the iterations.rng
: Refers to the sequence of elements the algorithm will be applied to.f
: Specifies the function (or function object) which will be invoked for each of the elements in the sequence specified by [first, last). The signature of this predicate should be equivalent to:bool pred(const Type &a);
The signature does not need to have const&, but the function must not modify the objects passed to it. The type
Type must be such that an object of type FwdIter can be dereferenced and then implicitly converted to Type.proj
: Specifies the function (or function object) which will be invoked for each of the elements as a projection operation before the actual predicate is invoked.
The application of function objects in parallel algorithm invoked with an execution policy object of type parallel_policy or parallel_task_policy are permitted to execute in an unordered fashion in unspecified threads, and indeterminately sequenced within each thread.
- Return
- The any_of algorithm returns a hpx::future<bool> if the execution policy is of type sequenced_task_policy or parallel_task_policy and returns bool otherwise. The any_of algorithm returns true if the unary predicate f returns true for at least one element in the range, false otherwise. It returns false if the range is empty.
-
template<typename
ExPolicy
, typenameRng
, typenameF
, typenameProj
= util::projection_identity>
util::detail::algorithm_result<ExPolicy, bool>::typeall_of
(ExPolicy &&policy, Rng &&rng, F &&f, Proj &&proj = Proj())¶ Checks if unary predicate f returns true for all elements in the range rng.
The application of function objects in parallel algorithm invoked with an execution policy object of type
sequenced_policy execute in sequential order in the calling thread.- Note
- Complexity: At most std::distance(begin(rng), end(rng)) applications of the predicate f
- Template Parameters
ExPolicy
: The type of the execution policy to use (deduced). It describes the manner in which the execution of the algorithm may be parallelized and the manner in which it applies user-provided function objects.Rng
: The type of the source range used (deduced). The iterators extracted from this range type must meet the requirements of an input iterator.F
: The type of the function/function object to use (deduced). Unlike its sequential form, the parallel overload of none_of requires F to meet the requirements of CopyConstructible.Proj
: The type of an optional projection function. This defaults to util::projection_identity
- Parameters
policy
: The execution policy to use for the scheduling of the iterations.rng
: Refers to the sequence of elements the algorithm will be applied to.f
: Specifies the function (or function object) which will be invoked for each of the elements in the sequence specified by [first, last). The signature of this predicate should be equivalent to:bool pred(const Type &a);
The signature does not need to have const&, but the function must not modify the objects passed to it. The type
Type must be such that an object of type FwdIter can be dereferenced and then implicitly converted to Type.proj
: Specifies the function (or function object) which will be invoked for each of the elements as a projection operation before the actual predicate is invoked.
The application of function objects in parallel algorithm invoked with an execution policy object of type parallel_policy or parallel_task_policy are permitted to execute in an unordered fashion in unspecified threads, and indeterminately sequenced within each thread.
- Return
- The all_of algorithm returns a hpx::future<bool> if the execution policy is of type sequenced_task_policy or parallel_task_policy and returns bool otherwise. The all_of algorithm returns true if the unary predicate f returns true for all elements in the range, false otherwise. It returns true if the range is empty.
-
template<typename
ExPolicy
, typenameRng
, typenameOutIter
>
util::detail::algorithm_result<ExPolicy, hpx::util::tagged_pair<tag::in(typename hpx::traits::range_traits<Rng>::iterator_type), tag::out(OutIter)>>::typecopy
(ExPolicy &&policy, Rng &&rng, OutIter dest)¶ Copies the elements in the range rng to another range beginning at dest.
The assignments in the parallel
copy algorithm invoked with an execution policy object of type sequenced_policy execute in sequential order in the calling thread.- Note
- Complexity: Performs exactly std::distance(begin(rng), end(rng)) assignments.
- Template Parameters
ExPolicy
: The type of the execution policy to use (deduced). It describes the manner in which the execution of the algorithm may be parallelized and the manner in which it executes the assignments.Rng
: The type of the source range used (deduced). The iterators extracted from this range type must meet the requirements of an input iterator.OutIter
: The type of the iterator representing the destination range (deduced). This iterator type must meet the requirements of an output iterator.
- Parameters
policy
: The execution policy to use for the scheduling of the iterations.rng
: Refers to the sequence of elements the algorithm will be applied to.dest
: Refers to the beginning of the destination range.
The assignments in the parallel copy algorithm invoked with an execution policy object of type parallel_policy or parallel_task_policy are permitted to execute in an unordered fashion in unspecified threads, and indeterminately sequenced within each thread.
- Return
- The copy algorithm returns a hpx::future<tagged_pair<tag::in(iterator_t<Rng>), tag::out(FwdIter2)> > if the execution policy is of type sequenced_task_policy or parallel_task_policy and returns tagged_pair<tag::in(iterator_t<Rng>), tag::out(FwdIter2)> otherwise. The copy algorithm returns the pair of the input iterator last and the output iterator to the element in the destination range, one past the last element copied.
-
template<typename
ExPolicy
, typenameRng
, typenameOutIter
, typenameF
, typenameProj
= util::projection_identity>
util::detail::algorithm_result<ExPolicy, hpx::util::tagged_pair<tag::in(typename hpx::traits::range_traits<Rng>::iterator_type), tag::out(OutIter)>>::typecopy_if
(ExPolicy &&policy, Rng &&rng, OutIter dest, F &&f, Proj &&proj = Proj())¶ Copies the elements in the range rng to another range beginning at dest. Copies only the elements for which the predicate f returns true. The order of the elements that are not removed is preserved.
The assignments in the parallel
copy_if algorithm invoked with an execution policy object of type sequenced_policy execute in sequential order in the calling thread.- Note
- Complexity: Performs not more than std::distance(begin(rng), end(rng)) assignments, exactly std::distance(begin(rng), end(rng)) applications of the predicate f.
- Template Parameters
ExPolicy
: The type of the execution policy to use (deduced). It describes the manner in which the execution of the algorithm may be parallelized and the manner in which it executes the assignments.Rng
: The type of the source range used (deduced). The iterators extracted from this range type must meet the requirements of an input iterator.OutIter
: The type of the iterator representing the destination range (deduced). This iterator type must meet the requirements of an output iterator.F
: The type of the function/function object to use (deduced). Unlike its sequential form, the parallel overload of copy_if requires F to meet the requirements of CopyConstructible.Proj
: The type of an optional projection function. This defaults to util::projection_identity
- Parameters
policy
: The execution policy to use for the scheduling of the iterations.rng
: Refers to the sequence of elements the algorithm will be applied to.dest
: Refers to the beginning of the destination range.f
: Specifies the function (or function object) which will be invoked for each of the elements in the sequence specified by [first, last).This is an unary predicate which returns true for the required elements. The signature of this predicate should be equivalent to:bool pred(const Type &a);
The signature does not need to have const&, but the function must not modify the objects passed to it. The type
Type must be such that an object of type InIter can be dereferenced and then implicitly converted to Type.proj
: Specifies the function (or function object) which will be invoked for each of the elements as a projection operation before the actual predicate is invoked.
The assignments in the parallel copy_if algorithm invoked with an execution policy object of type parallel_policy or parallel_task_policy are permitted to execute in an unordered fashion in unspecified threads, and indeterminately sequenced within each thread.
- Return
- The copy_if algorithm returns a hpx::future<tagged_pair<tag::in(iterator_t<Rng>), tag::out(FwdIter2)> > if the execution policy is of type sequenced_task_policy or parallel_task_policy and returns tagged_pair<tag::in(iterator_t<Rng>), tag::out(FwdIter2)> otherwise. The copy_if algorithm returns the pair of the input iterator last and the output iterator to the element in the destination range, one past the last element copied.
-
template<typename
ExPolicy
, typenameRng
, typenameT
, typenameProj
= util::projection_identity>
util::detail::algorithm_result<ExPolicy, typename std::iterator_traits<typename hpx::traits::range_traits<Rng>::iterator_type>::difference_type>::typecount
(ExPolicy &&policy, Rng &&rng, T const &value, Proj &&proj = Proj())¶ Returns the number of elements in the range [first, last) satisfying a specific criteria. This version counts the elements that are equal to the given value.
The comparisons in the parallel
count algorithm invoked with an execution policy object of type sequenced_policy execute in sequential order in the calling thread.- Note
- Complexity: Performs exactly last - first comparisons.
- Template Parameters
ExPolicy
: The type of the execution policy to use (deduced). It describes the manner in which the execution of the algorithm may be parallelized and the manner in which it executes the comparisons.Rng
: The type of the source range used (deduced). The iterators extracted from this range type must meet the requirements of an input iterator.T
: The type of the value to search for (deduced).Proj
: The type of an optional projection function. This defaults to util::projection_identity
- Parameters
policy
: The execution policy to use for the scheduling of the iterations.rng
: Refers to the sequence of elements the algorithm will be applied to.value
: The value to search for.proj
: Specifies the function (or function object) which will be invoked for each of the elements as a projection operation before the actual predicate is invoked.
- Note
- The comparisons in the parallel count algorithm invoked with an execution policy object of type parallel_policy or parallel_task_policy are permitted to execute in an unordered fashion in unspecified threads, and indeterminately sequenced within each thread.
- Return
- The count algorithm returns a hpx::future<difference_type> if the execution policy is of type sequenced_task_policy or parallel_task_policy and returns difference_type otherwise (where difference_type is defined by std::iterator_traits<FwdIter>::difference_type. The count algorithm returns the number of elements satisfying the given criteria.
-
template<typename
ExPolicy
, typenameRng
, typenameF
, typenameProj
= util::projection_identity>
util::detail::algorithm_result<ExPolicy, typename std::iterator_traits<typename hpx::traits::range_traits<Rng>::iterator_type>::difference_type>::typecount_if
(ExPolicy &&policy, Rng &&rng, F &&f, Proj &&proj = Proj())¶ Returns the number of elements in the range [first, last) satisfying a specific criteria. This version counts elements for which predicate f returns true.
- Note
- Complexity: Performs exactly last - first applications of the predicate.
- Note
- The assignments in the parallel count_if algorithm invoked with an execution policy object of type sequenced_policy execute in sequential order in the calling thread.
- Note
- The assignments in the parallel count_if algorithm invoked with an execution policy object of type parallel_policy or parallel_task_policy are permitted to execute in an unordered fashion in unspecified threads, and indeterminately sequenced within each thread.
- Return
- The count_if algorithm returns hpx::future<difference_type> if the execution policy is of type sequenced_task_policy or parallel_task_policy and returns difference_type otherwise (where difference_type is defined by std::iterator_traits<FwdIter>::difference_type. The count algorithm returns the number of elements satisfying the given criteria.
- Template Parameters
ExPolicy
: The type of the execution policy to use (deduced). It describes the manner in which the execution of the algorithm may be parallelized and the manner in which it executes the comparisons.Rng
: The type of the source range used (deduced). The iterators extracted from this range type must meet the requirements of an input iterator.F
: The type of the function/function object to use (deduced). Unlike its sequential form, the parallel overload of count_if requires F to meet the requirements of CopyConstructible.Proj
: The type of an optional projection function. This defaults to util::projection_identity
- Parameters
policy
: The execution policy to use for the scheduling of the iterations.rng
: Refers to the sequence of elements the algorithm will be applied to.f
: Specifies the function (or function object) which will be invoked for each of the elements in the sequence specified by [first, last).This is an unary predicate which returns true for the required elements. The signature of this predicate should be equivalent to:bool pred(const Type &a);
The signature does not need to have const&, but the function must not modify the objects passed to it. The type
Type must be such that an object of type FwdIter can be dereferenced and then implicitly converted to Type.proj
: Specifies the function (or function object) which will be invoked for each of the elements as a projection operation before the actual predicate is invoked.
-
template<typename
ExPolicy
, typenameRng
, typenameT
>
util::detail::algorithm_result<ExPolicy>::typefill
(ExPolicy &&policy, Rng &&rng, T value)¶ Assigns the given value to the elements in the range [first, last).
The comparisons in the parallel
fill algorithm invoked with an execution policy object of type sequenced_policy execute in sequential order in the calling thread.- Note
- Complexity: Performs exactly last - first assignments.
- Template Parameters
ExPolicy
: The type of the execution policy to use (deduced). It describes the manner in which the execution of the algorithm may be parallelized and the manner in which it executes the assignments.Rng
: The type of the source range used (deduced). The iterators extracted from this range type must meet the requirements of an input iterator.T
: The type of the value to be assigned (deduced).
- Parameters
policy
: The execution policy to use for the scheduling of the iterations.rng
: Refers to the sequence of elements the algorithm will be applied to.value
: The value to be assigned.
The comparisons in the parallel fill algorithm invoked with an execution policy object of type parallel_policy or parallel_task_policy are permitted to execute in an unordered fashion in unspecified threads, and indeterminately sequenced within each thread.
- Return
- The fill algorithm returns a hpx::future<void> if the execution policy is of type sequenced_task_policy or parallel_task_policy and returns difference_type otherwise (where difference_type is defined by void.
-
template<typename
ExPolicy
, typenameRng
, typenameSize
, typenameT
>
util::detail::algorithm_result<ExPolicy, typename hpx::traits::range_traits<Rng>::iterator_type>::typefill_n
(ExPolicy &&policy, Rng &rng, Size count, T value)¶ Assigns the given value value to the first count elements in the range beginning at first if count > 0. Does nothing otherwise.
The comparisons in the parallel
fill_n algorithm invoked with an execution policy object of type sequenced_policy execute in sequential order in the calling thread.- Note
- Complexity: Performs exactly count assignments, for count > 0.
- Template Parameters
ExPolicy
: The type of the execution policy to use (deduced). It describes the manner in which the execution of the algorithm may be parallelized and the manner in which it executes the assignments.Rng
: The type of the source range used (deduced). The iterators extracted from this range type must meet the requirements of an input iterator.Size
: The type of the argument specifying the number of elements to apply f to.T
: The type of the value to be assigned (deduced).
- Parameters
policy
: The execution policy to use for the scheduling of the iterations.rng
: Refers to the sequence of elements the algorithm will be applied to.count
: Refers to the number of elements starting at first the algorithm will be applied to.value
: The value to be assigned.
The comparisons in the parallel fill_n algorithm invoked with an execution policy object of type parallel_policy or parallel_task_policy are permitted to execute in an unordered fashion in unspecified threads, and indeterminately sequenced within each thread.
- Return
- The fill_n algorithm returns a hpx::future<void> if the execution policy is of type sequenced_task_policy or parallel_task_policy and returns difference_type otherwise (where difference_type is defined by void.
-
template<typename
ExPolicy
, typenameRng
, typenameRng2
, typenamePred
= detail::equal_to, typenameProj
= util::projection_identity>
util::detail::algorithm_result<ExPolicy, typename hpx::traits::range_iterator<Rng>::type>::typefind_end
(ExPolicy &&policy, Rng &&rng, Rng2 &&rng2, Pred &&op = Pred(), Proj &&proj = Proj())¶ Returns the last subsequence of elements rng2 found in the range rng using the given predicate f to compare elements.
The comparison operations in the parallel
find_end algorithm invoked with an execution policy object of type sequenced_policy execute in sequential order in the calling thread.- Note
- Complexity: at most S*(N-S+1) comparisons where S = distance(begin(rng2), end(rng2)) and N = distance(begin(rng), end(rng)).
- Template Parameters
ExPolicy
: The type of the execution policy to use (deduced). It describes the manner in which the execution of the algorithm may be parallelized and the manner in which it executes the assignments.Rng
: The type of the first source range (deduced). The iterators extracted from this range type must meet the requirements of a forward iterator.Rng2
: The type of the second source range (deduced). The iterators extracted from this range type must meet the requirements of a forward iterator.Pred
: The type of an optional function/function object to use. Unlike its sequential form, the parallel overload of replace requires Pred to meet the requirements of CopyConstructible. This defaults to std::equal_to<>Proj
: The type of an optional projection function. This defaults to util::projection_identity
- Parameters
policy
: The execution policy to use for the scheduling of the iterations.rng
: Refers to the first sequence of elements the algorithm will be applied to.rng2
: Refers to the second sequence of elements the algorithm will be applied to.op
: The binary predicate which returns true if the elements should be treated as equal. The signature should be equivalent to the following:bool pred(const Type1 &a, const Type2 &b);
The signature does not need to have const &, but the function must not modify the objects passed to it. The types
Type1 and Type2 must be such that objects of types iterator_t<Rng> and iterator_t<Rng2> can be dereferenced and then implicitly converted to Type1 and Type2 respectively.proj
: Specifies the function (or function object) which will be invoked for each of the elements of type dereferenced iterator_t<Rng> and dereferenced iterator_t<Rng2> as a projection operation before the function op is invoked.
The comparison operations in the parallel find_end algorithm invoked with an execution policy object of type parallel_policy or parallel_task_policy are permitted to execute in an unordered fashion in unspecified threads, and indeterminately sequenced within each thread.
This overload of
find_end is available if the user decides to provide the algorithm their own predicate op.- Return
- The find_end algorithm returns a hpx::future<iterator_t<Rng> > if the execution policy is of type sequenced_task_policy or parallel_task_policy and returns iterator_t<Rng> otherwise. The find_end algorithm returns an iterator to the beginning of the last subsequence rng2 in range rng. If the length of the subsequence rng2 is greater than the length of the range rng, end(rng) is returned. Additionally if the size of the subsequence is empty or no subsequence is found, end(rng) is also returned.
-
template<typename
ExPolicy
, typenameRng1
, typenameRng2
, typenamePred
= detail::equal_to, typenameProj1
= util::projection_identity, typenameProj2
= util::projection_identity>
util::detail::algorithm_result<ExPolicy, typename hpx::traits::range_iterator<Rng1>::type>::typefind_first_of
(ExPolicy &&policy, Rng1 &&rng1, Rng2 &&rng2, Pred &&op = Pred(), Proj1 &&proj1 = Proj1(), Proj2 &&proj2 = Proj2())¶ Searches the range rng1 for any elements in the range rng2. Uses binary predicate p to compare elements
The comparison operations in the parallel
find_first_of algorithm invoked with an execution policy object of type sequenced_policy execute in sequential order in the calling thread.- Note
- Complexity: at most (S*N) comparisons where S = distance(begin(rng2), end(rng2)) and N = distance(begin(rng1), end(rng1)).
- Template Parameters
ExPolicy
: The type of the execution policy to use (deduced). It describes the manner in which the execution of the algorithm may be parallelized and the manner in which it executes the assignments.Rng1
: The type of the first source range (deduced). The iterators extracted from this range type must meet the requirements of a forward iterator.Rng2
: The type of the second source range (deduced). The iterators extracted from this range type must meet the requirements of a forward iterator.Pred
: The type of an optional function/function object to use. Unlike its sequential form, the parallel overload of replace requires Pred to meet the requirements of CopyConstructible. This defaults to std::equal_to<>Proj1
: The type of an optional projection function. This defaults to util::projection_identity and is applied to the elements in rng1.Proj2
: The type of an optional projection function. This defaults to util::projection_identity and is applied to the elements in rng2.
- Parameters
policy
: The execution policy to use for the scheduling of the iterations.rng1
: Refers to the first sequence of elements the algorithm will be applied to.rng2
: Refers to the second sequence of elements the algorithm will be applied to.op
: The binary predicate which returns true if the elements should be treated as equal. The signature should be equivalent to the following:bool pred(const Type1 &a, const Type2 &b);
The signature does not need to have const &, but the function must not modify the objects passed to it. The types
Type1 and Type2 must be such that objects of types iterator_t<Rng1> and iterator_t<Rng2> can be dereferenced and then implicitly converted to Type1 and Type2 respectively.proj1
: Specifies the function (or function object) which will be invoked for each of the elements of type dereferenced iterator_t<Rng1> before the function op is invoked.proj2
: Specifies the function (or function object) which will be invoked for each of the elements of type dereferenced iterator_t<Rng2> before the function op is invoked.
The comparison operations in the parallel find_first_of algorithm invoked with an execution policy object of type parallel_policy or parallel_task_policy are permitted to execute in an unordered fashion in unspecified threads, and indeterminately sequenced within each thread.
This overload of
find_first_of is available if the user decides to provide the algorithm their own predicate op.- Return
- The find_end algorithm returns a hpx::future<iterator_t<Rng1> > if the execution policy is of type sequenced_task_policy or parallel_task_policy and returns iterator_t<Rng1> otherwise. The find_first_of algorithm returns an iterator to the first element in the range rng1 that is equal to an element from the range rng2. If the length of the subsequence rng2 is greater than the length of the range rng1, end(rng1) is returned. Additionally if the size of the subsequence is empty or no subsequence is found, end(rng1) is also returned.
-
template<typename
ExPolicy
, typenameRng
, typenameF
, typenameProj
= util::projection_identity>
util::detail::algorithm_result<ExPolicy, typename hpx::traits::range_iterator<Rng>::type>::typefor_each
(ExPolicy &&policy, Rng &&rng, F &&f, Proj &&proj = Proj())¶ Applies f to the result of dereferencing every iterator in the given range rng.
If
f returns a result, the result is ignored.- Note
- Complexity: Applies f exactly size(rng) times.
If the type of first satisfies the requirements of a mutable iterator, f may apply non-constant functions through the dereferenced iterator.
Unlike its sequential form, the parallel overload of for_each does not return a copy of its Function parameter, since parallelization may not permit efficient state accumulation.
The application of function objects in parallel algorithm invoked with an execution policy object of type
sequenced_policy execute in sequential order in the calling thread.- Template Parameters
ExPolicy
: The type of the execution policy to use (deduced). It describes the manner in which the execution of the algorithm may be parallelized and the manner in which it applies user-provided function objects.Rng
: The type of the source range used (deduced). The iterators extracted from this range type must meet the requirements of an input iterator.F
: The type of the function/function object to use (deduced). Unlike its sequential form, the parallel overload of for_each requires F to meet the requirements of CopyConstructible.Proj
: The type of an optional projection function. This defaults to util::projection_identity
- Parameters
policy
: The execution policy to use for the scheduling of the iterations.rng
: Refers to the sequence of elements the algorithm will be applied to.f
: Specifies the function (or function object) which will be invoked for each of the elements in the sequence specified by [first, last). The signature of this predicate should be equivalent to:<ignored> pred(const Type &a);
The signature does not need to have const&. The type
Type must be such that an object of type InIter can be dereferenced and then implicitly converted to Type.proj
: Specifies the function (or function object) which will be invoked for each of the elements as a projection operation before the actual predicate is invoked.
The application of function objects in parallel algorithm invoked with an execution policy object of type parallel_policy or parallel_task_policy are permitted to execute in an unordered fashion in unspecified threads, and indeterminately sequenced within each thread.
- Return
- The for_each algorithm returns a hpx::future<InIter> if the execution policy is of type sequenced_task_policy or parallel_task_policy and returns InIter otherwise. It returns last.
-
template<typename
ExPolicy
, typenameRng
, typenameF
>
util::detail::algorithm_result<ExPolicy, typename hpx::traits::range_iterator<Rng>::type>::typegenerate
(ExPolicy &&policy, Rng &&rng, F &&f)¶ Assign each element in range [first, last) a value generated by the given function object f
The assignments in the parallel
generate algorithm invoked with an execution policy object of type sequenced_policy execute in sequential order in the calling thread.- Note
- Complexity: Exactly distance(first, last) invocations of f and assignments.
- Template Parameters
ExPolicy
: The type of the execution policy to use (deduced). It describes the manner in which the execution of the algorithm may be parallelized and the manner in which it executes the assignments.Rng
: The type of the source range used (deduced). The iterators extracted from this range type must meet the requirements of an forward iterator.F
: The type of the function/function object to use (deduced). Unlike its sequential form, the parallel overload of equal requires F to meet the requirements of CopyConstructible.
- Parameters
policy
: The execution policy to use for the scheduling of the iterations.rng
: Refers to the sequence of elements the algorithm will be applied to.f
: generator function that will be called. signature of function should be equivalent to the following:Ret fun();
The type
Ret must be such that an object of type FwdIter can be dereferenced and assigned a value of type Ret.
The assignments in the parallel generate algorithm invoked with an execution policy object of type parallel_policy or parallel_task_policy are permitted to execute in an unordered fashion in unspecified threads, and indeterminately sequenced within each thread.
- Return
- The replace_if algorithm returns a hpx::future<FwdIter> if the execution policy is of type sequenced_task_policy or parallel_task_policy and returns FwdIter otherwise. It returns last.
-
template<typename
ExPolicy
, typenameRng
, typenameComp
= detail::less, typenameProj
= util::projection_identity>
util::detail::algorithm_result<ExPolicy, bool>::typeis_heap
(ExPolicy &&policy, Rng &&rng, Comp &&comp = Comp(), Proj &&proj = Proj())¶ Returns whether the range is max heap. That is, true if the range is max heap, false otherwise. The function uses the given comparison function object comp (defaults to using operator<()).
comp has to induce a strict weak ordering on the values.
- Note
- Complexity: Performs at most N applications of the comparison comp, at most 2 * N applications of the projection proj, where N = last - first.
- Template Parameters
ExPolicy
: The type of the execution policy to use (deduced). It describes the manner in which the execution of the algorithm may be parallelized and the manner in which it executes the assignments.Rng
: The type of the source range used (deduced). The iterators extracted from this range type must meet the requirements of an random access iterator.Comp
: The type of the function/function object to use (deduced).Proj
: The type of an optional projection function. This defaults to util::projection_identity
- Parameters
policy
: The execution policy to use for the scheduling of the iterations.rng
: Refers to the sequence of elements the algorithm will be applied to.comp
: comp is a callable object. The return value of the INVOKE operation applied to an object of type Comp, when contextually converted to bool, yields true if the first argument of the call is less than the second, and false otherwise. It is assumed that comp will not apply any non-constant function through the dereferenced iterator.proj
: Specifies the function (or function object) which will be invoked for each of the elements as a projection operation before the actual predicate is invoked.
The application of function objects in parallel algorithm invoked with an execution policy object of type sequenced_policy execute in sequential order in the calling thread.
The application of function objects in parallel algorithm invoked with an execution policy object of type parallel_policy or parallel_task_policy are permitted to execute in an unordered fashion in unspecified threads, and indeterminately sequenced within each thread.
- Return
- The is_heap algorithm returns a hpx::future<bool> if the execution policy is of type sequenced_task_policy or parallel_task_policy and returns bool otherwise. The is_heap algorithm returns whether the range is max heap. That is, true if the range is max heap, false otherwise.
-
template<typename
ExPolicy
, typenameRng
, typenameComp
= detail::less, typenameProj
= util::projection_identity>
util::detail::algorithm_result<ExPolicy, typename hpx::traits::range_iterator<Rng>::type>::typeis_heap_until
(ExPolicy &&policy, Rng &&rng, Comp &&comp = Comp(), Proj &&proj = Proj())¶ Returns the upper bound of the largest range beginning at first which is a max heap. That is, the last iterator it for which range [first, it) is a max heap. The function uses the given comparison function object comp (defaults to using operator<()).
comp has to induce a strict weak ordering on the values.
- Note
- Complexity: Performs at most N applications of the comparison comp, at most 2 * N applications of the projection proj, where N = last - first.
- Template Parameters
ExPolicy
: The type of the execution policy to use (deduced). It describes the manner in which the execution of the algorithm may be parallelized and the manner in which it executes the assignments.Rng
: The type of the source range used (deduced). The iterators extracted from this range type must meet the requirements of an random access iterator.Comp
: The type of the function/function object to use (deduced).Proj
: The type of an optional projection function. This defaults to util::projection_identity
- Parameters
policy
: The execution policy to use for the scheduling of the iterations.rng
: Refers to the sequence of elements the algorithm will be applied to.comp
: comp is a callable object. The return value of the INVOKE operation applied to an object of type Comp, when contextually converted to bool, yields true if the first argument of the call is less than the second, and false otherwise. It is assumed that comp will not apply any non-constant function through the dereferenced iterator.proj
: Specifies the function (or function object) which will be invoked for each of the elements as a projection operation before the actual predicate is invoked.
The application of function objects in parallel algorithm invoked with an execution policy object of type sequenced_policy execute in sequential order in the calling thread.
The application of function objects in parallel algorithm invoked with an execution policy object of type parallel_policy or parallel_task_policy are permitted to execute in an unordered fashion in unspecified threads, and indeterminately sequenced within each thread.
- Return
- The is_heap_until algorithm returns a hpx::future<RandIter> if the execution policy is of type sequenced_task_policy or parallel_task_policy and returns RandIter otherwise. The is_heap_until algorithm returns the upper bound of the largest range beginning at first which is a max heap. That is, the last iterator it for which range [first, it) is a max heap.
-
template<typename
ExPolicy
, typenameRng1
, typenameRng2
, typenameRandIter3
, typenameComp
= detail::less, typenameProj1
= util::projection_identity, typenameProj2
= util::projection_identity>
util::detail::algorithm_result<ExPolicy, hpx::util::tagged_tuple<tag::in1(typename hpx::traits::range_iterator<Rng1>::type), tag::in2(typename hpx::traits::range_iterator<Rng2>::type), tag::out(RandIter3)>>::typemerge
(ExPolicy &&policy, Rng1 &&rng1, Rng2 &&rng2, RandIter3 dest, Comp &&comp = Comp(), Proj1 &&proj1 = Proj1(), Proj2 &&proj2 = Proj2())¶ Merges two sorted ranges [first1, last1) and [first2, last2) into one sorted range beginning at dest. The order of equivalent elements in the each of original two ranges is preserved. For equivalent elements in the original two ranges, the elements from the first range precede the elements from the second range. The destination range cannot overlap with either of the input ranges.
The assignments in the parallel
merge algorithm invoked with an execution policy object of type sequenced_policy execute in sequential order in the calling thread.- Note
- Complexity: Performs O(std::distance(first1, last1) + std::distance(first2, last2)) applications of the comparison comp and the each projection.
- Template Parameters
ExPolicy
: The type of the execution policy to use (deduced). It describes the manner in which the execution of the algorithm may be parallelized and the manner in which it executes the assignments.Rng1
: The type of the first source range used (deduced). The iterators extracted from this range type must meet the requirements of an random access iterator.Rng2
: The type of the second source range used (deduced). The iterators extracted from this range type must meet the requirements of an random access iterator.RandIter3
: The type of the iterator representing the destination range (deduced). This iterator type must meet the requirements of an random access iterator.Comp
: The type of the function/function object to use (deduced). Unlike its sequential form, the parallel overload of merge requires Comp to meet the requirements of CopyConstructible. This defaults to std::less<>Proj1
: The type of an optional projection function to be used for elements of the first range. This defaults to util::projection_identityProj2
: The type of an optional projection function to be used for elements of the second range. This defaults to util::projection_identity
- Parameters
policy
: The execution policy to use for the scheduling of the iterations.rng1
: Refers to the first range of elements the algorithm will be applied to.rng2
: Refers to the second range of elements the algorithm will be applied to.dest
: Refers to the beginning of the destination range.comp
: comp is a callable object which returns true if the first argument is less than the second, and false otherwise. The signature of this comparison should be equivalent to:bool comp(const Type1 &a, const Type2 &b);
The signature does not need to have const&, but the function must not modify the objects passed to it. The types
Type1 and Type2 must be such that objects of types RandIter1 and RandIter2 can be dereferenced and then implicitly converted to both Type1 and Type2proj1
: Specifies the function (or function object) which will be invoked for each of the elements of the first range as a projection operation before the actual comparison comp is invoked.proj2
: Specifies the function (or function object) which will be invoked for each of the elements of the second range as a projection operation before the actual comparison comp is invoked.
The assignments in the parallel merge algorithm invoked with an execution policy object of type parallel_policy or parallel_task_policy are permitted to execute in an unordered fashion in unspecified threads, and indeterminately sequenced within each thread.
- Return
- The merge algorithm returns a hpx::future<tagged_tuple<tag::in1(RandIter1), tag::in2(RandIter2), tag::out(RandIter3)> > if the execution policy is of type sequenced_task_policy or parallel_task_policy and returns tagged_tuple<tag::in1(RandIter1), tag::in2(RandIter2), tag::out(RandIter3)> otherwise. The merge algorithm returns the tuple of the source iterator last1, the source iterator last2, the destination iterator to the end of the dest range.
-
template<typename
ExPolicy
, typenameRng
, typenameRandIter
, typenameComp
= detail::less, typenameProj
= util::projection_identity>
util::detail::algorithm_result<ExPolicy, RandIter>::typeinplace_merge
(ExPolicy &&policy, Rng &&rng, RandIter middle, Comp &&comp = Comp(), Proj &&proj = Proj())¶ Merges two consecutive sorted ranges [first, middle) and [middle, last) into one sorted range [first, last). The order of equivalent elements in the each of original two ranges is preserved. For equivalent elements in the original two ranges, the elements from the first range precede the elements from the second range.
The assignments in the parallel
inplace_merge algorithm invoked with an execution policy object of type sequenced_policy execute in sequential order in the calling thread.- Note
- Complexity: Performs O(std::distance(first, last)) applications of the comparison comp and the each projection.
- Template Parameters
ExPolicy
: The type of the execution policy to use (deduced). It describes the manner in which the execution of the algorithm may be parallelized and the manner in which it executes the assignments.Rng
: The type of the source range used (deduced). The iterators extracted from this range type must meet the requirements of an random access iterator.RandIter
: The type of the source iterators used (deduced). This iterator type must meet the requirements of an random access iterator.Comp
: The type of the function/function object to use (deduced). Unlike its sequential form, the parallel overload of inplace_merge requires Comp to meet the requirements of CopyConstructible. This defaults to std::less<>Proj
: The type of an optional projection function. This defaults to util::projection_identity
- Parameters
policy
: The execution policy to use for the scheduling of the iterations.rng
: Refers to the range of elements the algorithm will be applied to.middle
: Refers to the end of the first sorted range and the beginning of the second sorted range the algorithm will be applied to.comp
: comp is a callable object which returns true if the first argument is less than the second, and false otherwise. The signature of this comparison should be equivalent to:bool comp(const Type1 &a, const Type2 &b);
The signature does not need to have const&, but the function must not modify the objects passed to it. The types
Type1 and Type2 must be such that objects of types RandIter can be dereferenced and then implicitly converted to both Type1 and Type2proj
: Specifies the function (or function object) which will be invoked for each of the elements as a projection operation before the actual predicate is invoked.
The assignments in the parallel inplace_merge algorithm invoked with an execution policy object of type parallel_policy or parallel_task_policy are permitted to execute in an unordered fashion in unspecified threads, and indeterminately sequenced within each thread.
- Return
- The inplace_merge algorithm returns a hpx::future<RandIter> if the execution policy is of type sequenced_task_policy or parallel_task_policy and returns RandIter otherwise. The inplace_merge algorithm returns the source iterator last
-
template<typename
ExPolicy
, typenameRng
, typenameProj
= util::projection_identity, typenameF
= detail::less>
util::detail::algorithm_result<ExPolicy, typename hpx::traits::range_traits<Rng>::iterator_type>::typemin_element
(ExPolicy &&policy, Rng &&rng, F &&f = F(), Proj &&proj = Proj())¶ Finds the smallest element in the range [first, last) using the given comparison function f.
The comparisons in the parallel
min_element algorithm invoked with an execution policy object of type sequenced_policy execute in sequential order in the calling thread.- Note
- Complexity: Exactly max(N-1, 0) comparisons, where N = std::distance(first, last).
- Template Parameters
ExPolicy
: The type of the execution policy to use (deduced). It describes the manner in which the execution of the algorithm may be parallelized and the manner in which it executes the assignments.Rng
: The type of the source range used (deduced). The iterators extracted from this range type must meet the requirements of an forward iterator.F
: The type of the function/function object to use (deduced). Unlike its sequential form, the parallel overload of min_element requires F to meet the requirements of CopyConstructible.Proj
: The type of an optional projection function. This defaults to util::projection_identity
- Parameters
policy
: The execution policy to use for the scheduling of the iterations.rng
: Refers to the sequence of elements the algorithm will be applied to.f
: The binary predicate which returns true if the the left argument is less than the right element. The signature of the predicate function should be equivalent to the following:bool pred(const Type1 &a, const Type1 &b);
The signature does not need to have const &, but the function must not modify the objects passed to it. The type
Type1 must be such that objects of type FwdIter can be dereferenced and then implicitly converted to Type1.proj
: Specifies the function (or function object) which will be invoked for each of the elements as a projection operation before the actual predicate is invoked.
The comparisons in the parallel min_element algorithm invoked with an execution policy object of type parallel_policy or parallel_task_policy are permitted to execute in an unordered fashion in unspecified threads, and indeterminately sequenced within each thread.
- Return
- The min_element algorithm returns a hpx::future<FwdIter> if the execution policy is of type sequenced_task_policy or parallel_task_policy and returns FwdIter otherwise. The min_element algorithm returns the iterator to the smallest element in the range [first, last). If several elements in the range are equivalent to the smallest element, returns the iterator to the first such element. Returns last if the range is empty.
-
template<typename
ExPolicy
, typenameRng
, typenameProj
= util::projection_identity, typenameF
= detail::less>
util::detail::algorithm_result<ExPolicy, typename hpx::traits::range_traits<Rng>::iterator_type>::typemax_element
(ExPolicy &&policy, Rng &&rng, F &&f = F(), Proj &&proj = Proj())¶ Finds the greatest element in the range [first, last) using the given comparison function f.
The comparisons in the parallel
max_element algorithm invoked with an execution policy object of type sequenced_policy execute in sequential order in the calling thread.- Note
- Complexity: Exactly max(N-1, 0) comparisons, where N = std::distance(first, last).
- Template Parameters
ExPolicy
: The type of the execution policy to use (deduced). It describes the manner in which the execution of the algorithm may be parallelized and the manner in which it executes the assignments.Rng
: The type of the source range used (deduced). The iterators extracted from this range type must meet the requirements of an forward iterator.F
: The type of the function/function object to use (deduced). Unlike its sequential form, the parallel overload of max_element requires F to meet the requirements of CopyConstructible.Proj
: The type of an optional projection function. This defaults to util::projection_identity
- Parameters
policy
: The execution policy to use for the scheduling of the iterations.rng
: Refers to the sequence of elements the algorithm will be applied to.f
: The binary predicate which returns true if the This argument is optional and defaults to std::less. the left argument is less than the right element. The signature of the predicate function should be equivalent to the following:bool pred(const Type1 &a, const Type1 &b);
The signature does not need to have const &, but the function must not modify the objects passed to it. The type
Type1 must be such that objects of type FwdIter can be dereferenced and then implicitly converted to Type1.proj
: Specifies the function (or function object) which will be invoked for each of the elements as a projection operation before the actual predicate is invoked.
The comparisons in the parallel max_element algorithm invoked with an execution policy object of type parallel_policy or parallel_task_policy are permitted to execute in an unordered fashion in unspecified threads, and indeterminately sequenced within each thread.
- Return
- The max_element algorithm returns a hpx::future<FwdIter> if the execution policy is of type sequenced_task_policy or parallel_task_policy and returns FwdIter otherwise. The max_element algorithm returns the iterator to the smallest element in the range [first, last). If several elements in the range are equivalent to the smallest element, returns the iterator to the first such element. Returns last if the range is empty.
-
template<typename
ExPolicy
, typenameRng
, typenameProj
= util::projection_identity, typenameF
= detail::less>
util::detail::algorithm_result<ExPolicy, hpx::util::tagged_pair<tag::min(typename hpx::traits::range_traits<Rng>::iterator_type), tag::max(typename hpx::traits::range_traits<Rng>::iterator_type)>>::typeminmax_element
(ExPolicy &&policy, Rng &&rng, F &&f = F(), Proj &&proj = Proj())¶ Finds the greatest element in the range [first, last) using the given comparison function f.
The comparisons in the parallel
minmax_element algorithm invoked with an execution policy object of type sequenced_policy execute in sequential order in the calling thread.- Note
- Complexity: At most max(floor(3/2*(N-1)), 0) applications of the predicate, where N = std::distance(first, last).
- Template Parameters
ExPolicy
: The type of the execution policy to use (deduced). It describes the manner in which the execution of the algorithm may be parallelized and the manner in which it executes the assignments.Rng
: The type of the source range used (deduced). The iterators extracted from this range type must meet the requirements of an forward iterator.F
: The type of the function/function object to use (deduced). Unlike its sequential form, the parallel overload of minmax_element requires F to meet the requirements of CopyConstructible.Proj
: The type of an optional projection function. This defaults to util::projection_identity
- Parameters
policy
: The execution policy to use for the scheduling of the iterations.rng
: Refers to the sequence of elements the algorithm will be applied to.f
: The binary predicate which returns true if the the left argument is less than the right element. This argument is optional and defaults to std::less. The signature of the predicate function should be equivalent to the following:bool pred(const Type1 &a, const Type1 &b);
The signature does not need to have const &, but the function must not modify the objects passed to it. The type
Type1 must be such that objects of type FwdIter can be dereferenced and then implicitly converted to Type1.proj
: Specifies the function (or function object) which will be invoked for each of the elements as a projection operation before the actual predicate is invoked.
The comparisons in the parallel minmax_element algorithm invoked with an execution policy object of type parallel_policy or parallel_task_policy are permitted to execute in an unordered fashion in unspecified threads, and indeterminately sequenced within each thread.
- Return
- The minmax_element algorithm returns a hpx::future<tagged_pair<tag::min(FwdIter), tag::max(FwdIter)> > if the execution policy is of type sequenced_task_policy or parallel_task_policy and returns tagged_pair<tag::min(FwdIter), tag::max(FwdIter)> otherwise. The minmax_element algorithm returns a pair consisting of an iterator to the smallest element as the first element and an iterator to the greatest element as the second. Returns std::make_pair(first, first) if the range is empty. If several elements are equivalent to the smallest element, the iterator to the first such element is returned. If several elements are equivalent to the largest element, the iterator to the last such element is returned.
-
template<typename
ExPolicy
, typenameRng
, typenamePred
, typenameProj
= util::projection_identity>
util::detail::algorithm_result<ExPolicy, typename hpx::traits::range_iterator<Rng>::type>::typepartition
(ExPolicy &&policy, Rng &&rng, Pred &&pred, Proj &&proj = Proj())¶ Reorders the elements in the range rng in such a way that all elements for which the predicate pred returns true precede the elements for which the predicate pred returns false. Relative order of the elements is not preserved.
The assignments in the parallel
partition algorithm invoked with an execution policy object of type sequenced_policy execute in sequential order in the calling thread.- Note
- Complexity: Performs at most 2 * N swaps, exactly N applications of the predicate and projection, where N = std::distance(begin(rng), end(rng)).
- Template Parameters
ExPolicy
: The type of the execution policy to use (deduced). It describes the manner in which the execution of the algorithm may be parallelized and the manner in which it executes the assignments.Rng
: The type of the source range used (deduced). The iterators extracted from this range type must meet the requirements of an forward iterator.Pred
: The type of the function/function object to use (deduced). Unlike its sequential form, the parallel overload of partition requires Pred to meet the requirements of CopyConstructible.Proj
: The type of an optional projection function. This defaults to util::projection_identity
- Parameters
policy
: The execution policy to use for the scheduling of the iterations.rng
: Refers to the sequence of elements the algorithm will be applied to.pred
: Specifies the function (or function object) which will be invoked for each of the elements in the sequence specified by the range rng. This is an unary predicate for partitioning the source iterators. The signature of this predicate should be equivalent to:bool pred(const Type &a);
The signature does not need to have const&, but the function must not modify the objects passed to it. The type
Type must be such that an object of type FwdIter can be dereferenced and then implicitly converted to Type.proj
: Specifies the function (or function object) which will be invoked for each of the elements as a projection operation before the actual predicate is invoked.
The assignments in the parallel partition algorithm invoked with an execution policy object of type parallel_policy or parallel_task_policy are permitted to execute in an unordered fashion in unspecified threads, and indeterminately sequenced within each thread.
- Return
- The partition algorithm returns a hpx::future<FwdIter> if the execution policy is of type parallel_task_policy and returns FwdIter otherwise. The partition algorithm returns the iterator to the first element of the second group.
-
template<typename
ExPolicy
, typenameRng
, typenameFwdIter2
, typenameFwdIter3
, typenamePred
, typenameProj
= util::projection_identity>
util::detail::algorithm_result<ExPolicy, hpx::util::tagged_tuple<tag::in(typename hpx::traits::range_iterator<Rng>::type), tag::out1(FwdIter2), tag::out2(FwdIter3)>>::typepartition_copy
(ExPolicy &&policy, Rng &&rng, FwdIter2 dest_true, FwdIter3 dest_false, Pred &&pred, Proj &&proj = Proj())¶ Copies the elements in the range rng, to two different ranges depending on the value returned by the predicate pred. The elements, that satisfy the predicate pred, are copied to the range beginning at dest_true. The rest of the elements are copied to the range beginning at dest_false. The order of the elements is preserved.
The assignments in the parallel
partition_copy algorithm invoked with an execution policy object of type sequenced_policy execute in sequential order in the calling thread.- Note
- Complexity: Performs not more than N assignments, exactly N applications of the predicate pred, where N = std::distance(begin(rng), end(rng)).
- Template Parameters
ExPolicy
: The type of the execution policy to use (deduced). It describes the manner in which the execution of the algorithm may be parallelized and the manner in which it executes the assignments.Rng
: The type of the source range used (deduced). The iterators extracted from this range type must meet the requirements of an forward iterator.FwdIter2
: The type of the iterator representing the destination range for the elements that satisfy the predicate pred (deduced). This iterator type must meet the requirements of an forward iterator.FwdIter3
: The type of the iterator representing the destination range for the elements that don’t satisfy the predicate pred (deduced). This iterator type must meet the requirements of an forward iterator.Pred
: The type of the function/function object to use (deduced). Unlike its sequential form, the parallel overload of partition_copy requires Pred to meet the requirements of CopyConstructible.Proj
: The type of an optional projection function. This defaults to util::projection_identity
- Parameters
policy
: The execution policy to use for the scheduling of the iterations.rng
: Refers to the sequence of elements the algorithm will be applied to.dest_true
: Refers to the beginning of the destination range for the elements that satisfy the predicate pred.dest_false
: Refers to the beginning of the destination range for the elements that don’t satisfy the predicate pred.pred
: Specifies the function (or function object) which will be invoked for each of the elements in the sequence specified by the range rng. This is an unary predicate for partitioning the source iterators. The signature of this predicate should be equivalent to:bool pred(const Type &a);
The signature does not need to have const&, but the function must not modify the objects passed to it. The type
Type must be such that an object of type FwdIter1 can be dereferenced and then implicitly converted to Type.proj
: Specifies the function (or function object) which will be invoked for each of the elements as a projection operation before the actual predicate is invoked.
The assignments in the parallel partition_copy algorithm invoked with an execution policy object of type parallel_policy or parallel_task_policy are permitted to execute in an unordered fashion in unspecified threads, and indeterminately sequenced within each thread.
- Return
- The partition_copy algorithm returns a hpx::future<tagged_tuple<tag::in(InIter), tag::out1(OutIter1), tag::out2(OutIter2)> > if the execution policy is of type parallel_task_policy and returns tagged_tuple<tag::in(InIter), tag::out1(OutIter1), tag::out2(OutIter2)> otherwise. The partition_copy algorithm returns the tuple of the source iterator last, the destination iterator to the end of the dest_true range, and the destination iterator to the end of the dest_false range.
-
template<typename
ExPolicy
, typenameRng
, typenameT
, typenameProj
= util::projection_identity>
util::detail::algorithm_result<ExPolicy, typename hpx::traits::range_iterator<Rng>::type>::typeremove
(ExPolicy &&policy, Rng &&rng, T const &value, Proj &&proj = Proj())¶ Removes all elements satisfying specific criteria from the range [first, last) and returns a past-the-end iterator for the new end of the range. This version removes all elements that are equal to value.
The assignments in the parallel
remove algorithm invoked with an execution policy object of type sequenced_policy execute in sequential order in the calling thread.- Note
- Complexity: Performs not more than last - first assignments, exactly last - first applications of the operator==() and the projection proj.
- Template Parameters
ExPolicy
: The type of the execution policy to use (deduced). It describes the manner in which the execution of the algorithm may be parallelized and the manner in which it executes the assignments.Rng
: The type of the source range used (deduced). The iterators extracted from this range type must meet the requirements of an forward iterator.T
: The type of the value to remove (deduced). This value type must meet the requirements of CopyConstructible.Proj
: The type of an optional projection function. This defaults to util::projection_identity
- Parameters
policy
: The execution policy to use for the scheduling of the iterations.rng
: Refers to the sequence of elements the algorithm will be applied to.value
: Specifies the value of elements to remove.proj
: Specifies the function (or function object) which will be invoked for each of the elements as a projection operation before the actual predicate is invoked.
The assignments in the parallel remove algorithm invoked with an execution policy object of type parallel_policy or parallel_task_policy are permitted to execute in an unordered fashion in unspecified threads, and indeterminately sequenced within each thread.
- Return
- The remove algorithm returns a hpx::future<FwdIter> if the execution policy is of type sequenced_task_policy or parallel_task_policy and returns FwdIter otherwise. The remove algorithm returns the iterator to the new end of the range.
-
template<typename
ExPolicy
, typenameRng
, typenamePred
, typenameProj
= util::projection_identity>
util::detail::algorithm_result<ExPolicy, typename hpx::traits::range_iterator<Rng>::type>::typeremove_if
(ExPolicy &&policy, Rng &&rng, Pred &&pred, Proj &&proj = Proj())¶ Removes all elements satisfying specific criteria from the range [first, last) and returns a past-the-end iterator for the new end of the range. This version removes all elements for which predicate pred returns true.
The assignments in the parallel
remove_if algorithm invoked with an execution policy object of type sequenced_policy execute in sequential order in the calling thread.- Note
- Complexity: Performs not more than last - first assignments, exactly last - first applications of the predicate pred and the projection proj.
- Template Parameters
ExPolicy
: The type of the execution policy to use (deduced). It describes the manner in which the execution of the algorithm may be parallelized and the manner in which it executes the assignments.Rng
: The type of the source range used (deduced). The iterators extracted from this range type must meet the requirements of an forward iterator.Pred
: The type of the function/function object to use (deduced). Unlike its sequential form, the parallel overload of remove_if requires Pred to meet the requirements of CopyConstructible.Proj
: The type of an optional projection function. This defaults to util::projection_identity
- Parameters
policy
: The execution policy to use for the scheduling of the iterations.rng
: Refers to the sequence of elements the algorithm will be applied to.pred
: Specifies the function (or function object) which will be invoked for each of the elements in the sequence specified by [first, last).This is an unary predicate which returns true for the required elements. The signature of this predicate should be equivalent to:bool pred(const Type &a);
The signature does not need to have const&, but the function must not modify the objects passed to it. The type
Type must be such that an object of type FwdIter can be dereferenced and then implicitly converted to Type.proj
: Specifies the function (or function object) which will be invoked for each of the elements as a projection operation before the actual predicate is invoked.
The assignments in the parallel remove_if algorithm invoked with an execution policy object of type parallel_policy or parallel_task_policy are permitted to execute in an unordered fashion in unspecified threads, and indeterminately sequenced within each thread.
- Return
- The remove_if algorithm returns a hpx::future<FwdIter> if the execution policy is of type sequenced_task_policy or parallel_task_policy and returns FwdIter otherwise. The remove_if algorithm returns the iterator to the new end of the range.
-
template<typename
ExPolicy
, typenameRng
, typenameOutIter
, typenameT
, typenameProj
= util::projection_identity>
util::detail::algorithm_result<ExPolicy, hpx::util::tagged_pair<tag::in(typename hpx::traits::range_traits<Rng>::iterator_type), tag::out(OutIter)>>::typeremove_copy
(ExPolicy &&policy, Rng &&rng, OutIter dest, T const &val, Proj &&proj = Proj())¶ Copies the elements in the range, defined by [first, last), to another range beginning at dest. Copies only the elements for which the comparison operator returns false when compare to val. The order of the elements that are not removed is preserved.
Effects: Copies all the elements referred to by the iterator it in the range [first,last) for which the following corresponding conditions do not hold: INVOKE(proj, *it) == value
The assignments in the parallel
remove_copy algorithm invoked with an execution policy object of type sequenced_policy execute in sequential order in the calling thread.- Note
- Complexity: Performs not more than last - first assignments, exactly last - first applications of the predicate f.
- Template Parameters
ExPolicy
: The type of the execution policy to use (deduced). It describes the manner in which the execution of the algorithm may be parallelized and the manner in which it executes the assignments.Rng
: The type of the source range used (deduced). The iterators extracted from this range type must meet the requirements of an input iterator.OutIter
: The type of the iterator representing the destination range (deduced). This iterator type must meet the requirements of an output iterator.T
: The type that the result of dereferencing InIter is compared to.Proj
: The type of an optional projection function. This defaults to util::projection_identity
- Parameters
policy
: The execution policy to use for the scheduling of the iterations.rng
: Refers to the sequence of elements the algorithm will be applied to.dest
: Refers to the beginning of the destination range.val
: Value to be removed.proj
: Specifies the function (or function object) which will be invoked for each of the elements as a projection operation before the actual predicate is invoked.
The assignments in the parallel remove_copy algorithm invoked with an execution policy object of type parallel_policy or parallel_task_policy are permitted to execute in an unordered fashion in unspecified threads, and indeterminately sequenced within each thread.
- Return
- The remove_copy algorithm returns a hpx::future<tagged_pair<tag::in(InIter), tag::out(OutIter)> > if the execution policy is of type sequenced_task_policy or parallel_task_policy and returns tagged_pair<tag::in(InIter), tag::out(OutIter)> otherwise. The copy algorithm returns the pair of the input iterator forwarded to the first element after the last in the input sequence and the output iterator to the element in the destination range, one past the last element copied.
-
template<typename
ExPolicy
, typenameRng
, typenameOutIter
, typenameF
, typenameProj
= util::projection_identity>
util::detail::algorithm_result<ExPolicy, hpx::util::tagged_pair<tag::in(typename hpx::traits::range_traits<Rng>::iterator_type), tag::out(OutIter)>>::typeremove_copy_if
(ExPolicy &&policy, Rng &&rng, OutIter dest, F &&f, Proj &&proj = Proj())¶ Copies the elements in the range, defined by [first, last), to another range beginning at dest. Copies only the elements for which the predicate f returns false. The order of the elements that are not removed is preserved.
Effects: Copies all the elements referred to by the iterator it in the range [first,last) for which the following corresponding conditions do not hold: INVOKE(pred, INVOKE(proj, *it)) != false.
The assignments in the parallel
remove_copy_if algorithm invoked with an execution policy object of type sequenced_policy execute in sequential order in the calling thread.- Note
- Complexity: Performs not more than last - first assignments, exactly last - first applications of the predicate f.
- Template Parameters
ExPolicy
: The type of the execution policy to use (deduced). It describes the manner in which the execution of the algorithm may be parallelized and the manner in which it executes the assignments.Rng
: The type of the source range used (deduced). The iterators extracted from this range type must meet the requirements of an input iterator.OutIter
: The type of the iterator representing the destination range (deduced). This iterator type must meet the requirements of an output iterator.F
: The type of the function/function object to use (deduced). Unlike its sequential form, the parallel overload of copy_if requires F to meet the requirements of CopyConstructible.Proj
: The type of an optional projection function. This defaults to util::projection_identity
- Parameters
policy
: The execution policy to use for the scheduling of the iterations.rng
: Refers to the sequence of elements the algorithm will be applied to.dest
: Refers to the beginning of the destination range.f
: Specifies the function (or function object) which will be invoked for each of the elements in the sequence specified by [first, last).This is an unary predicate which returns true for the elements to be removed. The signature of this predicate should be equivalent to:bool pred(const Type &a);
The signature does not need to have const&, but the function must not modify the objects passed to it. The type
Type must be such that an object of type InIter can be dereferenced and then implicitly converted to Type.proj
: Specifies the function (or function object) which will be invoked for each of the elements as a projection operation before the actual predicate is invoked.
The assignments in the parallel remove_copy_if algorithm invoked with an execution policy object of type parallel_policy or parallel_task_policy are permitted to execute in an unordered fashion in unspecified threads, and indeterminately sequenced within each thread.
- Return
- The remove_copy_if algorithm returns a hpx::future<tagged_pair<tag::in(InIter), tag::out(OutIter)> > if the execution policy is of type sequenced_task_policy or parallel_task_policy and returns tagged_pair<tag::in(InIter), tag::out(OutIter)> otherwise. The copy algorithm returns the pair of the input iterator forwarded to the first element after the last in the input sequence and the output iterator to the element in the destination range, one past the last element copied.
-
template<typename
ExPolicy
, typenameRng
, typenameT1
, typenameT2
, typenameProj
= util::projection_identity>
util::detail::algorithm_result<ExPolicy, typename hpx::traits::range_traits<Rng>::iterator_type>::typereplace
(ExPolicy &&policy, Rng &&rng, T1 const &old_value, T2 const &new_value, Proj &&proj = Proj())¶ Replaces all elements satisfying specific criteria with new_value in the range [first, last).
Effects: Substitutes elements referred by the iterator it in the range [first,last) with new_value, when the following corresponding conditions hold: INVOKE(proj, *i) == old_value
- Note
- Complexity: Performs exactly last - first assignments.
The assignments in the parallel
replace algorithm invoked with an execution policy object of type sequenced_policy execute in sequential order in the calling thread.- Template Parameters
ExPolicy
: The type of the execution policy to use (deduced). It describes the manner in which the execution of the algorithm may be parallelized and the manner in which it executes the assignments.Rng
: The type of the source range used (deduced). The iterators extracted from this range type must meet the requirements of a forward iterator.T1
: The type of the old value to replace (deduced).T2
: The type of the new values to replace (deduced).Proj
: The type of an optional projection function. This defaults to util::projection_identity
- Parameters
policy
: The execution policy to use for the scheduling of the iterations.rng
: Refers to the sequence of elements the algorithm will be applied to.old_value
: Refers to the old value of the elements to replace.new_value
: Refers to the new value to use as the replacement.proj
: Specifies the function (or function object) which will be invoked for each of the elements as a projection operation before the actual predicate is invoked.
The assignments in the parallel replace algorithm invoked with an execution policy object of type parallel_policy or parallel_task_policy are permitted to execute in an unordered fashion in unspecified threads, and indeterminately sequenced within each thread.
- Return
- The replace algorithm returns a hpx::future<void> if the execution policy is of type sequenced_task_policy or parallel_task_policy and returns void otherwise.
-
template<typename
ExPolicy
, typenameRng
, typenameF
, typenameT
, typenameProj
= util::projection_identity>
util::detail::algorithm_result<ExPolicy, typename hpx::traits::range_traits<Rng>::iterator_type>::typereplace_if
(ExPolicy &&policy, Rng &&rng, F &&f, T const &new_value, Proj &&proj = Proj())¶ Replaces all elements satisfying specific criteria (for which predicate f returns true) with new_value in the range [first, last).
Effects: Substitutes elements referred by the iterator it in the range [first, last) with new_value, when the following corresponding conditions hold: INVOKE(f, INVOKE(proj, *it)) != false
- Note
- Complexity: Performs exactly last - first applications of the predicate.
The assignments in the parallel
replace_if algorithm invoked with an execution policy object of type sequenced_policy execute in sequential order in the calling thread.- Template Parameters
ExPolicy
: The type of the execution policy to use (deduced). It describes the manner in which the execution of the algorithm may be parallelized and the manner in which it executes the assignments.Rng
: The type of the source range used (deduced). The iterators extracted from this range type must meet the requirements of a forward iterator.F
: The type of the function/function object to use (deduced). Unlike its sequential form, the parallel overload of equal requires F to meet the requirements of CopyConstructible. (deduced).T
: The type of the new values to replace (deduced).Proj
: The type of an optional projection function. This defaults to util::projection_identity
- Parameters
policy
: The execution policy to use for the scheduling of the iterations.rng
: Refers to the sequence of elements the algorithm will be applied to.f
: Specifies the function (or function object) which will be invoked for each of the elements in the sequence specified by [first, last).This is an unary predicate which returns true for the elements which need to replaced. The signature of this predicate should be equivalent to:bool pred(const Type &a);
The signature does not need to have const&, but the function must not modify the objects passed to it. The type
Type must be such that an object of type FwdIter can be dereferenced and then implicitly converted to Type.new_value
: Refers to the new value to use as the replacement.proj
: Specifies the function (or function object) which will be invoked for each of the elements as a projection operation before the actual predicate is invoked.
The assignments in the parallel replace_if algorithm invoked with an execution policy object of type parallel_policy or parallel_task_policy are permitted to execute in an unordered fashion in unspecified threads, and indeterminately sequenced within each thread.
- Return
- The replace_if algorithm returns a hpx::future<FwdIter> if the execution policy is of type sequenced_task_policy or parallel_task_policy and returns void otherwise. It returns last.
-
template<typename
ExPolicy
, typenameRng
, typenameOutIter
, typenameT1
, typenameT2
, typenameProj
= util::projection_identity>
util::detail::algorithm_result<ExPolicy, hpx::util::tagged_pair<tag::in(typename hpx::traits::range_traits<Rng>::iterator_type), tag::out(OutIter)>>::typereplace_copy
(ExPolicy &&policy, Rng &&rng, OutIter dest, T1 const &old_value, T2 const &new_value, Proj &&proj = Proj())¶ Copies the all elements from the range [first, last) to another range beginning at dest replacing all elements satisfying a specific criteria with new_value.
Effects: Assigns to every iterator it in the range [result, result + (last - first)) either new_value or *(first + (it - result)) depending on whether the following corresponding condition holds: INVOKE(proj, *(first + (i - result))) == old_value
The assignments in the parallel
replace_copy algorithm invoked with an execution policy object of type sequenced_policy execute in sequential order in the calling thread.- Note
- Complexity: Performs exactly last - first applications of the predicate.
- Template Parameters
ExPolicy
: The type of the execution policy to use (deduced). It describes the manner in which the execution of the algorithm may be parallelized and the manner in which it executes the assignments.Rng
: The type of the source range used (deduced). The iterators extracted from this range type must meet the requirements of an input iterator.OutIter
: The type of the iterator representing the destination range (deduced). This iterator type must meet the requirements of an output iterator.T1
: The type of the old value to replace (deduced).T2
: The type of the new values to replace (deduced).Proj
: The type of an optional projection function. This defaults to util::projection_identity
- Parameters
policy
: The execution policy to use for the scheduling of the iterations.rng
: Refers to the sequence of elements the algorithm will be applied to.dest
: Refers to the beginning of the destination range.old_value
: Refers to the old value of the elements to replace.new_value
: Refers to the new value to use as the replacement.proj
: Specifies the function (or function object) which will be invoked for each of the elements as a projection operation before the actual predicate is invoked.
The assignments in the parallel replace_copy algorithm invoked with an execution policy object of type parallel_policy or parallel_task_policy are permitted to execute in an unordered fashion in unspecified threads, and indeterminately sequenced within each thread.
- Return
- The replace_copy algorithm returns a hpx::future<tagged_pair<tag::in(InIter), tag::out(OutIter)> > if the execution policy is of type sequenced_task_policy or parallel_task_policy and returns tagged_pair<tag::in(InIter), tag::out(OutIter)> otherwise. The copy algorithm returns the pair of the input iterator last and the output iterator to the element in the destination range, one past the last element copied.
-
template<typename
ExPolicy
, typenameRng
, typenameOutIter
, typenameF
, typenameT
, typenameProj
= util::projection_identity>
util::detail::algorithm_result<ExPolicy, hpx::util::tagged_pair<tag::in(typename hpx::traits::range_traits<Rng>::iterator_type), tag::out(OutIter)>>::typereplace_copy_if
(ExPolicy &&policy, Rng &&rng, OutIter dest, F &&f, T const &new_value, Proj &&proj = Proj())¶ Copies the all elements from the range [first, last) to another range beginning at dest replacing all elements satisfying a specific criteria with new_value.
Effects: Assigns to every iterator it in the range [result, result + (last - first)) either new_value or *(first + (it - result)) depending on whether the following corresponding condition holds: INVOKE(f, INVOKE(proj, *(first + (i - result)))) != false
The assignments in the parallel
replace_copy_if algorithm invoked with an execution policy object of type sequenced_policy execute in sequential order in the calling thread.- Note
- Complexity: Performs exactly last - first applications of the predicate.
- Template Parameters
ExPolicy
: The type of the execution policy to use (deduced). It describes the manner in which the execution of the algorithm may be parallelized and the manner in which it executes the assignments.Rng
: The type of the source range used (deduced). The iterators extracted from this range type must meet the requirements of an input iterator.OutIter
: The type of the iterator representing the destination range (deduced). This iterator type must meet the requirements of an output iterator.F
: The type of the function/function object to use (deduced). Unlike its sequential form, the parallel overload of equal requires F to meet the requirements of CopyConstructible. (deduced).T
: The type of the new values to replace (deduced).Proj
: The type of an optional projection function. This defaults to util::projection_identity
- Parameters
policy
: The execution policy to use for the scheduling of the iterations.rng
: Refers to the sequence of elements the algorithm will be applied to.dest
: Refers to the beginning of the destination range.f
: Specifies the function (or function object) which will be invoked for each of the elements in the sequence specified by [first, last).This is an unary predicate which returns true for the elements which need to replaced. The signature of this predicate should be equivalent to:bool pred(const Type &a);
The signature does not need to have const&, but the function must not modify the objects passed to it. The type
Type must be such that an object of type FwdIter can be dereferenced and then implicitly converted to Type.new_value
: Refers to the new value to use as the replacement.proj
: Specifies the function (or function object) which will be invoked for each of the elements as a projection operation before the actual predicate is invoked.
The assignments in the parallel replace_copy_if algorithm invoked with an execution policy object of type parallel_policy or parallel_task_policy are permitted to execute in an unordered fashion in unspecified threads, and indeterminately sequenced within each thread.
- Return
- The replace_copy_if algorithm returns a hpx::future<tagged_pair<tag::in(InIter), tag::out(OutIter)> > if the execution policy is of type sequenced_task_policy or parallel_task_policy and returns tagged_pair<tag::in(InIter), tag::out(OutIter)> otherwise. The replace_copy_if algorithm returns the input iterator last and the output iterator to the element in the destination range, one past the last element copied.
-
template<typename
ExPolicy
, typenameRng
>
util::detail::algorithm_result<ExPolicy, typename hpx::traits::range_iterator<Rng>::type>::typereverse
(ExPolicy &&policy, Rng &&rng)¶ Reverses the order of the elements in the range [first, last). Behaves as if applying std::iter_swap to every pair of iterators first+i, (last-i) - 1 for each non-negative i < (last-first)/2.
The assignments in the parallel
reverse algorithm invoked with an execution policy object of type sequenced_policy execute in sequential order in the calling thread.- Note
- Complexity: Linear in the distance between first and last.
- Template Parameters
ExPolicy
: The type of the execution policy to use (deduced). It describes the manner in which the execution of the algorithm may be parallelized and the manner in which it executes the assignments.Rng
: The type of the source range used (deduced). The iterators extracted from this range type must meet the requirements of a bidirectional iterator.
- Parameters
policy
: The execution policy to use for the scheduling of the iterations.rng
: Refers to the sequence of elements the algorithm will be applied to.
The assignments in the parallel reverse algorithm invoked with an execution policy object of type parallel_policy or parallel_task_policy are permitted to execute in an unordered fashion in unspecified threads, and indeterminately sequenced within each thread.
- Return
- The reverse algorithm returns a hpx::future<BidirIter> if the execution policy is of type sequenced_task_policy or parallel_task_policy and returns BidirIter otherwise. It returns last.
-
template<typename
ExPolicy
, typenameRng
, typenameOutIter
>
util::detail::algorithm_result<ExPolicy, hpx::util::tagged_pair<tag::in(typename hpx::traits::range_iterator<Rng>::type), tag::out(OutIter)>>::typereverse_copy
(ExPolicy &&policy, Rng &&rng, OutIter dest_first)¶ Copies the elements from the range [first, last) to another range beginning at dest_first in such a way that the elements in the new range are in reverse order. Behaves as if by executing the assignment *(dest_first + (last - first) - 1 - i) = *(first + i) once for each non-negative i < (last - first) If the source and destination ranges (that is, [first, last) and [dest_first, dest_first+(last-first)) respectively) overlap, the behavior is undefined.
The assignments in the parallel
reverse_copy algorithm invoked with an execution policy object of type sequenced_policy execute in sequential order in the calling thread.- Note
- Complexity: Performs exactly last - first assignments.
- Template Parameters
ExPolicy
: The type of the execution policy to use (deduced). It describes the manner in which the execution of the algorithm may be parallelized and the manner in which it executes the assignments.Rng
: The type of the source range used (deduced). The iterators extracted from this range type must meet the requirements of a bidirectional iterator.OutputIter
: The type of the iterator representing the destination range (deduced). This iterator type must meet the requirements of an output iterator.
- Parameters
policy
: The execution policy to use for the scheduling of the iterations.rng
: Refers to the sequence of elements the algorithm will be applied to.dest_first
: Refers to the begin of the destination range.
The assignments in the parallel reverse_copy algorithm invoked with an execution policy object of type parallel_policy or parallel_task_policy are permitted to execute in an unordered fashion in unspecified threads, and indeterminately sequenced within each thread.
- Return
- The reverse_copy algorithm returns a hpx::future<tagged_pair<tag::in(BidirIter), tag::out(OutIter)> > if the execution policy is of type sequenced_task_policy or parallel_task_policy and returns tagged_pair<tag::in(BidirIter), tag::out(OutIter)> otherwise. The copy algorithm returns the pair of the input iterator forwarded to the first element after the last in the input sequence and the output iterator to the element in the destination range, one past the last element copied.
-
template<typename
ExPolicy
, typenameRng
>
util::detail::algorithm_result<ExPolicy, hpx::util::tagged_pair<tag::begin(typename hpx::traits::range_iterator<Rng>::type), tag::end(typename hpx::traits::range_iterator<Rng>::type)>>::typerotate
(ExPolicy &&policy, Rng &&rng, typename hpx::traits::range_iterator<Rng>::type middle)¶ Performs a left rotation on a range of elements. Specifically, rotate swaps the elements in the range [first, last) in such a way that the element new_first becomes the first element of the new range and new_first - 1 becomes the last element.
The assignments in the parallel
rotate algorithm invoked with an execution policy object of type sequenced_policy execute in sequential order in the calling thread.- Note
- Complexity: Linear in the distance between first and last.
- Template Parameters
ExPolicy
: The type of the execution policy to use (deduced). It describes the manner in which the execution of the algorithm may be parallelized and the manner in which it executes the assignments.Rng
: The type of the source range used (deduced). The iterators extracted from this range type must meet the requirements of a forward iterator.
- Parameters
policy
: The execution policy to use for the scheduling of the iterations.rng
: Refers to the sequence of elements the algorithm will be applied to.middle
: Refers to the element that should appear at the beginning of the rotated range.
The assignments in the parallel rotate algorithm invoked with an execution policy object of type parallel_policy or parallel_task_policy are permitted to execute in an unordered fashion in unspecified threads, and indeterminately sequenced within each thread.
- Note
- The type of dereferenced FwdIter must meet the requirements of MoveAssignable and MoveConstructible.
- Return
- The rotate algorithm returns a hpx::future<tagged_pair<tag::begin(FwdIter), tag::end(FwdIter)> > if the execution policy is of type parallel_task_policy and returns tagged_pair<tag::begin(FwdIter), tag::end(FwdIter)> otherwise. The rotate algorithm returns the iterator equal to pair(first + (last - new_first), last).
-
template<typename
ExPolicy
, typenameRng
, typenameOutIter
>
util::detail::algorithm_result<ExPolicy, hpx::util::tagged_pair<tag::in(typename hpx::traits::range_iterator<Rng>::type), tag::out(OutIter)>>::typerotate_copy
(ExPolicy &&policy, Rng &&rng, typename hpx::traits::range_iterator<Rng>::type middle, OutIter dest_first)¶ Copies the elements from the range [first, last), to another range beginning at dest_first in such a way, that the element new_first becomes the first element of the new range and new_first - 1 becomes the last element.
The assignments in the parallel
rotate_copy algorithm invoked with an execution policy object of type sequenced_policy execute in sequential order in the calling thread.- Note
- Complexity: Performs exactly last - first assignments.
- Template Parameters
ExPolicy
: The type of the execution policy to use (deduced). It describes the manner in which the execution of the algorithm may be parallelized and the manner in which it executes the assignments.Rng
: The type of the source range used (deduced). The iterators extracted from this range type must meet the requirements of a forward iterator.OutIter
: The type of the iterator representing the destination range (deduced). This iterator type must meet the requirements of an output iterator.
- Parameters
policy
: The execution policy to use for the scheduling of the iterations.rng
: Refers to the sequence of elements the algorithm will be applied to.middle
: Refers to the element that should appear at the beginning of the rotated range.dest_first
: Refers to the begin of the destination range.
The assignments in the parallel rotate_copy algorithm invoked with an execution policy object of type parallel_policy or parallel_task_policy are permitted to execute in an unordered fashion in unspecified threads, and indeterminately sequenced within each thread.
- Return
- The rotate_copy algorithm returns a hpx::future<tagged_pair<tag::in(FwdIter), tag::out(OutIter)> > if the execution policy is of type parallel_task_policy and returns tagged_pair<tag::in(FwdIter), tag::out(OutIter)> otherwise. The rotate_copy algorithm returns the output iterator to the element past the last element copied.
-
template<typename
ExPolicy
, typenameRng1
, typenameRng2
, typenamePred
= detail::equal_to, typenameProj1
= util::projection_identity, typenameProj2
= util::projection_identity>
util::detail::algorithm_result<ExPolicy, typename hpx::traits::range_iterator<Rng1>::type>::typesearch
(ExPolicy &&policy, Rng1 &&rng1, Rng2 &&rng2, Pred &&op = Pred(), Proj1 &&proj1 = Proj1(), Proj2 &&proj2 = Proj2())¶ Searches the range [first, last) for any elements in the range [s_first, s_last). Uses a provided predicate to compare elements.
The comparison operations in the parallel
search algorithm invoked with an execution policy object of type sequenced_policy execute in sequential order in the calling thread.- Note
- Complexity: at most (S*N) comparisons where S = distance(s_first, s_last) and N = distance(first, last).
- Template Parameters
ExPolicy
: The type of the execution policy to use (deduced). It describes the manner in which the execution of the algorithm may be parallelized and the manner in which it executes the assignments.Rng1
: The type of the examine range used (deduced). The iterators extracted from this range type must meet the requirements of an input iterator.Rng2
: The type of the search range used (deduced). The iterators extracted from this range type must meet the requirements of an input iterator.Pred
: The type of an optional function/function object to use. Unlike its sequential form, the parallel overload of adjacent_find requires Pred to meet the requirements of CopyConstructible. This defaults to std::equal_to<>Proj1
: The type of an optional projection function. This defaults to util::projection_identity and is applied to the elements of Rng1.Proj2
: The type of an optional projection function. This defaults to util::projection_identity and is applied to the elements of Rng2.
- Parameters
policy
: The execution policy to use for the scheduling of the iterations.rng1
: Refers to the sequence of elements the algorithm will be examining.rng2
: Refers to the sequence of elements the algorithm will be searching for.op
: Refers to the binary predicate which returns true if the elements should be treated as equal. the signature of the function should be equivalent tobool pred(const Type1 &a, const Type2 &b);
The signature does not need to have const &, but the function must not modify the objects passed to it. The types
Type1 and Type2 must be such that objects of types FwdIter1 and FwdIter2 can be dereferenced and then implicitly converted to Type1 and Type2 respectivelyproj1
: Specifies the function (or function object) which will be invoked for each of the elements of rng1 as a projection operation before the actual predicate is invoked.proj2
: Specifies the function (or function object) which will be invoked for each of the elements of rng2 as a projection operation before the actual predicate is invoked.
The comparison operations in the parallel search algorithm invoked with an execution policy object of type parallel_policy or parallel_task_policy are permitted to execute in an unordered fashion in unspecified threads, and indeterminately sequenced within each thread.
- Return
- The search algorithm returns a hpx::future<FwdIter> if the execution policy is of type task_execution_policy and returns FwdIter otherwise. The search algorithm returns an iterator to the beginning of the first subsequence [s_first, s_last) in range [first, last). If the length of the subsequence [s_first, s_last) is greater than the length of the range [first, last), last is returned. Additionally if the size of the subsequence is empty first is returned. If no subsequence is found, last is returned.
-
template<typename
ExPolicy
, typenameRng1
, typenameRng2
, typenamePred
= detail::equal_to, typenameProj1
= util::projection_identity, typenameProj2
= util::projection_identity>
util::detail::algorithm_result<ExPolicy, typename hpx::traits::range_iterator<Rng1>::type>::typesearch_n
(ExPolicy &&policy, Rng1 &&rng1, std::size_t count, Rng2 &&rng2, Pred &&op = Pred(), Proj1 &&proj1 = Proj1(), Proj2 &&proj2 = Proj2())¶ Searches the range [first, last) for any elements in the range [s_first, s_last). Uses a provided predicate to compare elements.
The comparison operations in the parallel
search algorithm invoked with an execution policy object of type sequenced_policy execute in sequential order in the calling thread.- Note
- Complexity: at most (S*N) comparisons where S = distance(s_first, s_last) and N = distance(first, last).
- Template Parameters
ExPolicy
: The type of the execution policy to use (deduced). It describes the manner in which the execution of the algorithm may be parallelized and the manner in which it executes the assignments.Rng1
: The type of the examine range used (deduced). The iterators extracted from this range type must meet the requirements of an input iterator.Rng2
: The type of the search range used (deduced). The iterators extracted from this range type must meet the requirements of an input iterator.Pred
: The type of an optional function/function object to use. Unlike its sequential form, the parallel overload of adjacent_find requires Pred to meet the requirements of CopyConstructible. This defaults to std::equal_to<>Proj1
: The type of an optional projection function. This defaults to util::projection_identity and is applied to the elements of Rng1.Proj2
: The type of an optional projection function. This defaults to util::projection_identity and is applied to the elements of Rng2.
- Parameters
policy
: The execution policy to use for the scheduling of the iterations.rng1
: Refers to the sequence of elements the algorithm will be examining.count
: The number of elements to apply the algorithm on.rng2
: Refers to the sequence of elements the algorithm will be searching for.op
: Refers to the binary predicate which returns true if the elements should be treated as equal. the signature of the function should be equivalent tobool pred(const Type1 &a, const Type2 &b);
The signature does not need to have const &, but the function must not modify the objects passed to it. The types
Type1 and Type2 must be such that objects of types FwdIter1 and FwdIter2 can be dereferenced and then implicitly converted to Type1 and Type2 respectivelyproj1
: Specifies the function (or function object) which will be invoked for each of the elements of rng1 as a projection operation before the actual predicate is invoked.proj2
: Specifies the function (or function object) which will be invoked for each of the elements of rng2 as a projection operation before the actual predicate is invoked.
The comparison operations in the parallel search algorithm invoked with an execution policy object of type parallel_policy or parallel_task_policy are permitted to execute in an unordered fashion in unspecified threads, and indeterminately sequenced within each thread.
- Return
- The search algorithm returns a hpx::future<FwdIter> if the execution policy is of type task_execution_policy and returns FwdIter otherwise. The search algorithm returns an iterator to the beginning of the first subsequence [s_first, s_last) in range [first, last). If the length of the subsequence [s_first, s_last) is greater than the length of the range [first, last), last is returned. Additionally if the size of the subsequence is empty first is returned. If no subsequence is found, last is returned.
-
template<typename
ExPolicy
, typenameRng
, typenameProj
= util::projection_identity, typenameCompare
= detail::less>
util::detail::algorithm_result<ExPolicy, typename hpx::traits::range_iterator<Rng>::type>::typesort
(ExPolicy &&policy, Rng &&rng, Compare &&comp = Compare(), Proj &&proj = Proj())¶ Sorts the elements in the range rng in ascending order. The order of equal elements is not guaranteed to be preserved. The function uses the given comparison function object comp (defaults to using operator<()).
A sequence is sorted with respect to a comparator
comp and a projection proj if for every iterator i pointing to the sequence and every non-negative integer n such that i + n is a valid iterator pointing to an element of the sequence, and INVOKE(comp, INVOKE(proj, *(i + n)), INVOKE(proj, *i)) == false.- Note
- Complexity: O(Nlog(N)), where N = std::distance(begin(rng), end(rng)) comparisons.
comp has to induce a strict weak ordering on the values.
- Template Parameters
ExPolicy
: The type of the execution policy to use (deduced). It describes the manner in which the execution of the algorithm may be parallelized and the manner in which it applies user-provided function objects.Rng
: The type of the source range used (deduced). The iterators extracted from this range type must meet the requirements of an input iterator.Comp
: The type of the function/function object to use (deduced).Proj
: The type of an optional projection function. This defaults to util::projection_identity
- Parameters
policy
: The execution policy to use for the scheduling of the iterations.rng
: Refers to the sequence of elements the algorithm will be applied to.comp
: comp is a callable object. The return value of the INVOKE operation applied to an object of type Comp, when contextually converted to bool, yields true if the first argument of the call is less than the second, and false otherwise. It is assumed that comp will not apply any non-constant function through the dereferenced iterator.proj
: Specifies the function (or function object) which will be invoked for each pair of elements as a projection operation before the actual predicate comp is invoked.
The application of function objects in parallel algorithm invoked with an execution policy object of type sequenced_policy execute in sequential order in the calling thread.
The application of function objects in parallel algorithm invoked with an execution policy object of type parallel_policy or parallel_task_policy are permitted to execute in an unordered fashion in unspecified threads, and indeterminately sequenced within each thread.
- Return
- The sort algorithm returns a hpx::future<Iter> if the execution policy is of type sequenced_task_policy or parallel_task_policy and returns Iter otherwise. It returns last.
-
template<typename
ExPolicy
, typenameRng
, typenameOutIter
, typenameF
, typenameProj
= util::projection_identity>
util::detail::algorithm_result<ExPolicy, hpx::util::tagged_pair<tag::in(typename hpx::traits::range_iterator<Rng>::type), tag::out(OutIter)>>::typetransform
(ExPolicy &&policy, Rng &&rng, OutIter dest, F &&f, Proj &&proj = Proj())¶ Applies the given function f to the given range rng and stores the result in another range, beginning at dest.
The invocations of
f in the parallel transform algorithm invoked with an execution policy object of type sequenced_policy execute in sequential order in the calling thread.- Note
- Complexity: Exactly size(rng) applications of f
- Template Parameters
ExPolicy
: The type of the execution policy to use (deduced). It describes the manner in which the execution of the algorithm may be parallelized and the manner in which it executes the invocations of f.Rng
: The type of the source range used (deduced). The iterators extracted from this range type must meet the requirements of an input iterator.OutIter
: The type of the iterator representing the destination range (deduced). This iterator type must meet the requirements of an output iterator.F
: The type of the function/function object to use (deduced). Unlike its sequential form, the parallel overload of transform requires F to meet the requirements of CopyConstructible.Proj
: The type of an optional projection function. This defaults to util::projection_identity
- Parameters
policy
: The execution policy to use for the scheduling of the iterations.rng
: Refers to the sequence of elements the algorithm will be applied to.dest
: Refers to the beginning of the destination range.f
: Specifies the function (or function object) which will be invoked for each of the elements in the sequence specified by [first, last).This is an unary predicate. The signature of this predicate should be equivalent to:Ret fun(const Type &a);
The signature does not need to have const&. The type
Type must be such that an object of type InIter can be dereferenced and then implicitly converted to Type. The type Ret must be such that an object of type OutIter can be dereferenced and assigned a value of type Ret.proj
: Specifies the function (or function object) which will be invoked for each of the elements as a projection operation before the actual predicate f is invoked.
The invocations of f in the parallel transform algorithm invoked with an execution policy object of type parallel_policy or parallel_task_policy are permitted to execute in an unordered fashion in unspecified threads, and indeterminately sequenced within each thread.
- Return
- The transform algorithm returns a hpx::future<tagged_pair<tag::in(InIter), tag::out(OutIter)> > if the execution policy is of type parallel_task_policy and returns tagged_pair<tag::in(InIter), tag::out(OutIter)> otherwise. The transform algorithm returns a tuple holding an iterator referring to the first element after the input sequence and the output iterator to the element in the destination range, one past the last element copied.
-
template<typename
ExPolicy
, typenameRng
, typenameInIter2
, typenameOutIter
, typenameF
, typenameProj1
= util::projection_identity, typenameProj2
= util::projection_identity>
util::detail::algorithm_result<ExPolicy, hpx::util::tagged_tuple<tag::in1(typename hpx::traits::range_iterator<Rng>::type), tag::in2(InIter2), tag::out(OutIter)>>::typetransform
(ExPolicy &&policy, Rng &&rng, InIter2 first2, OutIter dest, F &&f, Proj1 &&proj1 = Proj1(), Proj2 &&proj2 = Proj2())¶ Applies the given function f to pairs of elements from two ranges: one defined by rng and the other beginning at first2, and stores the result in another range, beginning at dest.
The invocations of
f in the parallel transform algorithm invoked with an execution policy object of type sequenced_policy execute in sequential order in the calling thread.- Note
- Complexity: Exactly size(rng) applications of f
- Template Parameters
ExPolicy
: The type of the execution policy to use (deduced). It describes the manner in which the execution of the algorithm may be parallelized and the manner in which it executes the invocations of f.Rng
: The type of the source range used (deduced). The iterators extracted from this range type must meet the requirements of an input iterator.InIter2
: The type of the source iterators for the second range used (deduced). This iterator type must meet the requirements of an input iterator.OutIter
: The type of the iterator representing the destination range (deduced). This iterator type must meet the requirements of an output iterator.F
: The type of the function/function object to use (deduced). Unlike its sequential form, the parallel overload of transform requires F to meet the requirements of CopyConstructible.Proj1
: The type of an optional projection function to be used for elements of the first sequence. This defaults to util::projection_identityProj2
: The type of an optional projection function to be used for elements of the second sequence. This defaults to util::projection_identity
- Parameters
policy
: The execution policy to use for the scheduling of the iterations.rng
: Refers to the sequence of elements the algorithm will be applied to.first2
: Refers to the beginning of the second sequence of elements the algorithm will be applied to.dest
: Refers to the beginning of the destination range.f
: Specifies the function (or function object) which will be invoked for each of the elements in the sequence specified by [first, last).This is a binary predicate. The signature of this predicate should be equivalent to:Ret fun(const Type1 &a, const Type2 &b);
The signature does not need to have const&. The types
Type1 and Type2 must be such that objects of types InIter1 and InIter2 can be dereferenced and then implicitly converted to Type1 and Type2 respectively. The type Ret must be such that an object of type OutIter can be dereferenced and assigned a value of type Ret.proj1
: Specifies the function (or function object) which will be invoked for each of the elements of the first sequence as a projection operation before the actual predicate f is invoked.proj2
: Specifies the function (or function object) which will be invoked for each of the elements of the second sequence as a projection operation before the actual predicate f is invoked.
The invocations of f in the parallel transform algorithm invoked with an execution policy object of type parallel_policy or parallel_task_policy are permitted to execute in an unordered fashion in unspecified threads, and indeterminately sequenced within each thread.
- Return
- The transform algorithm returns a hpx::future<tagged_tuple<tag::in1(InIter1), tag::in2(InIter2), tag::out(OutIter)> > if the execution policy is of type parallel_task_policy and returns tagged_tuple<tag::in1(InIter1), tag::in2(InIter2), tag::out(OutIter)> otherwise. The transform algorithm returns a tuple holding an iterator referring to the first element after the first input sequence, an iterator referring to the first element after the second input sequence, and the output iterator referring to the element in the destination range, one past the last element copied.
-
template<typename
ExPolicy
, typenameRng1
, typenameRng2
, typenameOutIter
, typenameF
, typenameProj1
= util::projection_identity, typenameProj2
= util::projection_identity>
util::detail::algorithm_result<ExPolicy, hpx::util::tagged_tuple<tag::in1(typename hpx::traits::range_iterator<Rng1>::type), tag::in2(typename hpx::traits::range_iterator<Rng2>::type), tag::out(OutIter)>>::typetransform
(ExPolicy &&policy, Rng1 &&rng1, Rng2 &&rng2, OutIter dest, F &&f, Proj1 &&proj1 = Proj1(), Proj2 &&proj2 = Proj2())¶ Applies the given function f to pairs of elements from two ranges: one defined by [first1, last1) and the other beginning at first2, and stores the result in another range, beginning at dest.
The invocations of
f in the parallel transform algorithm invoked with an execution policy object of type sequenced_policy execute in sequential order in the calling thread.- Note
- Complexity: Exactly min(last2-first2, last1-first1) applications of f
- Template Parameters
ExPolicy
: The type of the execution policy to use (deduced). It describes the manner in which the execution of the algorithm may be parallelized and the manner in which it executes the invocations of f.Rng1
: The type of the first source range used (deduced). The iterators extracted from this range type must meet the requirements of an input iterator.Rng2
: The type of the second source range used (deduced). The iterators extracted from this range type must meet the requirements of an input iterator.OutIter
: The type of the iterator representing the destination range (deduced). This iterator type must meet the requirements of an output iterator.F
: The type of the function/function object to use (deduced). Unlike its sequential form, the parallel overload of transform requires F to meet the requirements of CopyConstructible.Proj1
: The type of an optional projection function to be used for elements of the first sequence. This defaults to util::projection_identityProj2
: The type of an optional projection function to be used for elements of the second sequence. This defaults to util::projection_identity
- Parameters
policy
: The execution policy to use for the scheduling of the iterations.rng1
: Refers to the first sequence of elements the algorithm will be applied to.rng2
: Refers to the second sequence of elements the algorithm will be applied to.dest
: Refers to the beginning of the destination range.f
: Specifies the function (or function object) which will be invoked for each of the elements in the sequence specified by [first, last).This is a binary predicate. The signature of this predicate should be equivalent to:Ret fun(const Type1 &a, const Type2 &b);
The signature does not need to have const&. The types
Type1 and Type2 must be such that objects of types InIter1 and InIter2 can be dereferenced and then implicitly converted to Type1 and Type2 respectively. The type Ret must be such that an object of type OutIter can be dereferenced and assigned a value of type Ret.proj1
: Specifies the function (or function object) which will be invoked for each of the elements of the first sequence as a projection operation before the actual predicate f is invoked.proj2
: Specifies the function (or function object) which will be invoked for each of the elements of the second sequence as a projection operation before the actual predicate f is invoked.
The invocations of f in the parallel transform algorithm invoked with an execution policy object of type parallel_policy or parallel_task_policy are permitted to execute in an unordered fashion in unspecified threads, and indeterminately sequenced within each thread.
- Note
- The algorithm will invoke the binary predicate until it reaches the end of the shorter of the two given input sequences
- Return
- The transform algorithm returns a hpx::future<tagged_tuple<tag::in1(InIter1), tag::in2(InIter2), tag::out(OutIter)> > if the execution policy is of type parallel_task_policy and returns tagged_tuple<tag::in1(InIter1), tag::in2(InIter2), tag::out(OutIter)> otherwise. The transform algorithm returns a tuple holding an iterator referring to the first element r the first input sequence, an iterator referring to the first element after the second input sequence, and the output iterator referring to the element in the destination range, one past the last element copied.
-
template<typename
ExPolicy
, typenameRng
, typenamePred
= detail::equal_to, typenameProj
= util::projection_identity>
util::detail::algorithm_result<ExPolicy, typename hpx::traits::range_iterator<Rng>::type>::typeunique
(ExPolicy &&policy, Rng &&rng, Pred &&pred = Pred(), Proj &&proj = Proj())¶ Eliminates all but the first element from every consecutive group of equivalent elements from the range rng and returns a past-the-end iterator for the new logical end of the range.
The assignments in the parallel
unique algorithm invoked with an execution policy object of type sequenced_policy execute in sequential order in the calling thread.- Note
- Complexity: Performs not more than N assignments, exactly N - 1 applications of the predicate pred and no more than twice as many applications of the projection proj, where N = std::distance(begin(rng), end(rng)).
- Template Parameters
ExPolicy
: The type of the execution policy to use (deduced). It describes the manner in which the execution of the algorithm may be parallelized and the manner in which it executes the assignments.Rng
: The type of the source range used (deduced). The iterators extracted from this range type must meet the requirements of an forward iterator.Pred
: The type of the function/function object to use (deduced). Unlike its sequential form, the parallel overload of unique requires Pred to meet the requirements of CopyConstructible. This defaults to std::equal_to<>Proj
: The type of an optional projection function. This defaults to util::projection_identity
- Parameters
policy
: The execution policy to use for the scheduling of the iterations.rng
: Refers to the sequence of elements the algorithm will be applied to.pred
: Specifies the function (or function object) which will be invoked for each of the elements in the sequence specified by [first, last). This is an binary predicate which returns true for the required elements. The signature of this predicate should be equivalent to:bool pred(const Type &a, const Type &b);
The signature does not need to have const&, but the function must not modify the objects passed to it. The type
Type must be such that an object of type FwdIter1 can be dereferenced and then implicitly converted to Type.proj
: Specifies the function (or function object) which will be invoked for each of the elements as a projection operation before the actual predicate is invoked.
The assignments in the parallel unique algorithm invoked with an execution policy object of type parallel_policy or parallel_task_policy are permitted to execute in an unordered fashion in unspecified threads, and indeterminately sequenced within each thread.
- Return
- The unique algorithm returns a hpx::future<FwdIter> if the execution policy is of type sequenced_task_policy or parallel_task_policy and returns FwdIter otherwise. The unique algorithm returns the iterator to the new end of the range.
-
template<typename
ExPolicy
, typenameRng
, typenameFwdIter2
, typenamePred
= detail::equal_to, typenameProj
= util::projection_identity>
util::detail::algorithm_result<ExPolicy, hpx::util::tagged_pair<tag::in(typename hpx::traits::range_iterator<Rng>::type), tag::out(FwdIter2)>>::typeunique_copy
(ExPolicy &&policy, Rng &&rng, FwdIter2 dest, Pred &&pred = Pred(), Proj &&proj = Proj())¶ Copies the elements from the range rng, to another range beginning at dest in such a way that there are no consecutive equal elements. Only the first element of each group of equal elements is copied.
The assignments in the parallel
unique_copy algorithm invoked with an execution policy object of type sequenced_policy execute in sequential order in the calling thread.- Note
- Complexity: Performs not more than N assignments, exactly N - 1 applications of the predicate pred, where N = std::distance(begin(rng), end(rng)).
- Template Parameters
ExPolicy
: The type of the execution policy to use (deduced). It describes the manner in which the execution of the algorithm may be parallelized and the manner in which it executes the assignments.Rng
: The type of the source range used (deduced). The iterators extracted from this range type must meet the requirements of an forward iterator.FwdIter2
: The type of the iterator representing the destination range (deduced). This iterator type must meet the requirements of an forward iterator.Pred
: The type of the function/function object to use (deduced). Unlike its sequential form, the parallel overload of unique_copy requires Pred to meet the requirements of CopyConstructible. This defaults to std::equal_to<>Proj
: The type of an optional projection function. This defaults to util::projection_identity
- Parameters
policy
: The execution policy to use for the scheduling of the iterations.rng
: Refers to the sequence of elements the algorithm will be applied to.dest
: Refers to the beginning of the destination range.pred
: Specifies the function (or function object) which will be invoked for each of the elements in the sequence specified by the range rng. This is an binary predicate which returns true for the required elements. The signature of this predicate should be equivalent to:bool pred(const Type &a, const Type &b);
The signature does not need to have const&, but the function must not modify the objects passed to it. The type
Type must be such that an object of type FwdIter1 can be dereferenced and then implicitly converted to Type.proj
: Specifies the function (or function object) which will be invoked for each of the elements as a projection operation before the actual predicate is invoked.
The assignments in the parallel unique_copy algorithm invoked with an execution policy object of type parallel_policy or parallel_task_policy are permitted to execute in an unordered fashion in unspecified threads, and indeterminately sequenced within each thread.
- Return
- The unique_copy algorithm returns a hpx::future<tagged_pair<tag::in(FwdIter1), tag::out(FwdIter2)> > if the execution policy is of type sequenced_task_policy or parallel_task_policy and returns tagged_pair<tag::in(FwdIter1), tag::out(FwdIter2)> otherwise. The unique_copy algorithm returns the pair of the source iterator to last, and the destination iterator to the end of the dest range.
-
template<typename
-
namespace
v2
¶ Functions
-
template<typename
ExPolicy
, typenameF
>
util::detail::algorithm_result<ExPolicy>::typedefine_task_block
(ExPolicy &&policy, F &&f)¶ Constructs a task_block, tr, using the given execution policy policy,and invokes the expression f(tr) on the user-provided object, f.
Postcondition: All tasks spawned from
f have finished execution. A call to define_task_block may return on a different thread than that on which it was called.- Template Parameters
ExPolicy
: The type of the execution policy to use (deduced). It describes the manner in which the execution of the task block may be parallelized.F
: The type of the user defined function to invoke inside the define_task_block (deduced). F shall be MoveConstructible.
- Parameters
policy
: The execution policy to use for the scheduling of the iterations.f
: The user defined function to invoke inside the task block. Given an lvalue tr of type task_block, the expression, (void)f(tr), shall be well-formed.
- Note
- It is expected (but not mandated) that f will (directly or indirectly) call tr.run(callable_object).
- Exceptions
An
: exception_list, as specified in Exception Handling.
-
template<typename
F
>
voiddefine_task_block
(F &&f)¶ Constructs a task_block, tr, and invokes the expression f(tr) on the user-provided object, f. This version uses parallel_policy for task scheduling.
Postcondition: All tasks spawned from
f have finished execution. A call to define_task_block may return on a different thread than that on which it was called.- Template Parameters
F
: The type of the user defined function to invoke inside the define_task_block (deduced). F shall be MoveConstructible.
- Parameters
f
: The user defined function to invoke inside the task block. Given an lvalue tr of type task_block, the expression, (void)f(tr), shall be well-formed.
- Note
- It is expected (but not mandated) that f will (directly or indirectly) call tr.run(callable_object).
- Exceptions
An
: exception_list, as specified in Exception Handling.
-
template<typename
ExPolicy
, typenameF
>
util::detail::algorithm_result<ExPolicy>::typedefine_task_block_restore_thread
(ExPolicy &&policy, F &&f)¶ Constructs a task_block, tr, and invokes the expression f(tr) on the user-provided object, f.
Postcondition: All tasks spawned from
f have finished execution. A call to define_task_block_restore_thread always returns on the same thread as that on which it was called.- Template Parameters
ExPolicy
: The type of the execution policy to use (deduced). It describes the manner in which the execution of the task block may be parallelized.F
: The type of the user defined function to invoke inside the define_task_block (deduced). F shall be MoveConstructible.
- Parameters
policy
: The execution policy to use for the scheduling of the iterations.f
: The user defined function to invoke inside the define_task_block. Given an lvalue tr of type task_block, the expression, (void)f(tr), shall be well-formed.
- Exceptions
An
: exception_list, as specified in Exception Handling.
- Note
- It is expected (but not mandated) that f will (directly or indirectly) call tr.run(callable_object).
-
template<typename
F
>
voiddefine_task_block_restore_thread
(F &&f)¶ Constructs a task_block, tr, and invokes the expression f(tr) on the user-provided object, f. This version uses parallel_policy for task scheduling.
Postcondition: All tasks spawned from
f have finished execution. A call to define_task_block_restore_thread always returns on the same thread as that on which it was called.- Template Parameters
F
: The type of the user defined function to invoke inside the define_task_block (deduced). F shall be MoveConstructible.
- Parameters
f
: The user defined function to invoke inside the define_task_block. Given an lvalue tr of type task_block, the expression, (void)f(tr), shall be well-formed.
- Exceptions
An
: exception_list, as specified in Exception Handling.
- Note
- It is expected (but not mandated) that f will (directly or indirectly) call tr.run(callable_object).
-
template<typename
ExPolicy
, typenameI
, typename ...Args
>
util::detail::algorithm_result<ExPolicy>::typefor_loop
(ExPolicy &&policy, typename std::decay<I>::type first, I last, Args&&... args)¶ The for_loop implements loop functionality over a range specified by integral or iterator bounds. For the iterator case, these algorithms resemble for_each from the Parallelism TS, but leave to the programmer when and if to dereference the iterator.
Requires:
I shall be an integral type or meet the requirements of an input iterator type. The args parameter pack shall have at least one element, comprising objects returned by invocations of reduction and/or induction function templates followed by exactly one element invocable element-access function, f. f shall meet the requirements of MoveConstructible.- Template Parameters
ExPolicy
: The type of the execution policy to use (deduced). It describes the manner in which the execution of the algorithm may be parallelized and the manner in which it applies user-provided function objects.I
: The type of the iteration variable. This could be an (forward) iterator type or an integral type.Args
: A parameter pack, it’s last element is a function object to be invoked for each iteration, the others have to be either conforming to the induction or reduction concept.
- Parameters
policy
: The execution policy to use for the scheduling of the iterations.first
: Refers to the beginning of the sequence of elements the algorithm will be applied to.last
: Refers to the end of the sequence of elements the algorithm will be applied to.args
: The last element of this parameter pack is the function (object) to invoke, while the remaining elements of the parameter pack are instances of either induction or reduction objects. The function (or function object) which will be invoked for each of the elements in the sequence specified by [first, last) should expose a signature equivalent to:<ignored> pred(I const& a, ...);
The signature does not need to have const&. It will receive the current value of the iteration variable and one argument for each of the induction or reduction objects passed to the algorithms, representing their current values.
Effects: Applies f to each element in the input sequence, with additional arguments corresponding to the reductions and inductions in the args parameter pack. The length of the input sequence is last - first.
The first element in the input sequence is specified by first. Each subsequent element is generated by incrementing the previous element.
Along with an element from the input sequence, for each member of the
args parameter pack excluding f, an additional argument is passed to each application of f as follows:- Note
- As described in the C++ standard, arithmetic on non-random-access iterators is performed using advance and distance.
- Note
- The order of the elements of the input sequence is important for determining ordinal position of an application of f, even though the applications themselves may be unordered.
If the pack member is an object returned by a call to a reduction function listed in section, then the additional argument is a reference to a view of that reduction object. If the pack member is an object returned by a call to induction, then the additional argument is the induction value for that induction object corresponding to the position of the application of f in the input sequence.
Complexity: Applies f exactly once for each element of the input sequence.
Remarks: If f returns a result, the result is ignored.
- Return
- The for_loop algorithm returns a hpx::future<void> if the execution policy is of type sequenced_task_policy or parallel_task_policy and returns void otherwise.
-
template<typename
I
, typename ...Args
>
voidfor_loop
(typename std::decay<I>::type first, I last, Args&&... args)¶ The for_loop implements loop functionality over a range specified by integral or iterator bounds. For the iterator case, these algorithms resemble for_each from the Parallelism TS, but leave to the programmer when and if to dereference the iterator.
The execution of for_loop without specifying an execution policy is equivalent to specifying parallel::execution::seq as the execution policy.
Requires:
I shall be an integral type or meet the requirements of an input iterator type. The args parameter pack shall have at least one element, comprising objects returned by invocations of reduction and/or induction function templates followed by exactly one element invocable element-access function, f. f shall meet the requirements of MoveConstructible.- Template Parameters
I
: The type of the iteration variable. This could be an (forward) iterator type or an integral type.Args
: A parameter pack, it’s last element is a function object to be invoked for each iteration, the others have to be either conforming to the induction or reduction concept.
- Parameters
first
: Refers to the beginning of the sequence of elements the algorithm will be applied to.last
: Refers to the end of the sequence of elements the algorithm will be applied to.args
: The last element of this parameter pack is the function (object) to invoke, while the remaining elements of the parameter pack are instances of either induction or reduction objects. The function (or function object) which will be invoked for each of the elements in the sequence specified by [first, last) should expose a signature equivalent to:<ignored> pred(I const& a, ...);
The signature does not need to have const&. It will receive the current value of the iteration variable and one argument for each of the induction or reduction objects passed to the algorithms, representing their current values.
Effects: Applies f to each element in the input sequence, with additional arguments corresponding to the reductions and inductions in the args parameter pack. The length of the input sequence is last - first.
The first element in the input sequence is specified by first. Each subsequent element is generated by incrementing the previous element.
Along with an element from the input sequence, for each member of the
args parameter pack excluding f, an additional argument is passed to each application of f as follows:- Note
- As described in the C++ standard, arithmetic on non-random-access iterators is performed using advance and distance.
- Note
- The order of the elements of the input sequence is important for determining ordinal position of an application of f, even though the applications themselves may be unordered.
If the pack member is an object returned by a call to a reduction function listed in section, then the additional argument is a reference to a view of that reduction object. If the pack member is an object returned by a call to induction, then the additional argument is the induction value for that induction object corresponding to the position of the application of f in the input sequence.
Complexity: Applies f exactly once for each element of the input sequence.
Remarks: If f returns a result, the result is ignored.
-
template<typename ExPolicy, typename I, typename S, typename... Args, &&std::is_integral< S >::value>util::detail::algorithm_result<ExPolicy>::type hpx::parallel::v2::for_loop_strided(ExPolicy && policy, typename std::decay< I >::type first, I last, S stride, Args &&... args)
The for_loop_strided implements loop functionality over a range specified by integral or iterator bounds. For the iterator case, these algorithms resemble for_each from the Parallelism TS, but leave to the programmer when and if to dereference the iterator.
Requires:
I shall be an integral type or meet the requirements of an input iterator type. The args parameter pack shall have at least one element, comprising objects returned by invocations of reduction and/or induction function templates followed by exactly one element invocable element-access function, f. f shall meet the requirements of MoveConstructible.- Template Parameters
ExPolicy
: The type of the execution policy to use (deduced). It describes the manner in which the execution of the algorithm may be parallelized and the manner in which it applies user-provided function objects.I
: The type of the iteration variable. This could be an (forward) iterator type or an integral type.S
: The type of the stride variable. This should be an integral type.Args
: A parameter pack, it’s last element is a function object to be invoked for each iteration, the others have to be either conforming to the induction or reduction concept.
- Parameters
policy
: The execution policy to use for the scheduling of the iterations.first
: Refers to the beginning of the sequence of elements the algorithm will be applied to.last
: Refers to the end of the sequence of elements the algorithm will be applied to.stride
: Refers to the stride of the iteration steps. This shall have non-zero value and shall be negative only if I has integral type or meets the requirements of a bidirectional iterator.args
: The last element of this parameter pack is the function (object) to invoke, while the remaining elements of the parameter pack are instances of either induction or reduction objects. The function (or function object) which will be invoked for each of the elements in the sequence specified by [first, last) should expose a signature equivalent to:<ignored> pred(I const& a, ...);
The signature does not need to have const&. It will receive the current value of the iteration variable and one argument for each of the induction or reduction objects passed to the algorithms, representing their current values.
Effects: Applies f to each element in the input sequence, with additional arguments corresponding to the reductions and inductions in the args parameter pack. The length of the input sequence is last - first.
The first element in the input sequence is specified by first. Each subsequent element is generated by incrementing the previous element.
Along with an element from the input sequence, for each member of the
args parameter pack excluding f, an additional argument is passed to each application of f as follows:- Note
- As described in the C++ standard, arithmetic on non-random-access iterators is performed using advance and distance.
- Note
- The order of the elements of the input sequence is important for determining ordinal position of an application of f, even though the applications themselves may be unordered.
If the pack member is an object returned by a call to a reduction function listed in section, then the additional argument is a reference to a view of that reduction object. If the pack member is an object returned by a call to induction, then the additional argument is the induction value for that induction object corresponding to the position of the application of f in the input sequence.
Complexity: Applies f exactly once for each element of the input sequence.
Remarks: If f returns a result, the result is ignored.
- Return
- The for_loop_strided algorithm returns a hpx::future<void> if the execution policy is of type sequenced_task_policy or parallel_task_policy and returns void otherwise.
-
template<typename I, typename S, typename... Args, &&std::is_integral< S >::value>void hpx::parallel::v2::for_loop_strided(typename std::decay< I >::type first, I last, S stride, Args &&... args)
The for_loop_strided implements loop functionality over a range specified by integral or iterator bounds. For the iterator case, these algorithms resemble for_each from the Parallelism TS, but leave to the programmer when and if to dereference the iterator.
The execution of for_loop without specifying an execution policy is equivalent to specifying parallel::execution::seq as the execution policy.
Requires:
I shall be an integral type or meet the requirements of an input iterator type. The args parameter pack shall have at least one element, comprising objects returned by invocations of reduction and/or induction function templates followed by exactly one element invocable element-access function, f. f shall meet the requirements of MoveConstructible.- Template Parameters
I
: The type of the iteration variable. This could be an (forward) iterator type or an integral type.S
: The type of the stride variable. This should be an integral type.Args
: A parameter pack, it’s last element is a function object to be invoked for each iteration, the others have to be either conforming to the induction or reduction concept.
- Parameters
first
: Refers to the beginning of the sequence of elements the algorithm will be applied to.last
: Refers to the end of the sequence of elements the algorithm will be applied to.stride
: Refers to the stride of the iteration steps. This shall have non-zero value and shall be negative only if I has integral type or meets the requirements of a bidirectional iterator.args
: The last element of this parameter pack is the function (object) to invoke, while the remaining elements of the parameter pack are instances of either induction or reduction objects. The function (or function object) which will be invoked for each of the elements in the sequence specified by [first, last) should expose a signature equivalent to:<ignored> pred(I const& a, ...);
The signature does not need to have const&. It will receive the current value of the iteration variable and one argument for each of the induction or reduction objects passed to the algorithms, representing their current values.
Effects: Applies f to each element in the input sequence, with additional arguments corresponding to the reductions and inductions in the args parameter pack. The length of the input sequence is last - first.
The first element in the input sequence is specified by first. Each subsequent element is generated by incrementing the previous element.
Along with an element from the input sequence, for each member of the
args parameter pack excluding f, an additional argument is passed to each application of f as follows:- Note
- As described in the C++ standard, arithmetic on non-random-access iterators is performed using advance and distance.
- Note
- The order of the elements of the input sequence is important for determining ordinal position of an application of f, even though the applications themselves may be unordered.
If the pack member is an object returned by a call to a reduction function listed in section, then the additional argument is a reference to a view of that reduction object. If the pack member is an object returned by a call to induction, then the additional argument is the induction value for that induction object corresponding to the position of the application of f in the input sequence.
Complexity: Applies f exactly once for each element of the input sequence.
Remarks: If f returns a result, the result is ignored.
-
template<typename ExPolicy, typename I, typename Size, typename... Args, &&std::is_integral< Size >::value>util::detail::algorithm_result<ExPolicy>::type hpx::parallel::v2::for_loop_n(ExPolicy && policy, I first, Size size, Args &&... args)
The for_loop_n implements loop functionality over a range specified by integral or iterator bounds. For the iterator case, these algorithms resemble for_each from the Parallelism TS, but leave to the programmer when and if to dereference the iterator.
Requires:
I shall be an integral type or meet the requirements of an input iterator type. The args parameter pack shall have at least one element, comprising objects returned by invocations of reduction and/or induction function templates followed by exactly one element invocable element-access function, f. f shall meet the requirements of MoveConstructible.- Template Parameters
ExPolicy
: The type of the execution policy to use (deduced). It describes the manner in which the execution of the algorithm may be parallelized and the manner in which it applies user-provided function objects.I
: The type of the iteration variable. This could be an (forward) iterator type or an integral type.Size
: The type of a non-negative integral value specifying the number of items to iterate over.Args
: A parameter pack, it’s last element is a function object to be invoked for each iteration, the others have to be either conforming to the induction or reduction concept.
- Parameters
policy
: The execution policy to use for the scheduling of the iterations.first
: Refers to the beginning of the sequence of elements the algorithm will be applied to.size
: Refers to the number of items the algorithm will be applied to.args
: The last element of this parameter pack is the function (object) to invoke, while the remaining elements of the parameter pack are instances of either induction or reduction objects. The function (or function object) which will be invoked for each of the elements in the sequence specified by [first, last) should expose a signature equivalent to:<ignored> pred(I const& a, ...);
The signature does not need to have const&. It will receive the current value of the iteration variable and one argument for each of the induction or reduction objects passed to the algorithms, representing their current values.
Effects: Applies f to each element in the input sequence, with additional arguments corresponding to the reductions and inductions in the args parameter pack. The length of the input sequence is last - first.
The first element in the input sequence is specified by first. Each subsequent element is generated by incrementing the previous element.
Along with an element from the input sequence, for each member of the
args parameter pack excluding f, an additional argument is passed to each application of f as follows:- Note
- As described in the C++ standard, arithmetic on non-random-access iterators is performed using advance and distance.
- Note
- The order of the elements of the input sequence is important for determining ordinal position of an application of f, even though the applications themselves may be unordered.
If the pack member is an object returned by a call to a reduction function listed in section, then the additional argument is a reference to a view of that reduction object. If the pack member is an object returned by a call to induction, then the additional argument is the induction value for that induction object corresponding to the position of the application of f in the input sequence.
Complexity: Applies f exactly once for each element of the input sequence.
Remarks: If f returns a result, the result is ignored.
- Return
- The for_loop_n algorithm returns a hpx::future<void> if the execution policy is of type sequenced_task_policy or parallel_task_policy and returns void otherwise.
-
template<typename I, typename Size, typename... Args, &&std::is_integral< Size >::value>void hpx::parallel::v2::for_loop_n(I first, Size size, Args &&... args)
The for_loop implements loop functionality over a range specified by integral or iterator bounds. For the iterator case, these algorithms resemble for_each from the Parallelism TS, but leave to the programmer when and if to dereference the iterator.
The execution of for_loop without specifying an execution policy is equivalent to specifying parallel::execution::seq as the execution policy.
Requires:
I shall be an integral type or meet the requirements of an input iterator type. The args parameter pack shall have at least one element, comprising objects returned by invocations of reduction and/or induction function templates followed by exactly one element invocable element-access function, f. f shall meet the requirements of MoveConstructible.- Template Parameters
I
: The type of the iteration variable. This could be an (forward) iterator type or an integral type.Size
: The type of a non-negative integral value specifying the number of items to iterate over.Args
: A parameter pack, it’s last element is a function object to be invoked for each iteration, the others have to be either conforming to the induction or reduction concept.
- Parameters
first
: Refers to the beginning of the sequence of elements the algorithm will be applied to.size
: Refers to the number of items the algorithm will be applied to.args
: The last element of this parameter pack is the function (object) to invoke, while the remaining elements of the parameter pack are instances of either induction or reduction objects. The function (or function object) which will be invoked for each of the elements in the sequence specified by [first, last) should expose a signature equivalent to:<ignored> pred(I const& a, ...);
The signature does not need to have const&. It will receive the current value of the iteration variable and one argument for each of the induction or reduction objects passed to the algorithms, representing their current values.
Effects: Applies f to each element in the input sequence, with additional arguments corresponding to the reductions and inductions in the args parameter pack. The length of the input sequence is last - first.
The first element in the input sequence is specified by first. Each subsequent element is generated by incrementing the previous element.
Along with an element from the input sequence, for each member of the
args parameter pack excluding f, an additional argument is passed to each application of f as follows:- Note
- As described in the C++ standard, arithmetic on non-random-access iterators is performed using advance and distance.
- Note
- The order of the elements of the input sequence is important for determining ordinal position of an application of f, even though the applications themselves may be unordered.
If the pack member is an object returned by a call to a reduction function listed in section, then the additional argument is a reference to a view of that reduction object. If the pack member is an object returned by a call to induction, then the additional argument is the induction value for that induction object corresponding to the position of the application of f in the input sequence.
Complexity: Applies f exactly once for each element of the input sequence.
Remarks: If f returns a result, the result is ignored.
-
template<typename ExPolicy, typename I, typename Size, typename S, typename... Args, &&std::is_integral< Size >::value &&std::is_integral< S >::value>util::detail::algorithm_result<ExPolicy>::type hpx::parallel::v2::for_loop_n_strided(ExPolicy && policy, I first, Size size, S stride, Args &&... args)
The for_loop_n_strided implements loop functionality over a range specified by integral or iterator bounds. For the iterator case, these algorithms resemble for_each from the Parallelism TS, but leave to the programmer when and if to dereference the iterator.
Requires:
I shall be an integral type or meet the requirements of an input iterator type. The args parameter pack shall have at least one element, comprising objects returned by invocations of reduction and/or induction function templates followed by exactly one element invocable element-access function, f. f shall meet the requirements of MoveConstructible.- Template Parameters
ExPolicy
: The type of the execution policy to use (deduced). It describes the manner in which the execution of the algorithm may be parallelized and the manner in which it applies user-provided function objects.I
: The type of the iteration variable. This could be an (forward) iterator type or an integral type.Size
: The type of a non-negative integral value specifying the number of items to iterate over.S
: The type of the stride variable. This should be an integral type.Args
: A parameter pack, it’s last element is a function object to be invoked for each iteration, the others have to be either conforming to the induction or reduction concept.
- Parameters
policy
: The execution policy to use for the scheduling of the iterations.first
: Refers to the beginning of the sequence of elements the algorithm will be applied to.size
: Refers to the number of items the algorithm will be applied to.stride
: Refers to the stride of the iteration steps. This shall have non-zero value and shall be negative only if I has integral type or meets the requirements of a bidirectional iterator.args
: The last element of this parameter pack is the function (object) to invoke, while the remaining elements of the parameter pack are instances of either induction or reduction objects. The function (or function object) which will be invoked for each of the elements in the sequence specified by [first, last) should expose a signature equivalent to:<ignored> pred(I const& a, ...);
The signature does not need to have const&. It will receive the current value of the iteration variable and one argument for each of the induction or reduction objects passed to the algorithms, representing their current values.
Effects: Applies f to each element in the input sequence, with additional arguments corresponding to the reductions and inductions in the args parameter pack. The length of the input sequence is last - first.
The first element in the input sequence is specified by first. Each subsequent element is generated by incrementing the previous element.
Along with an element from the input sequence, for each member of the
args parameter pack excluding f, an additional argument is passed to each application of f as follows:- Note
- As described in the C++ standard, arithmetic on non-random-access iterators is performed using advance and distance.
- Note
- The order of the elements of the input sequence is important for determining ordinal position of an application of f, even though the applications themselves may be unordered.
If the pack member is an object returned by a call to a reduction function listed in section, then the additional argument is a reference to a view of that reduction object. If the pack member is an object returned by a call to induction, then the additional argument is the induction value for that induction object corresponding to the position of the application of f in the input sequence.
Complexity: Applies f exactly once for each element of the input sequence.
Remarks: If f returns a result, the result is ignored.
- Return
- The for_loop_n_strided algorithm returns a hpx::future<void> if the execution policy is of type sequenced_task_policy or parallel_task_policy and returns void otherwise.
-
template<typename I, typename Size, typename S, typename... Args, &&std::is_integral< Size >::value &&std::is_integral< S >::value>void hpx::parallel::v2::for_loop_n_strided(I first, Size size, S stride, Args &&... args)
The for_loop_n_strided implements loop functionality over a range specified by integral or iterator bounds. For the iterator case, these algorithms resemble for_each from the Parallelism TS, but leave to the programmer when and if to dereference the iterator.
The execution of for_loop without specifying an execution policy is equivalent to specifying parallel::execution::seq as the execution policy.
Requires:
I shall be an integral type or meet the requirements of an input iterator type. The args parameter pack shall have at least one element, comprising objects returned by invocations of reduction and/or induction function templates followed by exactly one element invocable element-access function, f. f shall meet the requirements of MoveConstructible.- Template Parameters
I
: The type of the iteration variable. This could be an (forward) iterator type or an integral type.Size
: The type of a non-negative integral value specifying the number of items to iterate over.S
: The type of the stride variable. This should be an integral type.Args
: A parameter pack, it’s last element is a function object to be invoked for each iteration, the others have to be either conforming to the induction or reduction concept.
- Parameters
first
: Refers to the beginning of the sequence of elements the algorithm will be applied to.size
: Refers to the number of items the algorithm will be applied to.stride
: Refers to the stride of the iteration steps. This shall have non-zero value and shall be negative only if I has integral type or meets the requirements of a bidirectional iterator.args
: The last element of this parameter pack is the function (object) to invoke, while the remaining elements of the parameter pack are instances of either induction or reduction objects. The function (or function object) which will be invoked for each of the elements in the sequence specified by [first, last) should expose a signature equivalent to:<ignored> pred(I const& a, ...);
The signature does not need to have const&. It will receive the current value of the iteration variable and one argument for each of the induction or reduction objects passed to the algorithms, representing their current values.
Effects: Applies f to each element in the input sequence, with additional arguments corresponding to the reductions and inductions in the args parameter pack. The length of the input sequence is last - first.
The first element in the input sequence is specified by first. Each subsequent element is generated by incrementing the previous element.
Along with an element from the input sequence, for each member of the
args parameter pack excluding f, an additional argument is passed to each application of f as follows:- Note
- As described in the C++ standard, arithmetic on non-random-access iterators is performed using advance and distance.
- Note
- The order of the elements of the input sequence is important for determining ordinal position of an application of f, even though the applications themselves may be unordered.
If the pack member is an object returned by a call to a reduction function listed in section, then the additional argument is a reference to a view of that reduction object. If the pack member is an object returned by a call to induction, then the additional argument is the induction value for that induction object corresponding to the position of the application of f in the input sequence.
Complexity: Applies f exactly once for each element of the input sequence.
Remarks: If f returns a result, the result is ignored.
-
template<typename
T
>
detail::induction_stride_helper<T>induction
(T &&value, std::size_t stride)¶ The function template returns an induction object of unspecified type having a value type and encapsulating an initial value value of that type and, optionally, a stride.
For each element in the input range, a looping algorithm over input sequence S computes an induction value from an induction variable and ordinal position p within S by the formula i + p * stride if a stride was specified or i + p otherwise. This induction value is passed to the element access function.
If the value argument to induction is a non-const lvalue, then that lvalue becomes the live-out object for the returned induction object. For each induction object that has a live-out object, the looping algorithm assigns the value of i + n * stride to the live-out object upon return, where n is the number of elements in the input range.
- Return
- This returns an induction object with value type T, initial value value, and (if specified) stride stride. If T is an lvalue of non-const type, value is used as the live-out object for the induction object; otherwise there is no live-out object.
- Template Parameters
T
: The value type to be used by the induction object.
- Parameters
value
: [in] The initial value to use for the induction objectstride
: [in] The (optional) stride to use for the induction object (default: 1)
-
template<typename
T
, typenameOp
>
detail::reduction_helper<T, typename std::decay<Op>::type>reduction
(T &var, T const &identity, Op &&combiner)¶ The function template returns a reduction object of unspecified type having a value type and encapsulating an identity value for the reduction, a combiner function object, and a live-out object from which the initial value is obtained and into which the final value is stored.
A parallel algorithm uses reduction objects by allocating an unspecified number of instances, called views, of the reduction’s value type. Each view is initialized with the reduction object’s identity value, except that the live-out object (which was initialized by the caller) comprises one of the views. The algorithm passes a reference to a view to each application of an element-access function, ensuring that no two concurrently-executing invocations share the same view. A view can be shared between two applications that do not execute concurrently, but initialization is performed only once per view.
Modifications to the view by the application of element access functions accumulate as partial results. At some point before the algorithm returns, the partial results are combined, two at a time, using the reduction object’s combiner operation until a single value remains, which is then assigned back to the live-out object.
T shall meet the requirements of CopyConstructible and MoveAssignable. The expression var = combiner(var, var) shall be well formed.
- Template Parameters
T
: The value type to be used by the induction object.Op
: The type of the binary function (object) used to perform the reduction operation.
- Parameters
var
: [in,out] The life-out value to use for the reduction object. This will hold the reduced value after the algorithm is finished executing.identity
: [in] The identity value to use for the reduction operation.combiner
: [in] The binary function (object) used to perform a pairwise reduction on the elements.
- Note
- In order to produce useful results, modifications to the view should be limited to commutative operations closely related to the combiner operation. For example if the combiner is plus<T>, incrementing the view would be consistent with the combiner but doubling it or assigning to it would not.
- Return
- This returns a reduction object of unspecified type having a value type of T. When the return value is used by an algorithm, the reference to var is used as the live-out object, new views are initialized to a copy of identity, and views are combined by invoking the copy of combiner, passing it the two views to be combined.
-
template<typename
-
namespace
performance_counters
¶ Functions
-
counter_status
install_counter_type
(std::string const &name, hpx::util::function_nonser<std::int64_t(bool)> const &counter_value, std::string const &helptext = "", std::string const &uom = "", error_code &ec = throws, )¶ Install a new generic performance counter type in a way, which will uninstall it automatically during shutdown.
The function install_counter_type will register a new generic counter type based on the provided function. The counter type will be automatically unregistered during system shutdown. Any consumer querying any instance of this this counter type will cause the provided function to be called and the returned value to be exposed as the counter value.
The counter type is registered such that there can be one counter instance per locality. The expected naming scheme for the counter instances is:
'/objectname{locality#<*>/total}/countername'
where ‘<*>’ is a zero based integer identifying the locality the counter is created on.- Note
- As long as ec is not pre-initialized to hpx::throws this function doesn’t throw but returns the result code using the parameter ec. Otherwise it throws an instance of hpx::exception.
- Return
- If successful, this function returns status_valid_data, otherwise it will either throw an exception or return an error_code from the enum counter_status (also, see note related to parameter ec).
- Note
- The counter type registry is a locality based service. You will have to register each counter type on every locality where a corresponding performance counter will be created.
- Parameters
name
: [in] The global virtual name of the counter type. This name is expected to have the format /objectname/countername.counter_value
: [in] The function to call whenever the counter value is requested by a consumer.helptext
: [in, optional] A longer descriptive text shown to the user to explain the nature of the counters created from this type.uom
: [in] The unit of measure for the new performance counter type.ec
: [in,out] this represents the error status on exit, if this is pre-initialized to hpx::throws the function will throw on error instead.
-
counter_status
install_counter_type
(std::string const &name, hpx::util::function_nonser<std::vector<std::int64_t>(bool)> const &counter_value, std::string const &helptext = "", std::string const &uom = "", error_code &ec = throws, )¶ Install a new generic performance counter type returning an array of values in a way, that will uninstall it automatically during shutdown.
The function install_counter_type will register a new generic counter type that returns an array of values based on the provided function. The counter type will be automatically unregistered during system shutdown. Any consumer querying any instance of this this counter type will cause the provided function to be called and the returned array value to be exposed as the counter value.
The counter type is registered such that there can be one counter instance per locality. The expected naming scheme for the counter instances is:
'/objectname{locality#<*>/total}/countername'
where ‘<*>’ is a zero based integer identifying the locality the counter is created on.- Note
- As long as ec is not pre-initialized to hpx::throws this function doesn’t throw but returns the result code using the parameter ec. Otherwise it throws an instance of hpx::exception.
- Return
- If successful, this function returns status_valid_data, otherwise it will either throw an exception or return an error_code from the enum counter_status (also, see note related to parameter ec).
- Note
- The counter type registry is a locality based service. You will have to register each counter type on every locality where a corresponding performance counter will be created.
- Parameters
name
: [in] The global virtual name of the counter type. This name is expected to have the format /objectname/countername.counter_value
: [in] The function to call whenever the counter value (array of values) is requested by a consumer.helptext
: [in, optional] A longer descriptive text shown to the user to explain the nature of the counters created from this type.uom
: [in] The unit of measure for the new performance counter type.ec
: [in,out] this represents the error status on exit, if this is pre-initialized to hpx::throws the function will throw on error instead.
-
void
install_counter_type
(std::string const &name, counter_type type, error_code &ec = throws)¶ Install a new performance counter type in a way, which will uninstall it automatically during shutdown.
The function install_counter_type will register a new counter type based on the provided counter_type_info. The counter type will be automatically unregistered during system shutdown.
- Return
- If successful, this function returns status_valid_data, otherwise it will either throw an exception or return an error_code from the enum counter_status (also, see note related to parameter ec).
- Note
- The counter type registry is a locality based service. You will have to register each counter type on every locality where a corresponding performance counter will be created.
- Note
- As long as ec is not pre-initialized to hpx::throws this function doesn’t throw but returns the result code using the parameter ec. Otherwise it throws an instance of hpx::exception.
- Parameters
name
: [in] The global virtual name of the counter type. This name is expected to have the format /objectname/countername.type
: [in] The type of the counters of this counter_type.ec
: [in,out] this represents the error status on exit, if this is pre-initialized to hpx::throws the function will throw on error instead.
-
counter_status
install_counter_type
(std::string const &name, counter_type type, std::string const &helptext, std::string const &uom = "", std::uint32_t version = HPX_PERFORMANCE_COUNTER_V1, error_code &ec = throws)¶ Install a new performance counter type in a way, which will uninstall it automatically during shutdown.
The function install_counter_type will register a new counter type based on the provided counter_type_info. The counter type will be automatically unregistered during system shutdown.
- Return
- If successful, this function returns status_valid_data, otherwise it will either throw an exception or return an error_code from the enum counter_status (also, see note related to parameter ec).
- Note
- The counter type registry is a locality based service. You will have to register each counter type on every locality where a corresponding performance counter will be created.
- Note
- As long as ec is not pre-initialized to hpx::throws this function doesn’t throw but returns the result code using the parameter ec. Otherwise it throws an instance of hpx::exception.
- Parameters
name
: [in] The global virtual name of the counter type. This name is expected to have the format /objectname/countername.type
: [in] The type of the counters of this counter_type.helptext
: [in] A longer descriptive text shown to the user to explain the nature of the counters created from this type.uom
: [in] The unit of measure for the new performance counter type.version
: [in] The version of the counter type. This is currently expected to be set to HPX_PERFORMANCE_COUNTER_V1.ec
: [in,out] this represents the error status on exit, if this is pre-initialized to hpx::throws the function will throw on error instead.
-
counter_status
install_counter_type
(std::string const &name, counter_type type, std::string const &helptext, create_counter_func const &create_counter, discover_counters_func const &discover_counters, std::uint32_t version = HPX_PERFORMANCE_COUNTER_V1, std::string const &uom = "", error_code &ec = throws)¶ Install a new generic performance counter type in a way, which will uninstall it automatically during shutdown.
The function install_counter_type will register a new generic counter type based on the provided counter_type_info. The counter type will be automatically unregistered during system shutdown.
- Note
- As long as ec is not pre-initialized to hpx::throws this function doesn’t throw but returns the result code using the parameter ec. Otherwise it throws an instance of hpx::exception.
- Return
- If successful, this function returns status_valid_data, otherwise it will either throw an exception or return an error_code from the enum counter_status (also, see note related to parameter ec).
- Note
- The counter type registry is a locality based service. You will have to register each counter type on every locality where a corresponding performance counter will be created.
- Parameters
name
: [in] The global virtual name of the counter type. This name is expected to have the format /objectname/countername.type
: [in] The type of the counters of this counter_type.helptext
: [in] A longer descriptive text shown to the user to explain the nature of the counters created from this type.version
: [in] The version of the counter type. This is currently expected to be set to HPX_PERFORMANCE_COUNTER_V1.create_counter
: [in] The function which will be called to create a new instance of this counter type.discover_counters
: [in] The function will be called to discover counter instances which can be created.uom
: [in] The unit of measure of the counter type (default: “”)ec
: [in,out] this represents the error status on exit, if this is pre-initialized to hpx::throws the function will throw on error instead.
-
counter_status
-
namespace
resource
¶ Typedefs
Enums
-
enum
partitioner_mode
¶ This enumeration describes the modes available when creating a resource partitioner.
Values:
-
mode_default
= 0¶ Default mode.
-
mode_allow_oversubscription
= 1¶ Allow processing units to be oversubscribed, i.e. multiple worker threads to share a single processing unit.
-
mode_allow_dynamic_pools
= 2¶ Allow worker threads to be added and removed from thread pools.
-
-
enum
scheduling_policy
¶ This enumeration lists the available scheduling policies (or schedulers) when creating thread pools.
Values:
-
user_defined
= -2¶
-
unspecified
= -1¶
-
local
= 0¶
-
local_priority_fifo
= 1¶
-
local_priority_lifo
= 2¶
-
static_
= 3¶
-
static_priority
= 4¶
-
abp_priority_fifo
= 5¶
-
abp_priority_lifo
= 6¶
-
-
enum
-
namespace
this_thread
¶ Functions
-
threads::thread_state_ex_enum
suspend
(threads::thread_state_enum state, threads::thread_id_type const &id, util::thread_description const &description = util::thread_description("this_thread::suspend"), error_code &ec = throws)¶ The function suspend will return control to the thread manager (suspends the current thread). It sets the new state of this thread to the thread state passed as the parameter.
- Note
- Must be called from within a HPX-thread.
- Exceptions
If
:&ec != &throws
, never throws, but will set ec to an appropriate value when an error occurs. Otherwise, this function will throw an hpx::exception with an error code of hpx::yield_aborted if it is signaled with wait_aborted. If called outside of a HPX-thread, this function will throw an hpx::exception with an error code of hpx::null_thread_id. If this function is called while the thread-manager is not running, it will throw an hpx::exception with an error code of hpx::invalid_status.
-
threads::thread_state_ex_enum
suspend
(threads::thread_state_enum state = threads::pending, util::thread_description const &description = util::thread_description("this_thread::suspend"), error_code &ec = throws)¶ The function suspend will return control to the thread manager (suspends the current thread). It sets the new state of this thread to the thread state passed as the parameter.
- Note
- Must be called from within a HPX-thread.
- Exceptions
If
:&ec != &throws
, never throws, but will set ec to an appropriate value when an error occurs. Otherwise, this function will throw an hpx::exception with an error code of hpx::yield_aborted if it is signaled with wait_aborted. If called outside of a HPX-thread, this function will throw an hpx::exception with an error code of hpx::null_thread_id. If this function is called while the thread-manager is not running, it will throw an hpx::exception with an error code of hpx::invalid_status.
-
threads::thread_state_ex_enum
suspend
(util::steady_time_point const &abs_time, threads::thread_id_type const &id, util::thread_description const &description = util::thread_description("this_thread::suspend"), error_code &ec = throws)¶ The function suspend will return control to the thread manager (suspends the current thread). It sets the new state of this thread to suspended and schedules a wakeup for this threads at the given time.
- Note
- Must be called from within a HPX-thread.
- Exceptions
If
:&ec != &throws
, never throws, but will set ec to an appropriate value when an error occurs. Otherwise, this function will throw an hpx::exception with an error code of hpx::yield_aborted if it is signaled with wait_aborted. If called outside of a HPX-thread, this function will throw an hpx::exception with an error code of hpx::null_thread_id. If this function is called while the thread-manager is not running, it will throw an hpx::exception with an error code of hpx::invalid_status.
-
threads::thread_state_ex_enum
suspend
(util::steady_time_point const &abs_time, util::thread_description const &description = util::thread_description("this_thread::suspend"), error_code &ec = throws)¶ The function suspend will return control to the thread manager (suspends the current thread). It sets the new state of this thread to suspended and schedules a wakeup for this threads at the given time.
- Note
- Must be called from within a HPX-thread.
- Exceptions
If
:&ec != &throws
, never throws, but will set ec to an appropriate value when an error occurs. Otherwise, this function will throw an hpx::exception with an error code of hpx::yield_aborted if it is signaled with wait_aborted. If called outside of a HPX-thread, this function will throw an hpx::exception with an error code of hpx::null_thread_id. If this function is called while the thread-manager is not running, it will throw an hpx::exception with an error code of hpx::invalid_status.
-
threads::thread_state_ex_enum
suspend
(util::steady_duration const &rel_time, util::thread_description const &description = util::thread_description("this_thread::suspend"), error_code &ec = throws)¶ The function suspend will return control to the thread manager (suspends the current thread). It sets the new state of this thread to suspended and schedules a wakeup for this threads after the given duration.
- Note
- Must be called from within a HPX-thread.
- Exceptions
If
:&ec != &throws
, never throws, but will set ec to an appropriate value when an error occurs. Otherwise, this function will throw an hpx::exception with an error code of hpx::yield_aborted if it is signaled with wait_aborted. If called outside of a HPX-thread, this function will throw an hpx::exception with an error code of hpx::null_thread_id. If this function is called while the thread-manager is not running, it will throw an hpx::exception with an error code of hpx::invalid_status.
-
threads::thread_state_ex_enum
suspend
(util::steady_duration const &rel_time, threads::thread_id_type const &id, util::thread_description const &description = util::thread_description("this_thread::suspend"), error_code &ec = throws)¶ The function suspend will return control to the thread manager (suspends the current thread). It sets the new state of this thread to suspended and schedules a wakeup for this threads after the given duration.
- Note
- Must be called from within a HPX-thread.
- Exceptions
If
:&ec != &throws
, never throws, but will set ec to an appropriate value when an error occurs. Otherwise, this function will throw an hpx::exception with an error code of hpx::yield_aborted if it is signaled with wait_aborted. If called outside of a HPX-thread, this function will throw an hpx::exception with an error code of hpx::null_thread_id. If this function is called while the thread-manager is not running, it will throw an hpx::exception with an error code of hpx::invalid_status.
-
threads::thread_state_ex_enum
suspend
(std::uint64_t ms, util::thread_description const &description = util::thread_description("this_thread::suspend"), error_code &ec = throws)¶ The function suspend will return control to the thread manager (suspends the current thread). It sets the new state of this thread to suspended and schedules a wakeup for this threads after the given time (specified in milliseconds).
- Note
- Must be called from within a HPX-thread.
- Exceptions
If
:&ec != &throws
, never throws, but will set ec to an appropriate value when an error occurs. Otherwise, this function will throw an hpx::exception with an error code of hpx::yield_aborted if it is signaled with wait_aborted. If called outside of a HPX-thread, this function will throw an hpx::exception with an error code of hpx::null_thread_id. If this function is called while the thread-manager is not running, it will throw an hpx::exception with an error code of hpx::invalid_status.
-
threads::executors::current_executor
get_executor
(error_code &ec = throws)¶ Returns a reference to the executor which was used to create the current thread.
- Exceptions
If
:&ec != &throws
, never throws, but will set ec to an appropriate value when an error occurs. Otherwise, this function will throw an hpx::exception with an error code of hpx::yield_aborted if it is signaled with wait_aborted. If called outside of a HPX-thread, this function will throw an hpx::exception with an error code of hpx::null_thread_id. If this function is called while the thread-manager is not running, it will throw an hpx::exception with an error code of hpx::invalid_status.
-
threads::thread_pool_base *
get_pool
(error_code &ec = throws)¶ Returns a pointer to the pool that was used to run the current thread
- Exceptions
If
:&ec != &throws
, never throws, but will set ec to an appropriate value when an error occurs. Otherwise, this function will throw an hpx::exception with an error code of hpx::yield_aborted if it is signaled with wait_aborted. If called outside of a HPX-thread, this function will throw an hpx::exception with an error code of hpx::null_thread_id. If this function is called while the thread-manager is not running, it will throw an hpx::exception with an error code of hpx::invalid_status.
-
threads::thread_state_ex_enum
-
namespace
threads
¶ Enums
-
enum
thread_state_enum
¶ The thread_state_enum enumerator encodes the current state of a thread instance
Values:
-
unknown
= 0¶
-
active
= 1¶ thread is currently active (running, has resources)
-
pending
= 2¶ thread is pending (ready to run, but no hardware resource available)
-
suspended
= 3¶ thread has been suspended (waiting for synchronization event, but still known and under control of the thread-manager)
-
depleted
= 4¶ thread has been depleted (deeply suspended, it is not known to the thread-manager)
-
terminated
= 5¶ thread has been stopped an may be garbage collected
-
staged
= 6¶ this is not a real thread state, but allows to reference staged task descriptions, which eventually will be converted into thread objects
-
pending_do_not_schedule
= 7¶
-
pending_boost
= 8¶
-
-
enum
thread_priority
¶ This enumeration lists all possible thread-priorities for HPX threads.
Values:
-
thread_priority_unknown
= -1¶
-
thread_priority_default
= 0¶ Will assign the priority of the task to the default (normal) priority.
-
thread_priority_low
= 1¶ Task goes onto a special low priority queue and will not be executed until all high/normal priority tasks are done, even if they are added after the low priority task.
-
thread_priority_normal
= 2¶ Task will be executed when it is taken from the normal priority queue, this is usually a first in-first-out ordering of tasks (depending on scheduler choice). This is the default priority.
-
thread_priority_high_recursive
= 3¶ The task is a high priority task and any child tasks spawned by this task will be made high priority as well - unless they are specifically flagged as non default priority.
-
thread_priority_boost
= 4¶ Same as thread_priority_high except that the thread will fall back to thread_priority_normal if resumed after being suspended.
-
thread_priority_high
= 5¶ Task goes onto a special high priority queue and will be executed before normal/low priority tasks are taken (some schedulers modify the behavior slightly and the documentation for those should be consulted).
-
-
enum
thread_state_ex_enum
¶ The thread_state_ex_enum enumerator encodes the reason why a thread is being restarted
Values:
-
wait_unknown
= 0¶
-
wait_signaled
= 1¶ The thread has been signaled.
-
wait_timeout
= 2¶ The thread has been reactivated after a timeout.
-
wait_terminate
= 3¶ The thread needs to be terminated.
-
wait_abort
= 4¶ The thread needs to be aborted.
-
-
enum
thread_stacksize
¶ A thread_stacksize references any of the possible stack-sizes for HPX threads.
Values:
-
thread_stacksize_unknown
= -1¶
-
thread_stacksize_small
= 1¶ use small stack size
-
thread_stacksize_medium
= 2¶ use medium sized stack size
-
thread_stacksize_large
= 3¶ use large stack size
-
thread_stacksize_huge
= 4¶ use very large stack size
-
thread_stacksize_current
= 5¶ use size of current thread’s stack
-
thread_stacksize_default
= thread_stacksize_small¶ use default stack size
-
thread_stacksize_minimal
= thread_stacksize_small¶ use minimally stack size
-
thread_stacksize_maximal
= thread_stacksize_huge¶ use maximally stack size
-
Functions
-
char const *
get_thread_state_name
(thread_state_enum state)¶ Get the readable string representing the name of the given thread_state constant.
-
char const *
get_thread_priority_name
(thread_priority priority)¶ Get the readable string representing the name of the given thread_priority constant.
-
char const *
get_thread_state_ex_name
(thread_state_ex_enum state)¶ Get the readable string representing the name of the given thread_state_ex_enum constant.
-
char const *
get_thread_state_name
(thread_state state)¶ Get the readable string representing the name of the given thread_state constant.
-
char const *
get_stack_size_name
(std::ptrdiff_t size)¶ Get the readable string representing the given stack size constant.
-
thread_self &
get_self
()¶ The function get_self returns a reference to the (OS thread specific) self reference to the current HPX thread.
-
thread_self *
get_self_ptr
()¶ The function get_self_ptr returns a pointer to the (OS thread specific) self reference to the current HPX thread.
-
thread_self_impl_type *
get_ctx_ptr
()¶ The function get_ctx_ptr returns a pointer to the internal data associated with each coroutine.
-
thread_self *
get_self_ptr_checked
(error_code &ec = throws)¶ The function get_self_ptr_checked returns a pointer to the (OS thread specific) self reference to the current HPX thread.
-
thread_id_type
get_self_id
()¶ The function get_self_id returns the HPX thread id of the current thread (or zero if the current thread is not a HPX thread).
-
thread_id_type
get_parent_id
()¶ The function get_parent_id returns the HPX thread id of the current thread’s parent (or zero if the current thread is not a HPX thread).
- Note
- This function will return a meaningful value only if the code was compiled with HPX_HAVE_THREAD_PARENT_REFERENCE being defined.
-
std::size_t
get_parent_phase
()¶ The function get_parent_phase returns the HPX phase of the current thread’s parent (or zero if the current thread is not a HPX thread).
- Note
- This function will return a meaningful value only if the code was compiled with HPX_HAVE_THREAD_PARENT_REFERENCE being defined.
-
std::size_t
get_self_stacksize
()¶ The function get_self_stacksize returns the stack size of the current thread (or zero if the current thread is not a HPX thread).
-
std::uint32_t
get_parent_locality_id
()¶ The function get_parent_locality_id returns the id of the locality of the current thread’s parent (or zero if the current thread is not a HPX thread).
- Note
- This function will return a meaningful value only if the code was compiled with HPX_HAVE_THREAD_PARENT_REFERENCE being defined.
-
std::uint64_t
get_self_component_id
()¶ The function get_self_component_id returns the lva of the component the current thread is acting on
- Note
- This function will return a meaningful value only if the code was compiled with HPX_HAVE_THREAD_TARGET_ADDRESS being defined.
-
std::int64_t
get_thread_count
(thread_state_enum state = unknown)¶ The function get_thread_count returns the number of currently known threads.
- Note
- If state == unknown this function will not only return the number of currently existing threads, but will add the number of registered task descriptions (which have not been converted into threads yet).
- Parameters
state
: [in] This specifies the thread-state for which the number of threads should be retrieved.
-
std::int64_t
get_thread_count
(thread_priority priority, thread_state_enum state = unknown)¶ The function get_thread_count returns the number of currently known threads.
- Note
- If state == unknown this function will not only return the number of currently existing threads, but will add the number of registered task descriptions (which have not been converted into threads yet).
- Parameters
priority
: [in] This specifies the thread-priority for which the number of threads should be retrieved.state
: [in] This specifies the thread-state for which the number of threads should be retrieved.
-
bool
enumerate_threads
(util::function_nonser<bool(thread_id_type)> const &f, thread_state_enum state = unknown, )¶ The function enumerate_threads will invoke the given function f for each thread with a matching thread state.
- Parameters
f
: [in] The function which should be called for each matching thread. Returning ‘false’ from this function will stop the enumeration process.state
: [in] This specifies the thread-state for which the threads should be enumerated.
-
thread_state
set_thread_state
(thread_id_type const &id, thread_state_enum state = pending, thread_state_ex_enum stateex = wait_signaled, thread_priority priority = thread_priority_normal, bool retry_on_active = true, hpx::error_code &ec = throws)¶ Set the thread state of the thread referenced by the thread_id id.
- Note
- If the thread referenced by the parameter id is in thread_state::active state this function schedules a new thread which will set the state of the thread as soon as its not active anymore. The function returns thread_state::active in this case.
- Return
- This function returns the previous state of the thread referenced by the id parameter. It will return one of the values as defined by the thread_state enumeration. If the thread is not known to the thread-manager the return value will be thread_state::unknown.
- Note
- As long as ec is not pre-initialized to hpx::throws this function doesn’t throw but returns the result code using the parameter ec. Otherwise it throws an instance of hpx::exception.
- Parameters
id
: [in] The thread id of the thread the state should be modified for.state
: [in] The new state to be set for the thread referenced by the id parameter.stateex
: [in] The new extended state to be set for the thread referenced by the id parameter.priority
:ec
: [in,out] this represents the error status on exit, if this is pre-initialized to hpx::throws the function will throw on error instead.
-
thread_id_type
set_thread_state
(thread_id_type const &id, util::steady_time_point const &abs_time, std::atomic<bool> *started, thread_state_enum state = pending, thread_state_ex_enum stateex = wait_timeout, thread_priority priority = thread_priority_normal, bool retry_on_active = true, error_code &ec = throws)¶ Set the thread state of the thread referenced by the thread_id id.
Set a timer to set the state of the given thread to the given new value after it expired (at the given time)
- Return
- Note
- As long as ec is not pre-initialized to hpx::throws this function doesn’t throw but returns the result code using the parameter ec. Otherwise it throws an instance of hpx::exception.
- Parameters
id
: [in] The thread id of the thread the state should be modified for.abs_time
: [in] Absolute point in time for the new thread to be runstarted
: [in,out] A helper variable allowing to track the state of the timer helper threadstate
: [in] The new state to be set for the thread referenced by the id parameter.stateex
: [in] The new extended state to be set for the thread referenced by the id parameter.priority
:ec
: [in,out] this represents the error status on exit, if this is pre-initialized to hpx::throws the function will throw on error instead.
-
thread_id_type
set_thread_state
(thread_id_type const &id, util::steady_time_point const &abs_time, thread_state_enum state = pending, thread_state_ex_enum stateex = wait_timeout, thread_priority priority = thread_priority_normal, bool retry_on_active = true, error_code& = throws)¶
-
thread_id_type
set_thread_state
(thread_id_type const &id, util::steady_duration const &rel_time, thread_state_enum state = pending, thread_state_ex_enum stateex = wait_timeout, thread_priority priority = thread_priority_normal, bool retry_on_active = true, error_code &ec = throws)¶ Set the thread state of the thread referenced by the thread_id id.
Set a timer to set the state of the given thread to the given new value after it expired (after the given duration)
- Return
- Note
- As long as ec is not pre-initialized to hpx::throws this function doesn’t throw but returns the result code using the parameter ec. Otherwise it throws an instance of hpx::exception.
- Parameters
id
: [in] The thread id of the thread the state should be modified for.rel_time
: [in] Time duration after which the new thread should be runstate
: [in] The new state to be set for the thread referenced by the id parameter.stateex
: [in] The new extended state to be set for the thread referenced by the id parameter.priority
:ec
: [in,out] this represents the error status on exit, if this is pre-initialized to hpx::throws the function will throw on error instead.
-
util::thread_description
get_thread_description
(thread_id_type const &id, error_code &ec = throws)¶ The function get_thread_description is part of the thread related API allows to query the description of one of the threads known to the thread-manager.
- Return
- This function returns the description of the thread referenced by the id parameter. If the thread is not known to the thread-manager the return value will be the string “<unknown>”.
- Note
- As long as ec is not pre-initialized to hpx::throws this function doesn’t throw but returns the result code using the parameter ec. Otherwise it throws an instance of hpx::exception.
- Parameters
id
: [in] The thread id of the thread being queried.ec
: [in,out] this represents the error status on exit, if this is pre-initialized to hpx::throws the function will throw on error instead.
-
util::thread_description
set_thread_description
(thread_id_type const &id, util::thread_description const &desc = util::thread_description(), error_code &ec = throws)¶
-
util::thread_description
get_thread_lco_description
(thread_id_type const &id, error_code &ec = throws)¶
-
util::thread_description
set_thread_lco_description
(thread_id_type const &id, util::thread_description const &desc = util::thread_description(), error_code &ec = throws)¶
-
thread_state
get_thread_state
(thread_id_type const &id, error_code &ec = throws)¶ The function get_thread_backtrace is part of the thread related API allows to query the currently stored thread back trace (which is captured during thread suspension).
- Return
- This function returns the currently captured stack back trace of the thread referenced by the id parameter. If the thread is not known to the thread-manager the return value will be the zero.
- Note
- As long as ec is not pre-initialized to hpx::throws this function doesn’t throw but returns the result code using the parameter ec. Otherwise it throws an instance of hpx::exception. The function get_thread_state is part of the thread related API. It queries the state of one of the threads known to the thread-manager.
- Return
- This function returns the thread state of the thread referenced by the id parameter. If the thread is not known to the thread-manager the return value will be terminated.
- Note
- As long as ec is not pre-initialized to hpx::throws this function doesn’t throw but returns the result code using the parameter ec. Otherwise it throws an instance of hpx::exception.
- Parameters
id
: [in] The thread id of the thread being queried.ec
: [in,out] this represents the error status on exit, if this is pre-initialized to hpx::throws the function will throw on error instead.
- Parameters
id
: [in] The thread id of the thread the state should be modified for.ec
: [in,out] this represents the error status on exit, if this is pre-initialized to hpx::throws the function will throw on error instead.
-
std::size_t
get_thread_phase
(thread_id_type const &id, error_code &ec = throws)¶ The function get_thread_phase is part of the thread related API. It queries the phase of one of the threads known to the thread-manager.
- Return
- This function returns the thread phase of the thread referenced by the id parameter. If the thread is not known to the thread-manager the return value will be ~0.
- Note
- As long as ec is not pre-initialized to hpx::throws this function doesn’t throw but returns the result code using the parameter ec. Otherwise it throws an instance of hpx::exception.
- Parameters
id
: [in] The thread id of the thread the phase should be modified for.ec
: [in,out] this represents the error status on exit, if this is pre-initialized to hpx::throws the function will throw on error instead.
-
std::size_t
get_numa_node_number
()¶
-
bool
get_thread_interruption_enabled
(thread_id_type const &id, error_code &ec = throws)¶ Returns whether the given thread can be interrupted at this point.
- Return
- This function returns true if the given thread can be interrupted at this point in time. It will return false otherwise.
- Note
- As long as ec is not pre-initialized to hpx::throws this function doesn’t throw but returns the result code using the parameter ec. Otherwise it throws an instance of hpx::exception.
- Parameters
id
: [in] The thread id of the thread which should be queried.ec
: [in,out] this represents the error status on exit, if this is pre-initialized to hpx::throws the function will throw on error instead.
-
bool
set_thread_interruption_enabled
(thread_id_type const &id, bool enable, error_code &ec = throws)¶ Set whether the given thread can be interrupted at this point.
- Return
- This function returns the previous value of whether the given thread could have been interrupted.
- Note
- As long as ec is not pre-initialized to hpx::throws this function doesn’t throw but returns the result code using the parameter ec. Otherwise it throws an instance of hpx::exception.
- Parameters
id
: [in] The thread id of the thread which should receive the new value.enable
: [in] This value will determine the new interruption enabled status for the given thread.ec
: [in,out] this represents the error status on exit, if this is pre-initialized to hpx::throws the function will throw on error instead.
-
bool
get_thread_interruption_requested
(thread_id_type const &id, error_code &ec = throws)¶ Returns whether the given thread has been flagged for interruption.
- Return
- This function returns true if the given thread was flagged for interruption. It will return false otherwise.
- Note
- As long as ec is not pre-initialized to hpx::throws this function doesn’t throw but returns the result code using the parameter ec. Otherwise it throws an instance of hpx::exception.
- Parameters
id
: [in] The thread id of the thread which should be queried.ec
: [in,out] this represents the error status on exit, if this is pre-initialized to hpx::throws the function will throw on error instead.
-
void
interrupt_thread
(thread_id_type const &id, bool flag, error_code &ec = throws)¶ Flag the given thread for interruption.
- Note
- As long as ec is not pre-initialized to hpx::throws this function doesn’t throw but returns the result code using the parameter ec. Otherwise it throws an instance of hpx::exception.
- Parameters
id
: [in] The thread id of the thread which should be interrupted.flag
: [in] The flag encodes whether the thread should be interrupted (if it is true), or ‘uninterrupted’ (if it is false).ec
: [in,out] this represents the error status on exit, if this is pre-initialized to hpx::throws the function will throw on error instead.
-
void
interrupt_thread
(thread_id_type const &id, error_code &ec = throws)¶
-
void
interruption_point
(thread_id_type const &id, error_code &ec = throws)¶ Interrupt the current thread at this point if it was canceled. This will throw a thread_interrupted exception, which will cancel the thread.
- Note
- As long as ec is not pre-initialized to hpx::throws this function doesn’t throw but returns the result code using the parameter ec. Otherwise it throws an instance of hpx::exception.
- Parameters
id
: [in] The thread id of the thread which should be interrupted.ec
: [in,out] this represents the error status on exit, if this is pre-initialized to hpx::throws the function will throw on error instead.
-
threads::thread_priority
get_thread_priority
(thread_id_type const &id, error_code &ec = throws)¶ Return priority of the given thread
- Note
- As long as ec is not pre-initialized to hpx::throws this function doesn’t throw but returns the result code using the parameter ec. Otherwise it throws an instance of hpx::exception.
- Parameters
id
: [in] The thread id of the thread whose priority is queried.ec
: [in,out] this represents the error status on exit, if this is pre-initialized to hpx::throws the function will throw on error instead.
-
std::ptrdiff_t
get_stack_size
(thread_id_type const &id, error_code &ec = throws)¶ Return stack size of the given thread
- Note
- As long as ec is not pre-initialized to hpx::throws this function doesn’t throw but returns the result code using the parameter ec. Otherwise it throws an instance of hpx::exception.
- Parameters
id
: [in] The thread id of the thread whose priority is queried.ec
: [in,out] this represents the error status on exit, if this is pre-initialized to hpx::throws the function will throw on error instead.
-
threads::executors::current_executor
get_executor
(thread_id_type const &id, error_code &ec = throws)¶ Returns a reference to the executor which was used to create the given thread.
- Exceptions
If
:&ec != &throws
, never throws, but will set ec to an appropriate value when an error occurs. Otherwise, this function will throw an hpx::exception with an error code of hpx::yield_aborted if it is signaled with wait_aborted. If called outside of a HPX-thread, this function will throw an hpx::exception with an error code of hpx::null_thread_id. If this function is called while the thread-manager is not running, it will throw an hpx::exception with an error code of hpx::invalid_status.
-
threads::thread_pool_base *
get_pool
(thread_id_type const &id, error_code &ec = throws)¶ Returns a pointer to the pool that was used to run the current thread
- Exceptions
If
:&ec != &throws
, never throws, but will set ec to an appropriate value when an error occurs. Otherwise, this function will throw an hpx::exception with an error code of hpx::yield_aborted if it is signaled with wait_aborted. If called outside of a HPX-thread, this function will throw an hpx::exception with an error code of hpx::null_thread_id. If this function is called while the thread-manager is not running, it will throw an hpx::exception with an error code of hpx::invalid_status.
-
enum
-
namespace
policies
¶ Enums
-
enum
scheduler_mode
¶ This enumeration describes the possible modes of a scheduler.
Values:
-
nothing_special
= 0¶ can be used to disable all other options.
As the name suggests, this option
-
do_background_work
= 0x1¶ The scheduler will periodically call a provided callback function from a special HPX thread to enable performing background-work, for instance driving networking progress or garbage-collect AGAS.
-
reduce_thread_priority
= 0x02¶ os-thread driving the scheduler will be reduced below normal.
The kernel priority of the
-
delay_exit
= 0x04¶ The scheduler will wait for some unspecified amount of time before exiting the scheduling loop while being terminated to make sure no other work is being scheduled during processing the shutdown request.
Some schedulers have the capability to act as ‘embedded’ schedulers. In this case it needs to periodically invoke a provided callback into the outer scheduler more frequently than normal. This option enables this behavior.
-
enable_elasticity
= 0x10¶ This option allows for the scheduler to dynamically increase and reduce the number of processing units it runs on. Setting this value not succeed for schedulers that do not support this functionality.
-
enable_stealing
= 0x20¶ schedulers to explicitly disable thread stealing
This option allows for certain
-
enable_idle_backoff
= 0x40¶ schedulers to explicitly disable exponential idle-back off
This option allows for certain
-
default_mode
= do_background_work | reduce_thread_priority | delay_exit | enable_stealing | enable_idle_backoff¶ This option represents the default mode.
-
all_flags
= do_background_work | reduce_thread_priority | delay_exit | fast_idle_mode | enable_elasticity | enable_stealing | enable_idle_backoff¶
-
-
enum
-
namespace
traits
¶
-
namespace
util
¶ Functions
-
std::ostream &
operator<<
(std::ostream &ost, checkpoint const &ckp)¶ Operator<< Overload
This overload is the main way to write data from a checkpoint to an object such as a file. Inside the function, the size of the checkpoint will be written to the stream before the checkpoint’s data. The operator>> overload uses this to read the correct number of bytes. Be mindful of this additional write and read when you use different facilities to write out or read in data to a checkpoint!
- Parameters
ost
: Output stream to write to.ckp
: Checkpoint to copy from.
- Return
- Operator<< returns the ostream object.
-
std::istream &
operator>>
(std::istream &ist, checkpoint &ckp)¶ Operator>> Overload
This overload is the main way to read in data from an object such as a file to a checkpoint. It is important to note that inside the function, the first variable to be read is the size of the checkpoint. This size variable is written to the stream before the checkpoint’s data in the operator<< overload. Be mindful of this additional read and write when you use different facilities to read in or write out data from a checkpoint!
- Parameters
ist
: Input stream to write from.ckp
: Checkpoint to write to.
- Return
- Operator>> returns the ostream object.
-
template<typename
T
, typename ...Ts
, typenameU
= typename std::enable_if<!hpx::traits::is_launch_policy<T>::value && !std::is_same<typename std::decay<T>::type, checkpoint>::value>::type>
hpx::future<checkpoint>save_checkpoint
(T &&t, Ts&&... ts)¶ Save_checkpoint
Save_checkpoint takes any number of objects which a user may wish to store and returns a future to a checkpoint object. Additionally the function can take a policy as a first object which changes its behavior depending on the policy passed to it. Most notably, if a sync policy is used save_checkpoint will simply return a checkpoint object.
- Template Parameters
T
: Containers passed to save_checkpoint to be serialized and placed into a checkpoint object.Ts
: More containers passed to save_checkpoint to be serialized and placed into a checkpoint object.U
: This parameter is used to make sure that T is not a launch policy or a checkpoint. This forces the compiler to choose the correct overload.
- Parameters
t
: A container to restore.ts
: Other containers to restore Containers must be in the same order that they were inserted into the checkpoint.
- Return
- Save_checkpoint returns a future to a checkpoint with one exception: if you pass hpx::launch::sync as the first argument. In this case save_checkpoint will simply return a checkpoint.
-
template<typename
T
, typename ...Ts
>
hpx::future<checkpoint>save_checkpoint
(checkpoint &&c, T &&t, Ts&&... ts)¶ Save_checkpoint - Take a pre-initialized checkpoint
Save_checkpoint takes any number of objects which a user may wish to store and returns a future to a checkpoint object. Additionally the function can take a policy as a first object which changes its behavior depending on the policy passed to it. Most notably, if a sync policy is used save_checkpoint will simply return a checkpoint object.
- Template Parameters
T
: Containers passed to save_checkpoint to be serialized and placed into a checkpoint object.Ts
: More containers passed to save_checkpoint to be serialized and placed into a checkpoint object.
- Parameters
c
: Takes a pre-initialized checkpoint to copy data into.t
: A container to restore.ts
: Other containers to restore Containers must be in the same order that they were inserted into the checkpoint.
- Return
- Save_checkpoint returns a future to a checkpoint with one exception: if you pass hpx::launch::sync as the first argument. In this case save_checkpoint will simply return a checkpoint.
-
template<typename
T
, typename ...Ts
>
hpx::future<checkpoint>save_checkpoint
(hpx::launch p, T &&t, Ts&&... ts)¶ Save_checkpoint - Policy overload
Save_checkpoint takes any number of objects which a user may wish to store and returns a future to a checkpoint object. Additionally the function can take a policy as a first object which changes its behavior depending on the policy passed to it. Most notably, if a sync policy is used save_checkpoint will simply return a checkpoint object.
- Template Parameters
T
: Containers passed to save_checkpoint to be serialized and placed into a checkpoint object.Ts
: More containers passed to save_checkpoint to be serialized and placed into a checkpoint object.
- Parameters
p
: Takes an HPX launch policy. Allows the user to change the way the function is launched i.e. async, sync, etc.t
: A container to restore.ts
: Other containers to restore Containers must be in the same order that they were inserted into the checkpoint.
- Return
- Save_checkpoint returns a future to a checkpoint with one exception: if you pass hpx::launch::sync as the first argument. In this case save_checkpoint will simply return a checkpoint.
-
template<typename
T
, typename ...Ts
>
hpx::future<checkpoint>save_checkpoint
(hpx::launch p, checkpoint &&c, T &&t, Ts&&... ts)¶ Save_checkpoint - Policy overload & pre-initialized checkpoint
Save_checkpoint takes any number of objects which a user may wish to store and returns a future to a checkpoint object. Additionally the function can take a policy as a first object which changes its behavior depending on the policy passed to it. Most notably, if a sync policy is used save_checkpoint will simply return a checkpoint object.
- Template Parameters
T
: Containers passed to save_checkpoint to be serialized and placed into a checkpoint object.Ts
: More containers passed to save_checkpoint to be serialized and placed into a checkpoint object.
- Parameters
p
: Takes an HPX launch policy. Allows the user to change the way the function is launched i.e. async, sync, etc.c
: Takes a pre-initialized checkpoint to copy data into.t
: A container to restore.ts
: Other containers to restore Containers must be in the same order that they were inserted into the checkpoint.
- Return
- Save_checkpoint returns a future to a checkpoint with one exception: if you pass hpx::launch::sync as the first argument. In this case save_checkpoint will simply return a checkpoint.
-
template<typename
T
, typename ...Ts
, typenameU
= typename std::enable_if<!std::is_same<typename std::decay<T>::type, checkpoint>::value>::type>
checkpointsave_checkpoint
(hpx::launch::sync_policy sync_p, T &&t, Ts&&... ts)¶ Save_checkpoint - Sync_policy overload
Save_checkpoint takes any number of objects which a user may wish to store and returns a future to a checkpoint object. Additionally the function can take a policy as a first object which changes its behavior depending on the policy passed to it. Most notably, if a sync policy is used save_checkpoint will simply return a checkpoint object.
- Template Parameters
T
: Containers passed to save_checkpoint to be serialized and placed into a checkpoint object.Ts
: More containers passed to save_checkpoint to be serialized and placed into a checkpoint object.U
: This parameter is used to make sure that T is not a checkpoint. This forces the compiler to choose the correct overload.
- Parameters
sync_p
: hpx::launch::sync_policyt
: A container to restore.ts
: Other containers to restore Containers must be in the same order that they were inserted into the checkpoint.
- Return
- Save_checkpoint which is passed hpx::launch::sync_policy will return a checkpoint which contains the serialized values checkpoint.
-
template<typename
T
, typename ...Ts
>
checkpointsave_checkpoint
(hpx::launch::sync_policy sync_p, checkpoint &&c, T &&t, Ts&&... ts)¶ Save_checkpoint - Sync_policy overload & pre-init. checkpoint
Save_checkpoint takes any number of objects which a user may wish to store and returns a future to a checkpoint object. Additionally the function can take a policy as a first object which changes its behavior depending on the policy passed to it. Most notably, if a sync policy is used save_checkpoint will simply return a checkpoint object.
- Template Parameters
T
: Containers passed to save_checkpoint to be serialized and placed into a checkpoint object.Ts
: More containers passed to save_checkpoint to be serialized and placed into a checkpoint object.
- Parameters
sync_p
: hpx::launch::sync_policyc
: Takes a pre-initialized checkpoint to copy data into.t
: A container to restore.ts
: Other containers to restore Containers must be in the same order that they were inserted into the checkpoint.
- Return
- Save_checkpoint which is passed hpx::launch::sync_policy will return a checkpoint which contains the serialized values checkpoint.
-
template<typename
T
, typename ...Ts
>
voidrestore_checkpoint
(checkpoint const &c, T &t, Ts&... ts)¶ Resurrect
Restore_checkpoint takes a checkpoint object as a first argument and the containers which will be filled from the byte stream (in the same order as they were placed in save_checkpoint).
- Return
- Restore_checkpoint returns void.
- Template Parameters
T
: A container to restore.Ts
: Other containers to restore. Containers must be in the same order that they were inserted into the checkpoint.
- Parameters
c
: The checkpoint to restore.t
: A container to restore.ts
: Other containers to restore Containers must be in the same order that they were inserted into the checkpoint.
-
void
attach_debugger
()¶ Tries to break an attached debugger, if not supported a loop is invoked which gives enough time to attach a debugger manually.
-
template<typename F, typename ... Ts>HPX_HOST_DEVICE util::invoke_result<F, Ts...>::type hpx::util::invoke(F && f, Ts &&... vs)
Invokes the given callable object f with the content of the argument pack vs
- Return
- The result of the callable object when it’s called with the given argument types.
- Note
- This function is similar to
std::invoke
(C++17) - Parameters
f
: Requires to be a callable object. If f is a member function pointer, the first argument in the pack will be treated as the callee (this object).vs
: An arbitrary pack of arguments
- Exceptions
std::exception
: like objects thrown by call to object f with the argument types vs.
-
template<typename R, typename F, typename ... Ts>HPX_HOST_DEVICE R hpx::util::invoke_r(F && f, Ts &&... vs)
Invokes the given callable object f with the content of the argument pack vs
- Return
- The result of the callable object when it’s called with the given argument types.
- Note
- This function is similar to
std::invoke
(C++17) - Parameters
f
: Requires to be a callable object. If f is a member function pointer, the first argument in the pack will be treated as the callee (this object).vs
: An arbitrary pack of arguments
- Exceptions
std::exception
: like objects thrown by call to object f with the argument types vs.
- Template Parameters
R
: The result type of the function when it’s called with the content of the given argument types vs.
-
template<typename F, typename Tuple>HPX_HOST_DEVICE detail::invoke_fused_result<F, Tuple>::type hpx::util::invoke_fused(F && f, Tuple && t)
Invokes the given callable object f with the content of the sequenced type t (tuples, pairs)
- Return
- The result of the callable object when it’s called with the content of the given sequenced type.
- Note
- This function is similar to
std::apply
(C++17) - Parameters
f
: Must be a callable object. If f is a member function pointer, the first argument in the sequenced type will be treated as the callee (this object).t
: A type which is content accessible through a call to hpx::util::get.
- Exceptions
std::exception
: like objects thrown by call to object f with the arguments contained in the sequenceable type t.
-
template<typename R, typename F, typename Tuple>HPX_HOST_DEVICE R hpx::util::invoke_fused_r(F && f, Tuple && t)
Invokes the given callable object f with the content of the sequenced type t (tuples, pairs)
- Return
- The result of the callable object when it’s called with the content of the given sequenced type.
- Note
- This function is similar to
std::apply
(C++17) - Parameters
f
: Must be a callable object. If f is a member function pointer, the first argument in the sequenced type will be treated as the callee (this object).t
: A type which is content accessible through a call to hpx::util::get.
- Exceptions
std::exception
: like objects thrown by call to object f with the arguments contained in the sequenceable type t.
- Template Parameters
R
: The result type of the function when it’s called with the content of the given sequenced type.
-
template<typename Mapper, typename... T><unspecified> hpx::util::map_pack(Mapper && mapper, T &&... pack)
Maps the pack with the given mapper.
This function tries to visit all plain elements which may be wrapped in:
- homogeneous containers (
std::vector
,std::list
) - heterogenous containers
(hpx::tuple
,std::pair
,std::array
) and re-assembles the pack with the result of the mapper. Mapping from one type to a different one is supported.
Elements that aren’t accepted by the mapper are routed through and preserved through the hierarchy.
// Maps all integers to floats map_pack([](int value) { return float(value); }, 1, hpx::util::make_tuple(2, std::vector<int>{3, 4}), 5);
- Return
- The mapped element or in case the pack contains multiple elements, the pack is wrapped into a
hpx::tuple
. - Exceptions
std::exception
: like objects which are thrown by an invocation to the mapper.
- Parameters
mapper
: A callable object, which accept an arbitrary type and maps it to another type or the same one.pack
: An arbitrary variadic pack which may contain any type.
- homogeneous containers (
-
template<typename
Visitor
, typename ...T
>
autotraverse_pack_async
(Visitor &&visitor, T&&... pack)¶ Traverses the pack with the given visitor in an asynchronous way.
This function works in the same way as
traverse_pack
, however, we are able to suspend and continue the traversal at later time. Thus we require a visitor callable object which provides threeoperator()
overloads as depicted by the code sample below:struct my_async_visitor { template <typename T> bool operator()(async_traverse_visit_tag, T&& element) { return true; } template <typename T, typename N> void operator()(async_traverse_detach_tag, T&& element, N&& next) { } template <typename T> void operator()(async_traverse_complete_tag, T&& pack) { } };
See
traverse_pack
for a detailed description about the traversal behavior and capabilities.- Return
- A boost::intrusive_ptr that references an instance of the given visitor object.
- Parameters
visitor
: A visitor object which provides the threeoperator()
overloads that were described above. Additionally the visitor must be compatible for referencing it from aboost::intrusive_ptr
. The visitor should must have a virtual destructor!pack
: The arbitrary parameter pack which is traversed asynchronously. Nested objects inside containers and tuple like types are traversed recursively.
-
template<typename
Allocator
, typenameVisitor
, typename ...T
>
autotraverse_pack_async_allocator
(Allocator const &alloc, Visitor &&visitor, T&&... pack)¶ Traverses the pack with the given visitor in an asynchronous way.
This function works in the same way as
traverse_pack
, however, we are able to suspend and continue the traversal at later time. Thus we require a visitor callable object which provides threeoperator()
overloads as depicted by the code sample below:struct my_async_visitor { template <typename T> bool operator()(async_traverse_visit_tag, T&& element) { return true; } template <typename T, typename N> void operator()(async_traverse_detach_tag, T&& element, N&& next) { } template <typename T> void operator()(async_traverse_complete_tag, T&& pack) { } };
See
traverse_pack
for a detailed description about the traversal behavior and capabilities.- Return
- A boost::intrusive_ptr that references an instance of the given visitor object.
- Parameters
visitor
: A visitor object which provides the threeoperator()
overloads that were described above. Additionally the visitor must be compatible for referencing it from aboost::intrusive_ptr
. The visitor should must have a virtual destructor!pack
: The arbitrary parameter pack which is traversed asynchronously. Nested objects inside containers and tuple like types are traversed recursively.alloc
: Allocator instance to use to create the traversal frame.
-
template<typename ...
Args
>
autounwrap
(Args&&... args)¶ A helper function for retrieving the actual result of any hpx::lcos::future like type which is wrapped in an arbitrary way.
Unwraps the given pack of arguments, so that any hpx::lcos::future object is replaced by its future result type in the argument pack:
hpx::future<int>
->int
hpx::future<std::vector<float>>
->std::vector<float>
std::vector<future<float>>
->std::vector<float>
The function is capable of unwrapping hpx::lcos::future like objects that are wrapped inside any container or tuple like type, see hpx::util::map_pack() for a detailed description about which surrounding types are supported. Non hpx::lcos::future like types are permitted as arguments and passed through.
// Single arguments int i1 = hpx:util::unwrap(hpx::lcos::make_ready_future(0)); // Multiple arguments hpx::tuple<int, int> i2 = hpx:util::unwrap(hpx::lcos::make_ready_future(1), hpx::lcos::make_ready_future(2));
- Note
- This function unwraps the given arguments until the first traversed nested hpx::lcos::future which corresponds to an unwrapping depth of one. See hpx::util::unwrap_n() for a function which unwraps the given arguments to a particular depth or hpx::util::unwrap_all() that unwraps all future like objects recursively which are contained in the arguments.
- Return
- Depending on the count of arguments this function returns a hpx::util::tuple containing the unwrapped arguments if multiple arguments are given. In case the function is called with a single argument, the argument is unwrapped and returned.
- Parameters
args
: the arguments that are unwrapped which may contain any arbitrary future or non future type.
- Exceptions
std::exception
: like objects in case any of the given wrapped hpx::lcos::future objects were resolved through an exception. See hpx::lcos::future::get() for details.
-
template<std::size_t
Depth
, typename ...Args
>
autounwrap_n
(Args&&... args)¶ An alterntive version of hpx::util::unwrap(), which unwraps the given arguments to a certain depth of hpx::lcos::future like objects.
See unwrap for a detailed description.
- Template Parameters
Depth
: The count of hpx::lcos::future like objects which are unwrapped maximally.
-
template<typename ...
Args
>
autounwrap_all
(Args&&... args)¶ An alterntive version of hpx::util::unwrap(), which unwraps the given arguments recursively so that all contained hpx::lcos::future like objects are replaced by their actual value.
See hpx::util::unwrap() for a detailed description.
-
template<typename
T
>
autounwrapping
(T &&callable)¶ Returns a callable object which unwraps its arguments upon invocation using the hpx::util::unwrap() function and then passes the result to the given callable object.
auto callable = hpx::util::unwrapping([](int left, int right) { return left + right; }); int i1 = callable(hpx::lcos::make_ready_future(1), hpx::lcos::make_ready_future(2));
See hpx::util::unwrap() for a detailed description.
- Parameters
callable
: the callable object which which is called with the result of the corresponding unwrap function.
-
template<std::size_t
Depth
, typenameT
>
autounwrapping_n
(T &&callable)¶ Returns a callable object which unwraps its arguments upon invocation using the hpx::util::unwrap_n() function and then passes the result to the given callable object.
See hpx::util::unwrapping() for a detailed description.
-
template<typename
T
>
autounwrapping_all
(T &&callable)¶ Returns a callable object which unwraps its arguments upon invocation using the hpx::util::unwrap_all() function and then passes the result to the given callable object.
See hpx::util::unwrapping() for a detailed description.
-
std::ostream &
-
namespace
functional
¶
-
file
migrate_from_storage.hpp
- #include <hpx/config.hpp>#include <hpx/lcos/future.hpp>#include <hpx/error_code.hpp>#include <hpx/lcos/detail/future_data.hpp>#include <hpx/lcos/local/detail/condition_variable.hpp>#include <hpx/lcos/local/spinlock.hpp>#include <hpx/runtime/threads/thread_data_fwd.hpp>#include <hpx/runtime/threads/thread_enums.hpp>#include <hpx/util/steady_clock.hpp>#include <boost/intrusive/slist.hpp>#include <cstddef>#include <mutex>#include <utility>#include <hpx/runtime/launch_policy.hpp>#include <hpx/runtime/serialization/serialization_fwd.hpp>#include <type_traits>#include <hpx/runtime/threads/coroutines/detail/get_stack_pointer.hpp>#include <limits>#include <hpx/runtime/threads/thread_executor.hpp>#include <hpx/runtime/get_os_thread_count.hpp>#include <hpx/runtime/threads/cpu_mask.hpp>#include <hpx/util/assert.hpp>#include <climits>#include <cstdint>#include <string>#include <hpx/runtime/threads/policies/scheduler_mode.hpp>#include <hpx/runtime/threads/topology.hpp>#include <hpx/compat/thread.hpp>#include <thread>#include <hpx/exception_fwd.hpp>#include <hpx/runtime/naming_fwd.hpp>#include <hpx/runtime/resource/partitioner_fwd.hpp>#include <hpx/runtime/threads/policies/callback_notifier.hpp>#include <hpx/runtime/threads_fwd.hpp>#include <hpx/util/function.hpp>#include <exception>#include <hpx/config/warnings_prefix.hpp>#include <hpx/config/warnings_suffix.hpp>#include <memory>#include <hpx/util/spinlock.hpp>#include <hpx/util/itt_notify.hpp>#include <hpx/util/register_locks.hpp>#include <boost/smart_ptr/detail/spinlock.hpp>#include <hpx/util/static.hpp>#include <hpx/compat/mutex.hpp>#include <iosfwd>#include <vector>#include <hwloc.h>#include <hpx/util/atomic_count.hpp>#include <atomic>#include <hpx/util/thread_description.hpp>#include <hpx/util/unique_function.hpp>#include <boost/intrusive_ptr.hpp>#include <chrono>#include <hpx/runtime/threads/thread_helpers.hpp>#include <hpx/throw_exception.hpp>#include <hpx/traits/future_access.hpp>#include <hpx/traits/future_traits.hpp>#include <hpx/traits/is_future.hpp>#include <boost/ref.hpp>#include <functional>#include <hpx/traits/get_remote_result.hpp>#include <hpx/util/annotated_function.hpp>#include <hpx/util/assert_owns_lock.hpp>#include <hpx/traits/has_member_xxx.hpp>#include <hpx/util/bind.hpp>#include <hpx/traits/get_function_address.hpp>#include <hpx/traits/get_function_annotation.hpp>#include <hpx/traits/is_action.hpp>#include <hpx/traits/is_bind_expression.hpp>#include <hpx/traits/is_placeholder.hpp>#include <boost/bind/arg.hpp>#include <hpx/util/decay.hpp>#include <hpx/util/detail/pack.hpp>#include <hpx/util/invoke.hpp>#include <hpx/util/invoke_fused.hpp>#include <hpx/util/result_of.hpp>#include <hpx/util/tuple.hpp>#include <hpx/util/void_guard.hpp>#include <hpx/util/one_shot.hpp>#include <hpx/util/unused.hpp>#include <boost/container/small_vector.hpp>#include <hpx/lcos/detail/future_traits.hpp>#include <hpx/util/always_void.hpp>#include <iterator>#include <hpx/lcos_fwd.hpp>#include <hpx/traits/is_component.hpp>#include <hpx/traits/promise_local_result.hpp>#include <hpx/traits/promise_remote_result.hpp>#include <hpx/runtime/actions/continuation_fwd.hpp>#include <hpx/runtime/serialization/detail/polymorphic_nonintrusive_factory.hpp>#include <hpx/preprocessor/stringize.hpp>#include <hpx/preprocessor/strip_parens.hpp>#include <hpx/runtime/serialization/detail/non_default_constructible.hpp>#include <hpx/traits/needs_automatic_registration.hpp>#include <hpx/traits/polymorphic_traits.hpp>#include <hpx/traits/has_xxx.hpp>#include <hpx/preprocessor/cat.hpp>#include <hpx/util/debug/demangle_helper.hpp>#include <hpx/util/jenkins_hash.hpp>#include <typeinfo>#include <unordered_map>#include <hpx/traits/acquire_shared_state.hpp>#include <hpx/util/range.hpp>#include <hpx/traits/detail/reserve.hpp>#include <hpx/traits/is_range.hpp>#include <hpx/traits/is_future_range.hpp>#include <algorithm>#include <hpx/traits/concepts.hpp>#include <hpx/traits/future_then_result.hpp>#include <hpx/util/identity.hpp>#include <hpx/util/lazy_conditional.hpp>#include <hpx/traits/is_executor.hpp>#include <hpx/traits/is_callable.hpp>#include <hpx/traits/is_launch_policy.hpp>#include <hpx/traits/executor_traits.hpp>#include <hpx/util/detected.hpp>#include <hpx/util/allocator_deleter.hpp>#include <hpx/util/internal_allocator.hpp>#include <hpx/util/lazy_enable_if.hpp>#include <hpx/util/serialize_exception.hpp>#include <hpx/lcos/local/packaged_continuation.hpp>#include <hpx/parallel/executors/execution.hpp>#include <hpx/parallel/executors/execution_fwd.hpp>#include <hpx/parallel/executors/fused_bulk_execute.hpp>#include <hpx/util/deferred_call.hpp>#include <hpx/exception_list.hpp>#include <hpx/exception.hpp>#include <boost/system/error_code.hpp>#include <list>#include <hpx/lcos/dataflow.hpp>#include <hpx/lcos/detail/future_transforms.hpp>#include <hpx/traits/acquire_future.hpp>#include <array>#include <hpx/runtime/get_worker_thread_num.hpp>#include <hpx/traits/extract_action.hpp>#include <hpx/util/pack_traversal_async.hpp>#include <hpx/util/detail/pack_traversal_async_impl.hpp>#include <hpx/util/detail/container_category.hpp>#include <hpx/traits/is_tuple_like.hpp>#include <hpx/parallel/executors/parallel_executor.hpp>#include <hpx/async_launch_policy_dispatch.hpp>#include <hpx/lcos/async_fwd.hpp>#include <hpx/lcos/local/futures_factory.hpp>#include <hpx/lcos/local/latch.hpp>#include <hpx/util/cache_aligned_data.hpp>#include <hpx/parallel/algorithms/detail/predicates.hpp>#include <hpx/traits/is_iterator.hpp>#include <boost/iterator/iterator_categories.hpp>#include <hpx/parallel/algorithms/detail/is_negative.hpp>#include <cstdlib>#include <hpx/parallel/executors/post_policy_dispatch.hpp>#include <hpx/parallel/executors/static_chunk_size.hpp>#include <hpx/runtime/serialization/serialize.hpp>#include <hpx/runtime/serialization/access.hpp>#include <hpx/runtime/serialization/brace_initializable_fwd.hpp>#include <hpx/traits/brace_initializable_traits.hpp>#include <hpx/runtime/serialization/input_archive.hpp>#include <hpx/runtime/serialization/basic_archive.hpp>#include <iostream>#include <map>#include <hpx/runtime/serialization/detail/raw_ptr.hpp>#include <hpx/runtime/serialization/detail/pointer.hpp>#include <hpx/runtime/serialization/detail/polymorphic_id_factory.hpp>#include <hpx/runtime/serialization/detail/polymorphic_intrusive_factory.hpp>#include <hpx/runtime/serialization/string.hpp>#include <hpx/runtime/serialization/input_container.hpp>#include <hpx/runtime/serialization/binary_filter.hpp>#include <hpx/runtime/serialization/container.hpp>#include <hpx/runtime/naming/name.hpp>#include <hpx/util/spinlock_pool.hpp>#include <hpx/util/fibhash.hpp>#include <boost/version.hpp>#include <hpx/runtime/naming/id_type.hpp>#include <hpx/traits/is_bitwise_serializable.hpp>#include <hpx/util/detail/yield_k.hpp>#include <hpx/runtime/naming/id_type_impl.hpp>#include <hpx/runtime/serialization/serialization_chunk.hpp>#include <cstring>#include <hpx/traits/serialization_access_data.hpp>#include <boost/cstdint.hpp>#include <boost/predef/other/endian.h>#include <hpx/runtime/serialization/output_archive.hpp>#include <hpx/runtime/serialization/output_container.hpp>#include <hpx/runtime/serialization/detail/polymorphic_nonintrusive_factory_impl.hpp>#include <hpx/traits/is_executor_parameters.hpp>#include <hpx/parallel/executors/execution_parameters_fwd.hpp>#include <hpx/util/bind_back.hpp>#include <hpx/util/unwrap.hpp>#include <hpx/util/detail/unwrap_impl.hpp>#include <hpx/util/pack_traversal.hpp>#include <hpx/util/detail/pack_traversal_impl.hpp>#include <hpx/lcos/wait_all.hpp>#include <hpx/traits/detail/wrap_int.hpp>#include <stdexcept>#include <hpx/components/component_storage/server/migrate_from_storage.hpp>#include <hpx/runtime/components/runtime_support.hpp>#include <hpx/runtime/applier/applier.hpp>#include <hpx/runtime/agas_fwd.hpp>#include <hpx/runtime/applier_fwd.hpp>#include <hpx/runtime/components/component_type.hpp>#include <hpx/preprocessor/expand.hpp>#include <hpx/preprocessor/nargs.hpp>#include <hpx/traits/component_type_database.hpp>#include <hpx/util_fwd.hpp>#include <hpx/runtime/naming/address.hpp>#include <hpx/runtime/parcelset/parcel.hpp>#include <hpx/runtime/actions_fwd.hpp>#include <hpx/runtime/parcelset_fwd.hpp>#include <hpx/util/thread_specific_ptr.hpp>#include <boost/thread/tss.hpp>#include <hpx/runtime/components/stubs/runtime_support.hpp>#include <hpx/async.hpp>#include <hpx/lcos/async.hpp>#include <hpx/lcos/detail/async_implementations.hpp>#include <hpx/lcos/detail/async_implementations_fwd.hpp>#include <hpx/lcos/packaged_action.hpp>#include <hpx/lcos/promise.hpp>#include <hpx/lcos/detail/promise_base.hpp>#include <hpx/lcos/detail/promise_lco.hpp>#include <hpx/lcos/base_lco_with_value.hpp>#include <hpx/lcos/base_lco.hpp>#include <hpx/runtime/actions/basic_action.hpp>#include <hpx/lcos/sync_fwd.hpp>#include <hpx/runtime/actions/action_support.hpp>#include <hpx/runtime/components/pinned_ptr.hpp>#include <hpx/runtime/get_lva.hpp>#include <hpx/runtime/components_fwd.hpp>#include <hpx/traits/managed_component_policies.hpp>#include <hpx/traits/action_decorate_function.hpp>#include <hpx/traits/component_pin_support.hpp>#include <hpx/runtime/serialization/base_object.hpp>#include <hpx/runtime/threads/thread_init_data.hpp>#include <hpx/traits/action_remote_result.hpp>#include <hpx/runtime/actions/basic_action_fwd.hpp>#include <hpx/runtime/actions/continuation.hpp>#include <hpx/runtime/actions/action_priority.hpp>#include <hpx/traits/action_priority.hpp>#include <hpx/runtime/actions/trigger.hpp>#include <hpx/runtime/agas/interface.hpp>#include <boost/dynamic_bitset.hpp>#include <hpx/runtime/trigger_lco.hpp>#include <hpx/runtime/applier/detail/apply_implementations_fwd.hpp>#include <hpx/traits/is_continuation.hpp>#include <hpx/util/logging.hpp>#include <hpx/runtime/actions/detail/action_factory.hpp>#include <hpx/runtime/actions/detail/invocation_count_registry.hpp>#include <hpx/performance_counters/counters_fwd.hpp>#include <hpx/runtime/actions/preassigned_action_id.hpp>#include <hpx/runtime/actions/transfer_action.hpp>#include <hpx/runtime/actions/transfer_base_action.hpp>#include <hpx/runtime/actions/base_action.hpp>#include <hpx/runtime/threads/thread_id_type.hpp>#include <hpx/runtime/serialization/unique_ptr.hpp>#include <hpx/traits/action_does_termination_detection.hpp>#include <hpx/traits/action_message_handler.hpp>#include <hpx/traits/action_schedule_thread.hpp>#include <hpx/traits/action_serialization_filter.hpp>#include <hpx/traits/action_stacksize.hpp>#include <hpx/traits/action_was_object_migrated.hpp>#include <hpx/util/get_and_reset_value.hpp>#include <hpx/runtime/applier/apply_helper.hpp>#include <hpx/runtime_fwd.hpp>#include <hpx/runtime/basename_registration_fwd.hpp>#include <hpx/components_fwd.hpp>#include <hpx/runtime/components/make_client.hpp>#include <hpx/traits/is_client.hpp>#include <hpx/runtime/config_entry.hpp>#include <hpx/runtime/find_localities.hpp>#include <hpx/runtime/get_colocation_id.hpp>#include <hpx/runtime/get_locality_id.hpp>#include <hpx/runtime/get_locality_name.hpp>#include <hpx/runtime/get_num_localities.hpp>#include <hpx/runtime/get_thread_name.hpp>#include <hpx/runtime/report_error.hpp>#include <hpx/runtime/runtime_fwd.hpp>#include <hpx/runtime/runtime_mode.hpp>#include <hpx/runtime/set_parcel_write_handler.hpp>#include <hpx/runtime/shutdown_function.hpp>#include <hpx/runtime/startup_function.hpp>#include <hpx/state.hpp>#include <hpx/traits/action_continuation.hpp>#include <hpx/traits/action_decorate_continuation.hpp>#include <hpx/traits/action_select_direct_execution.hpp>#include <hpx/runtime/parcelset/detail/per_action_data_counter_registry.hpp>#include <hpx/runtime/actions/transfer_continuation_action.hpp>#include <hpx/traits/is_distribution_policy.hpp>#include <boost/utility/string_ref.hpp>#include <sstream>#include <hpx/runtime/actions/component_action.hpp>#include <hpx/runtime/components/server/managed_component_base.hpp>#include <hpx/runtime/components/server/create_component_fwd.hpp>#include <hpx/runtime/components/server/component_heap.hpp>#include <hpx/util/reinitializable_static.hpp>#include <hpx/util/bind_front.hpp>#include <hpx/util/static_reinit.hpp>#include <hpx/runtime/components/server/wrapper_heap.hpp>#include <hpx/util/generate_unique_ids.hpp>#include <hpx/util/wrapper_heap_base.hpp>#include <new>#include <hpx/runtime/components/server/wrapper_heap_list.hpp>#include <hpx/util/one_size_heap_list.hpp>#include <hpx/util/unlock_guard.hpp>#include <hpx/plugins/parcel/coalescing_message_handler_registration.hpp>#include <hpx/runtime/components/server/component_base.hpp>#include <hpx/util/ini.hpp>#include <boost/lexical_cast.hpp>#include <hpx/lcos/local/promise.hpp>#include <boost/utility/swap.hpp>#include <hpx/runtime/applier/apply.hpp>#include <hpx/runtime/applier/detail/apply_implementations.hpp>#include <hpx/traits/action_is_target_valid.hpp>#include <hpx/traits/component_supports_migration.hpp>#include <hpx/util/format.hpp>#include <cctype>#include <cstdio>#include <ostream>#include <hpx/runtime/components/client_base.hpp>#include <hpx/runtime/components/stubs/stub_base.hpp>#include <hpx/lcos/detail/async_colocated_fwd.hpp>#include <hpx/runtime/naming/unmanaged.hpp>#include <hpx/runtime/parcelset/detail/parcel_await.hpp>#include <hpx/runtime/parcelset/put_parcel.hpp>#include <hpx/runtime.hpp>#include <hpx/performance_counters/counters.hpp>#include <hpx/runtime/parcelset/locality.hpp>#include <hpx/runtime/serialization/map.hpp>#include <hpx/runtime/thread_hooks.hpp>#include <hpx/util/runtime_configuration.hpp>#include <hpx/runtime/components/static_factory_data.hpp>#include <hpx/util/plugin/export_plugin.hpp>#include <hpx/util/plugin/abstract_factory.hpp>#include <hpx/util/plugin/virtual_constructor.hpp>#include <hpx/util/plugin/config.hpp>#include <boost/any.hpp>#include <boost/shared_ptr.hpp>#include <hpx/util/plugin/concrete_factory.hpp>#include <hpx/util/plugin/plugin_wrapper.hpp>#include <boost/algorithm/string/case_conv.hpp>#include <hpx/util/plugin/dll.hpp>#include <hpx/util/plugin/detail/dll_dlopen.hpp>#include <boost/filesystem/convenience.hpp>#include <boost/filesystem/path.hpp>#include <link.h>#include <dlfcn.h>#include <limits.h>#include <hpx/plugins/plugin_registry_base.hpp>#include <hpx/util/plugin.hpp>#include <hpx/util/plugin/plugin_factory.hpp>#include <boost/filesystem.hpp>#include <set>#include <hpx/runtime/naming/split_gid.hpp>#include <hpx/runtime/parcelset/parcelhandler.hpp>#include <hpx/runtime/parcelset/parcelport.hpp>#include <hpx/performance_counters/parcels/data_point.hpp>#include <hpx/performance_counters/parcels/gatherer.hpp>#include <hpx/lcos/local/no_mutex.hpp>#include <hpx/runtime/parcelset/detail/per_action_data_counter.hpp>#include <hpx/util/high_resolution_timer.hpp>#include <hpx/util/high_resolution_clock.hpp>#include <hpx/plugins/parcelport_factory_base.hpp>#include <hpx/traits/component_type_is_compatible.hpp>#include <hpx/traits/is_valid_action.hpp>#include <hpx/runtime/applier/apply_callback.hpp>#include <boost/asio/error.hpp>#include <hpx/runtime/threads/thread.hpp>#include <hpx/lcos/sync.hpp>#include <hpx/lcos/detail/sync_implementations.hpp>#include <hpx/lcos/detail/sync_implementations_fwd.hpp>#include <hpx/lcos/async_continue.hpp>#include <hpx/lcos/async_continue_fwd.hpp>#include <hpx/util/bind_action.hpp>#include <hpx/runtime/actions/manage_object_action.hpp>#include <hpx/runtime/serialization/array.hpp>#include <boost/array.hpp>#include <hpx/runtime/serialization/serialize_buffer.hpp>#include <hpx/traits/supports_streaming_with_any.hpp>#include <boost/shared_array.hpp>#include <hpx/runtime/components/server/runtime_support.hpp>#include <hpx/compat/condition_variable.hpp>#include <condition_variable>#include <hpx/lcos/local/condition_variable.hpp>#include <hpx/lcos/local/mutex.hpp>#include <hpx/plugins/plugin_factory_base.hpp>#include <hpx/runtime/components/server/create_component.hpp>#include <hpx/runtime/find_here.hpp>#include <boost/program_options/options_description.hpp>#include <hpx/runtime/serialization/vector.hpp>#include <hpx/runtime/serialization/detail/serialize_collection.hpp>#include <hpx/runtime/components/server/migrate_component.hpp>#include <hpx/runtime/actions/plain_action.hpp>#include <hpx/runtime/get_ptr.hpp>#include <hpx/runtime/agas/gva.hpp>#include <boost/io/ios_state.hpp>#include <hpx/components/component_storage/export_definitions.hpp>#include <hpx/config/export_definitions.hpp>#include <hpx/components/component_storage/server/component_storage.hpp>#include <hpx/components/containers/unordered/unordered_map.hpp>#include <hpx/runtime/components/copy_component.hpp>#include <hpx/lcos/detail/async_colocated.hpp>#include <hpx/runtime/agas/primary_namespace.hpp>#include <hpx/runtime/agas/server/primary_namespace.hpp>#include <hpx/runtime/components/server/fixed_component_base.hpp>#include <hpx/runtime/applier/bind_naming_wrappers.hpp>#include <hpx/util/functional/colocated_helpers.hpp>#include <hpx/runtime/components/server/copy_component.hpp>#include <hpx/runtime/components/new.hpp>#include <hpx/runtime/components/default_distribution_policy.hpp>#include <hpx/runtime/serialization/shared_ptr.hpp>#include <hpx/runtime/components/server/distributed_metadata_base.hpp>#include <hpx/runtime/components/server/simple_component_base.hpp>#include <hpx/runtime/components/server/component.hpp>#include <hpx/traits/component_heap_type.hpp>#include <hpx/runtime/serialization/unordered_map.hpp>#include <hpx/components/containers/container_distribution_policy.hpp>#include <hpx/components/containers/unordered/partition_unordered_map_component.hpp>#include <hpx/lcos/reduce.hpp>#include <hpx/runtime/components/component_factory.hpp>#include <hpx/runtime/components/server/locking_hook.hpp>#include <hpx/runtime/threads/coroutines/coroutine.hpp>#include <hpx/runtime/threads/coroutines/coroutine_fwd.hpp>#include <hpx/runtime/threads/coroutines/detail/coroutine_accessor.hpp>#include <hpx/runtime/threads/coroutines/detail/coroutine_impl.hpp>#include <hpx/runtime/threads/coroutines/detail/context_base.hpp>#include <hpx/runtime/threads/coroutines/detail/context_impl.hpp>#include <hpx/runtime/threads/coroutines/detail/swap_context.hpp>#include <hpx/runtime/threads/coroutines/detail/tss.hpp>#include <hpx/runtime/threads/coroutines/detail/coroutine_self.hpp>#include <tuple>#include <hpx/components/containers/unordered/unordered_map_segmented_iterator.hpp>#include <hpx/util/iterator_adaptor.hpp>#include <hpx/util/iterator_facade.hpp>#include <boost/integer.hpp>
-
file
migrate_to_storage.hpp
- #include <hpx/config.hpp>#include <hpx/lcos/future.hpp>#include <hpx/runtime/components/client_base.hpp>#include <hpx/runtime/naming/id_type.hpp>#include <hpx/traits/is_component.hpp>#include <hpx/components/component_storage/component_storage.hpp>#include <hpx/runtime/launch_policy.hpp>#include <hpx/runtime/naming/address.hpp>#include <hpx/runtime/naming/name.hpp>#include <hpx/components/component_storage/server/component_storage.hpp>#include <cstddef>#include <vector>#include <hpx/components/component_storage/server/migrate_to_storage.hpp>#include <hpx/throw_exception.hpp>#include <hpx/util/bind_back.hpp>#include <hpx/components/component_storage/export_definitions.hpp>#include <cstdint>#include <memory>#include <utility>#include <type_traits>
-
file
error.hpp
- #include <hpx/config.hpp>#include <boost/system/error_code.hpp>#include <string>
-
file
error_code.hpp
- #include <hpx/config.hpp>#include <hpx/error.hpp>#include <hpx/exception_fwd.hpp>#include <boost/system/error_code.hpp>#include <exception>#include <stdexcept>#include <string>#include <hpx/throw_exception.hpp>
-
file
exception.hpp
- #include <hpx/config.hpp>#include <hpx/error.hpp>#include <hpx/error_code.hpp>#include <hpx/error.hpp>#include <hpx/exception_fwd.hpp>#include <boost/system/error_code.hpp>#include <exception>#include <stdexcept>#include <string>#include <hpx/throw_exception.hpp>#include <hpx/exception_fwd.hpp>#include <hpx/exception_info.hpp>#include <hpx/error_code.hpp>#include <hpx/util/detail/pack.hpp>#include <cstddef>#include <type_traits>#include <hpx/util/tuple.hpp>#include <hpx/runtime/serialization/detail/non_default_constructible.hpp>#include <memory>#include <hpx/traits/is_bitwise_serializable.hpp>#include <hpx/util/decay.hpp>#include <boost/ref.hpp>#include </hpx/build/docs/hpx/util/functional>#include <utility>#include <boost/array.hpp>#include <array>#include <algorithm>#include <typeinfo>#include <hpx/runtime/naming_fwd.hpp>#include <hpx/runtime/agas_fwd.hpp>#include <hpx/util/function.hpp>#include <cstdint>#include <boost/system/system_error.hpp>#include <hpx/config/warnings_prefix.hpp>#include <hpx/throw_exception.hpp>#include <hpx/config/warnings_suffix.hpp>
-
file
exception_fwd.hpp
- #include <hpx/config.hpp>#include <hpx/error.hpp>#include <hpx/throw_exception.hpp>
-
file
exception_list.hpp
- #include <hpx/config.hpp>#include <hpx/exception.hpp>#include <hpx/error.hpp>#include <hpx/error_code.hpp>#include <hpx/exception_fwd.hpp>#include <hpx/exception_info.hpp>#include <hpx/runtime/naming_fwd.hpp>#include <boost/system/error_code.hpp>#include <boost/system/system_error.hpp>#include <cstddef>#include <cstdint>#include <exception>#include <string>#include <hpx/config/warnings_prefix.hpp>#include <hpx/throw_exception.hpp>#include <hpx/config/warnings_suffix.hpp>#include <hpx/lcos/local/spinlock.hpp>#include <hpx/runtime/threads/thread_helpers.hpp>#include <hpx/runtime/threads_fwd.hpp>#include <hpx/runtime/threads/thread_data_fwd.hpp>#include <hpx/runtime/threads/coroutines/coroutine_fwd.hpp>#include <hpx/runtime/threads/thread_enums.hpp>#include <hpx/runtime/threads/detail/combined_tagged_state.hpp>#include <hpx/util/assert.hpp>#include <hpx/runtime/threads/thread_id_type.hpp>#include <hpx/config/constexpr.hpp>#include <hpx/config/export_definitions.hpp>#include <functional>#include <iosfwd>#include <hpx/util_fwd.hpp>#include <hpx/util/function.hpp>#include <hpx/util/unique_function.hpp>#include <utility>#include <memory>#include <hpx/runtime/thread_pool_helpers.hpp>#include <hpx/runtime/threads/policies/scheduler_mode.hpp>#include <hpx/util/register_locks.hpp>#include <hpx/traits/has_member_xxx.hpp>#include <hpx/preprocessor/cat.hpp>#include <type_traits>#include <hpx/util/steady_clock.hpp>#include <chrono>#include <hpx/util/thread_description.hpp>#include <hpx/runtime/actions/basic_action_fwd.hpp>#include <hpx/runtime/actions/preassigned_action_id.hpp>#include <hpx/traits/get_function_address.hpp>#include <hpx/traits/get_function_annotation.hpp>#include <hpx/traits/is_action.hpp>#include <hpx/util/always_void.hpp>#include <hpx/util/decay.hpp>#include <atomic>#include <hpx/util/detail/yield_k.hpp>#include <sched.h>#include <time.h>#include <hpx/util/itt_notify.hpp>#include <boost/smart_ptr/detail/spinlock.hpp>#include <list>#include <mutex>
-
file
hpx_finalize.hpp
- #include <hpx/config.hpp>#include <hpx/exception_fwd.hpp>
-
file
hpx_init.hpp
- #include <hpx/config.hpp>#include <hpx/config/attributes.hpp>#include <hpx/config/defines.hpp>#include <hpx/config/compiler_specific.hpp>#include <hpx/config/branch_hints.hpp>#include <hpx/config/compiler_fence.hpp>#include <hpx/config/compiler_native_tls.hpp>#include <ciso646>#include <hpx/config/constexpr.hpp>#include <hpx/config/debug.hpp>#include <hpx/config/emulate_deleted.hpp>#include <hpx/config/export_definitions.hpp>#include <hpx/config/forceinline.hpp>#include <hpx/config/lambda_capture.hpp>#include <utility>#include <hpx/config/manual_profiling.hpp>#include <hpx/config/threads_stack.hpp>#include <hpx/config/version.hpp>#include <hpx/config/weak_symbol.hpp>#include <boost/version.hpp>#include <hpx/preprocessor/cat.hpp>#include <hpx/preprocessor/stringize.hpp>#include <hpx/hpx_finalize.hpp>#include <hpx/exception_fwd.hpp>#include <hpx/error.hpp>#include <boost/system/error_code.hpp>#include <string>#include <hpx/throw_exception.hpp>#include <hpx/preprocessor/expand.hpp>#include <hpx/preprocessor/nargs.hpp>#include <boost/current_function.hpp>#include <exception>#include <hpx/config/warnings_prefix.hpp>#include <hpx/config/warnings_suffix.hpp>#include <hpx/hpx_suspend.hpp>#include <hpx/runtime/runtime_mode.hpp>#include <hpx/runtime/shutdown_function.hpp>#include <hpx/util/unique_function.hpp>#include <hpx/runtime/serialization/serialization_fwd.hpp>#include <hpx/preprocessor/strip_parens.hpp>#include <type_traits>#include <hpx/traits/get_function_address.hpp>#include <cstddef>#include <memory>#include <hpx/traits/get_function_annotation.hpp>#include <hpx/util/itt_notify.hpp>#include <cstdint>#include <cstring>#include <hpx/traits/is_callable.hpp>#include <hpx/util/always_void.hpp>#include <hpx/util/result_of.hpp>#include <boost/ref.hpp>#include <hpx/util/detail/basic_function.hpp>#include <hpx/util/assert.hpp>#include <assert.h>#include <cstdlib>#include <iostream>#include <hpx/util/detail/empty_function.hpp>#include <hpx/util/detail/vtable/vtable.hpp>#include <hpx/util/detail/vtable/function_vtable.hpp>#include <hpx/util/detail/vtable/callable_vtable.hpp>#include <hpx/util/invoke.hpp>#include <hpx/util/void_guard.hpp>#include </hpx/build/docs/hpx/util/functional>#include <hpx/util/detail/vtable/copyable_vtable.hpp>#include <new>#include <hpx/util/detail/vtable/serializable_function_vtable.hpp>#include <hpx/runtime/serialization/detail/polymorphic_intrusive_factory.hpp>#include <hpx/util/debug/demangle_helper.hpp>#include <typeinfo>#include <hpx/util/jenkins_hash.hpp>#include <random>#include <unordered_map>#include <hpx/util/detail/function_registration.hpp>#include <hpx/util/detail/vtable/serializable_vtable.hpp>#include <hpx/util_fwd.hpp>#include <hpx/runtime/startup_function.hpp>#include <hpx/util/function.hpp>#include <boost/program_options/options_description.hpp>#include <boost/program_options/variables_map.hpp>#include <vector>
-
file
hpx_start.hpp
- #include <hpx/config.hpp>#include <hpx/hpx_finalize.hpp>#include <hpx/runtime/runtime_mode.hpp>#include <hpx/runtime/shutdown_function.hpp>#include <hpx/runtime/startup_function.hpp>#include <hpx/util/function.hpp>#include <boost/program_options/options_description.hpp>#include <boost/program_options/variables_map.hpp>#include <cstddef>#include <string>#include <vector>
-
file
hpx_suspend.hpp
- #include <hpx/exception_fwd.hpp>
-
file
barrier.hpp
- #include <hpx/config.hpp>#include <hpx/lcos/future.hpp>#include <hpx/runtime/components/server/managed_component_base.hpp>#include <hpx/runtime/launch_policy.hpp>#include <boost/intrusive_ptr.hpp>#include <cstddef>#include <string>#include <utility>#include <vector>#include <hpx/config/warnings_prefix.hpp>#include <hpx/config/warnings_suffix.hpp>
-
file
broadcast.hpp
-
file
fold.hpp
-
file
gather.hpp
Defines
-
HPX_REGISTER_GATHER_DECLARATION
(type, name)¶ Declare a gather object named name for a given data type type.
The macro HPX_REGISTER_GATHER_DECLARATION can be used to declare all facilities necessary for a (possibly remote) gather operation.
The parameter type specifies for which data type the gather operations should be enabled.
The (optional) parameter name should be a unique C-style identifier which will be internally used to identify a particular gather operation. If this defaults to <type>_gather if not specified.
- Note
- The macro HPX_REGISTER_GATHER_DECLARATION can be used with 1 or 2 arguments. The second argument is optional and defaults to <type>_gather.
-
HPX_REGISTER_GATHER
(type, name)¶ Define a gather object named name for a given data type type.
The macro HPX_REGISTER_GATHER can be used to define all facilities necessary for a (possibly remote) gather operation.
The parameter type specifies for which data type the gather operations should be enabled.
The (optional) parameter name should be a unique C-style identifier which will be internally used to identify a particular gather operation. If this defaults to <type>_gather if not specified.
- Note
- The macro HPX_REGISTER_GATHER can be used with 1 or 2 arguments. The second argument is optional and defaults to <type>_gather.
-
-
file
split_future.hpp
-
file
wait_all.hpp
-
file
wait_any.hpp
-
file
wait_each.hpp
-
file
wait_some.hpp
-
file
when_all.hpp
-
file
when_any.hpp
-
file
when_each.hpp
-
file
when_some.hpp
-
file
algorithm.hpp
- #include <hpx/config.hpp>#include <algorithm>#include <hpx/parallel/algorithms/adjacent_find.hpp>#include <hpx/traits/is_iterator.hpp>#include <hpx/parallel/algorithms/detail/dispatch.hpp>#include <hpx/lcos/future.hpp>#include <hpx/runtime/serialization/serialization_fwd.hpp>#include <hpx/throw_exception.hpp>#include <hpx/traits/segmented_iterator_traits.hpp>#include <hpx/util/decay.hpp>#include <type_traits>#include <utility>#include <hpx/parallel/exception_list.hpp>#include <hpx/parallel/execution_policy.hpp>#include <hpx/parallel/datapar/execution_policy.hpp>#include <hpx/parallel/execution_policy_fwd.hpp>#include <hpx/parallel/executors/execution.hpp>#include <hpx/parallel/executors/execution_parameters.hpp>#include <hpx/parallel/executors/parallel_executor.hpp>#include <hpx/parallel/executors/rebind_executor.hpp>#include <hpx/parallel/executors/sequenced_executor.hpp>#include <hpx/runtime/serialization/serialize.hpp>#include <hpx/traits/executor_traits.hpp>#include <hpx/traits/is_execution_policy.hpp>#include <hpx/traits/is_executor.hpp>#include <hpx/traits/is_executor_parameters.hpp>#include <hpx/traits/is_launch_policy.hpp>#include <memory>#include <hpx/parallel/util/detail/algorithm_result.hpp>#include <hpx/traits/concepts.hpp>#include <hpx/util/invoke.hpp>#include <hpx/util/unused.hpp>#include <hpx/parallel/util/detail/scoped_executor_parameters.hpp>#include <hpx/util/tuple.hpp>#include <string>#include <hpx/parallel/algorithms/detail/predicates.hpp>#include <hpx/parallel/util/loop.hpp>#include <hpx/parallel/util/cancellation_token.hpp>#include <atomic>#include <functional>#include <hpx/parallel/util/projection_identity.hpp>#include <hpx/util/assert.hpp>#include <hpx/util/result_of.hpp>#include <cstddef>#include <iterator>#include <vector>#include <hpx/parallel/util/partitioner.hpp>#include <hpx/dataflow.hpp>#include <hpx/lcos/dataflow.hpp>#include <hpx/exception_list.hpp>#include <hpx/lcos/wait_all.hpp>#include <hpx/util/range.hpp>#include <hpx/parallel/util/detail/chunk_size.hpp>#include <hpx/util/iterator_range.hpp>#include <hpx/traits/is_range.hpp>#include <hpx/parallel/algorithms/detail/is_negative.hpp>#include <hpx/parallel/executors/execution_information.hpp>#include <hpx/runtime/threads/topology.hpp>#include <hpx/traits/detail/wrap_int.hpp>#include <hpx/parallel/executors/execution_information_fwd.hpp>#include <hpx/parallel/executors/execution_fwd.hpp>#include <hpx/runtime/threads/thread_data_fwd.hpp>#include <hpx/parallel/util/detail/chunk_size_iterator.hpp>#include <hpx/util/min.hpp>#include <hpx/util/iterator_facade.hpp>#include <hpx/parallel/util/detail/handle_local_exceptions.hpp>#include <hpx/async.hpp>#include <hpx/hpx_finalize.hpp>#include <exception>#include <list>#include <hpx/parallel/util/detail/partitioner_iteration.hpp>#include <hpx/util/invoke_fused.hpp>#include <hpx/parallel/util/detail/select_partitioner.hpp>#include <hpx/parallel/util/zip_iterator.hpp>#include <hpx/util/tagged_pair.hpp>#include <hpx/util/tagged.hpp>#include <hpx/util/detail/pack.hpp>#include </hpx/build/docs/hpx/util/functional>#include <hpx/util/zip_iterator.hpp>#include <hpx/runtime/naming/id_type.hpp>#include <hpx/util/functional/segmented_iterator_helpers.hpp>#include <hpx/parallel/algorithms/all_any_none.hpp>#include <hpx/util/void_guard.hpp>#include <hpx/parallel/traits/projected.hpp>#include <hpx/parallel/traits/vector_pack_load_store.hpp>#include <hpx/parallel/traits/vector_pack_type.hpp>#include <hpx/traits/is_callable.hpp>#include <hpx/util/always_void.hpp>#include <hpx/parallel/util/invoke_projected.hpp>#include <hpx/parallel/algorithms/copy.hpp>#include <hpx/parallel/algorithms/detail/transfer.hpp>#include <hpx/parallel/segmented_algorithms/detail/transfer.hpp>#include <hpx/parallel/segmented_algorithms/detail/dispatch.hpp>#include <hpx/runtime/actions/plain_action.hpp>#include <hpx/runtime/components/colocating_distribution_policy.hpp>#include <hpx/lcos/detail/async_colocated.hpp>#include <hpx/lcos/detail/async_colocated_callback.hpp>#include <hpx/lcos/async_continue_callback.hpp>#include <hpx/lcos/async_callback_fwd.hpp>#include <hpx/lcos/async_fwd.hpp>#include <hpx/runtime/actions/basic_action_fwd.hpp>#include <hpx/runtime/launch_policy.hpp>#include <hpx/lcos/async_continue.hpp>#include <hpx/runtime/applier/apply_callback.hpp>#include <hpx/traits/extract_action.hpp>#include <hpx/traits/is_distribution_policy.hpp>#include <hpx/traits/promise_local_result.hpp>#include <hpx/traits/promise_remote_result.hpp>#include <hpx/lcos/detail/async_colocated_callback_fwd.hpp>#include <hpx/lcos/detail/async_colocated_fwd.hpp>#include <hpx/runtime/agas/primary_namespace.hpp>#include <hpx/runtime/agas/server/primary_namespace.hpp>#include <hpx/lcos/detail/async_implementations.hpp>#include <hpx/runtime/applier/detail/apply_colocated_callback_fwd.hpp>#include <hpx/runtime/actions/action_support.hpp>#include <hpx/runtime/applier/detail/apply_colocated_fwd.hpp>#include <hpx/traits/is_continuation.hpp>#include <hpx/runtime/applier/detail/apply_implementations.hpp>#include <hpx/runtime/components/client_base.hpp>#include <hpx/runtime/components/stubs/stub_base.hpp>#include <hpx/runtime/find_here.hpp>#include <hpx/runtime/naming/name.hpp>#include <hpx/parallel/util/detail/handle_remote_exceptions.hpp>#include <hpx/parallel/tagspec.hpp>#include <hpx/parallel/util/foreach_partitioner.hpp>#include <hpx/parallel/util/scan_partitioner.hpp>#include <hpx/parallel/util/transfer.hpp>#include <hpx/traits/pointer_category.hpp>#include <cstring>#include <boost/shared_array.hpp>#include <hpx/parallel/algorithms/count.hpp>#include <hpx/util/bind_back.hpp>#include <hpx/util/unwrap.hpp>#include <hpx/parallel/algorithms/detail/distance.hpp>#include <hpx/parallel/traits/vector_pack_count_bits.hpp>#include <hpx/parallel/algorithms/equal.hpp>#include <hpx/parallel/algorithms/fill.hpp>#include <hpx/traits/is_value_proxy.hpp>#include <hpx/parallel/algorithms/for_each.hpp>#include <hpx/util/annotated_function.hpp>#include <hpx/util/identity.hpp>#include <cstdint>#include <hpx/parallel/algorithms/find.hpp>#include <hpx/parallel/util/compare_projected.hpp>#include <hpx/parallel/algorithms/for_each.hpp>#include <hpx/parallel/algorithms/generate.hpp>#include <hpx/parallel/algorithms/includes.hpp>#include <hpx/parallel/algorithms/is_heap.hpp>#include <hpx/parallel/algorithms/is_partitioned.hpp>#include <hpx/parallel/algorithms/is_sorted.hpp>#include <hpx/parallel/algorithms/lexicographical_compare.hpp>#include <hpx/parallel/algorithms/mismatch.hpp>#include <hpx/parallel/algorithms/merge.hpp>#include <hpx/util/tagged_tuple.hpp>#include <hpx/parallel/algorithms/minmax.hpp>#include <hpx/parallel/algorithms/mismatch.hpp>#include <hpx/parallel/algorithms/move.hpp>#include <hpx/parallel/algorithms/partition.hpp>#include <hpx/lcos/local/spinlock.hpp>#include <hpx/parallel/algorithms/remove.hpp>#include <hpx/parallel/algorithms/remove_copy.hpp>#include <hpx/parallel/algorithms/copy.hpp>#include <hpx/parallel/algorithms/replace.hpp>#include <hpx/parallel/algorithms/reverse.hpp>#include <hpx/parallel/algorithms/rotate.hpp>#include <hpx/parallel/algorithms/reverse.hpp>#include <hpx/parallel/algorithms/search.hpp>#include <hpx/parallel/algorithms/set_difference.hpp>#include <hpx/parallel/algorithms/detail/set_operation.hpp>#include <hpx/parallel/algorithms/set_intersection.hpp>#include <hpx/parallel/algorithms/set_symmetric_difference.hpp>#include <hpx/parallel/algorithms/set_union.hpp>#include <hpx/parallel/algorithms/sort.hpp>#include <hpx/parallel/algorithms/swap_ranges.hpp>#include <hpx/parallel/algorithms/unique.hpp>#include <hpx/parallel/algorithms/for_loop.hpp>#include <hpx/parallel/algorithms/for_loop_induction.hpp>#include <cstdlib>#include <hpx/parallel/algorithms/for_loop_reduction.hpp>#include <hpx/runtime/get_os_thread_count.hpp>#include <hpx/runtime/get_worker_thread_num.hpp>#include <hpx/util/cache_aligned_data.hpp>
-
file
adjacent_difference.hpp
- #include <hpx/config.hpp>#include <hpx/traits/is_iterator.hpp>#include <hpx/util/zip_iterator.hpp>#include <hpx/parallel/algorithms/detail/dispatch.hpp>#include <hpx/parallel/execution_policy.hpp>#include <hpx/parallel/util/detail/algorithm_result.hpp>#include <hpx/parallel/util/loop.hpp>#include <hpx/parallel/util/partitioner.hpp>#include <hpx/util/unused.hpp>#include <algorithm>#include <cstddef>#include <iterator>#include <numeric>#include <type_traits>#include <utility>#include <vector>
-
file
adjacent_find.hpp
- #include <hpx/config.hpp>#include <hpx/traits/is_iterator.hpp>#include <hpx/parallel/algorithms/detail/dispatch.hpp>#include <hpx/parallel/algorithms/detail/predicates.hpp>#include <hpx/parallel/execution_policy.hpp>#include <hpx/parallel/util/detail/algorithm_result.hpp>#include <hpx/parallel/util/loop.hpp>#include <hpx/parallel/util/partitioner.hpp>#include <hpx/parallel/util/zip_iterator.hpp>#include <algorithm>#include <cstddef>#include <iterator>#include <type_traits>#include <utility>#include <vector>
-
file
all_any_none.hpp
- #include <hpx/config.hpp>#include <hpx/traits/is_iterator.hpp>#include <hpx/util/range.hpp>#include <hpx/util/void_guard.hpp>#include <hpx/parallel/algorithms/detail/dispatch.hpp>#include <hpx/parallel/execution_policy.hpp>#include <hpx/parallel/traits/projected.hpp>#include <hpx/parallel/util/detail/algorithm_result.hpp>#include <hpx/parallel/util/invoke_projected.hpp>#include <hpx/parallel/util/loop.hpp>#include <hpx/parallel/util/partitioner.hpp>#include <hpx/util/unused.hpp>#include <algorithm>#include <cstddef>#include <iterator>#include <type_traits>#include <utility>#include <vector>
-
file
all_any_none.hpp
- #include <hpx/config.hpp>#include <hpx/traits/concepts.hpp>#include <hpx/traits/is_range.hpp>#include <hpx/util/range.hpp>#include <hpx/parallel/algorithms/all_any_none.hpp>#include <hpx/parallel/traits/projected_range.hpp>#include <hpx/util/decay.hpp>#include <hpx/util/result_of.hpp>#include <hpx/parallel/traits/projected.hpp>#include <iterator>#include <type_traits>#include <hpx/parallel/util/projection_identity.hpp>#include <utility>
-
file
copy.hpp
- #include <hpx/config.hpp>#include <hpx/traits/concepts.hpp>#include <hpx/traits/is_iterator.hpp>#include <hpx/util/assert.hpp>#include <hpx/util/invoke.hpp>#include <hpx/util/tagged_pair.hpp>#include <hpx/parallel/algorithms/detail/dispatch.hpp>#include <hpx/parallel/algorithms/detail/is_negative.hpp>#include <hpx/parallel/algorithms/detail/predicates.hpp>#include <hpx/parallel/algorithms/detail/transfer.hpp>#include <hpx/parallel/execution_policy.hpp>#include <hpx/parallel/tagspec.hpp>#include <hpx/parallel/traits/projected.hpp>#include <hpx/parallel/util/detail/algorithm_result.hpp>#include <hpx/parallel/util/foreach_partitioner.hpp>#include <hpx/parallel/util/loop.hpp>#include <hpx/parallel/util/projection_identity.hpp>#include <hpx/parallel/util/scan_partitioner.hpp>#include <hpx/parallel/util/transfer.hpp>#include <hpx/parallel/util/zip_iterator.hpp>#include <hpx/util/unused.hpp>#include <algorithm>#include <cstddef>#include <cstring>#include <iterator>#include <memory>#include <type_traits>#include <utility>#include <vector>#include <boost/shared_array.hpp>
-
file
copy.hpp
- #include <hpx/config.hpp>#include <hpx/traits/concepts.hpp>#include <hpx/traits/is_iterator.hpp>#include <hpx/traits/is_range.hpp>#include <hpx/util/range.hpp>#include <hpx/util/tagged_pair.hpp>#include <hpx/parallel/algorithms/copy.hpp>#include <hpx/parallel/traits/projected.hpp>#include <hpx/parallel/traits/projected_range.hpp>#include <type_traits>#include <utility>
-
file
count.hpp
- #include <hpx/config.hpp>#include <hpx/traits/is_iterator.hpp>#include <hpx/traits/segmented_iterator_traits.hpp>#include <hpx/util/bind_back.hpp>#include <hpx/util/range.hpp>#include <hpx/util/unwrap.hpp>#include <hpx/parallel/algorithms/detail/dispatch.hpp>#include <hpx/parallel/algorithms/detail/distance.hpp>#include <hpx/parallel/execution_policy.hpp>#include <hpx/parallel/traits/projected.hpp>#include <hpx/parallel/traits/vector_pack_count_bits.hpp>#include <hpx/parallel/util/detail/algorithm_result.hpp>#include <hpx/parallel/util/invoke_projected.hpp>#include <hpx/parallel/util/loop.hpp>#include <hpx/parallel/util/partitioner.hpp>#include <algorithm>#include <cstddef>#include <functional>#include <iterator>#include <type_traits>#include <utility>#include <vector>
-
file
count.hpp
- #include <hpx/config.hpp>#include <hpx/traits/concepts.hpp>#include <hpx/traits/is_range.hpp>#include <hpx/util/range.hpp>#include <hpx/parallel/algorithms/count.hpp>#include <hpx/parallel/traits/projected_range.hpp>#include <hpx/parallel/util/projection_identity.hpp>#include <type_traits>#include <utility>
-
file
destroy.hpp
- #include <hpx/config.hpp>#include <hpx/traits/is_iterator.hpp>#include <hpx/util/void_guard.hpp>#include <hpx/parallel/algorithms/detail/dispatch.hpp>#include <hpx/parallel/algorithms/detail/is_negative.hpp>#include <hpx/parallel/execution_policy.hpp>#include <hpx/parallel/util/detail/algorithm_result.hpp>#include <hpx/parallel/util/loop.hpp>#include <hpx/parallel/util/foreach_partitioner.hpp>#include <hpx/parallel/util/projection_identity.hpp>#include <algorithm>#include <cstddef>#include <iterator>#include <memory>#include <type_traits>#include <utility>#include <vector>
-
file
equal.hpp
- #include <hpx/config.hpp>#include <hpx/traits/is_iterator.hpp>#include <hpx/util/range.hpp>#include <hpx/parallel/algorithms/detail/dispatch.hpp>#include <hpx/parallel/algorithms/detail/predicates.hpp>#include <hpx/parallel/execution_policy.hpp>#include <hpx/parallel/util/detail/algorithm_result.hpp>#include <hpx/parallel/util/loop.hpp>#include <hpx/parallel/util/partitioner.hpp>#include <hpx/parallel/util/zip_iterator.hpp>#include <hpx/util/unused.hpp>#include <algorithm>#include <cstddef>#include <iterator>#include <type_traits>#include <utility>#include <vector>
-
file
exclusive_scan.hpp
- #include <hpx/config.hpp>#include <hpx/traits/is_iterator.hpp>#include <hpx/util/invoke.hpp>#include <hpx/util/unwrap.hpp>#include <hpx/util/zip_iterator.hpp>#include <hpx/parallel/algorithms/detail/dispatch.hpp>#include <hpx/parallel/algorithms/inclusive_scan.hpp>#include <hpx/util/invoke.hpp>#include <hpx/util/unwrap.hpp>#include <hpx/parallel/execution_policy.hpp>#include <hpx/parallel/util/detail/algorithm_result.hpp>#include <hpx/parallel/util/loop.hpp>#include <hpx/parallel/util/partitioner.hpp>#include <hpx/parallel/util/scan_partitioner.hpp>#include <hpx/parallel/util/projection_identity.hpp>#include <hpx/util/unused.hpp>#include <algorithm>#include <cstddef>#include <iterator>#include <numeric>#include <type_traits>#include <utility>#include <vector>#include <hpx/parallel/execution_policy.hpp>
-
file
fill.hpp
- #include <hpx/config.hpp>#include <hpx/traits/is_iterator.hpp>#include <hpx/traits/is_value_proxy.hpp>#include <hpx/util/void_guard.hpp>#include <hpx/parallel/algorithms/for_each.hpp>#include <hpx/parallel/algorithms/detail/dispatch.hpp>#include <hpx/parallel/algorithms/detail/is_negative.hpp>#include <hpx/parallel/execution_policy.hpp>#include <hpx/parallel/util/detail/algorithm_result.hpp>#include <hpx/parallel/util/projection_identity.hpp>#include <algorithm>#include <cstddef>#include <iterator>#include <type_traits>#include <utility>
-
file
fill.hpp
- #include <hpx/config.hpp>#include <hpx/traits/is_execution_policy.hpp>#include <hpx/traits/is_range.hpp>#include <hpx/util/range.hpp>#include <hpx/parallel/algorithms/fill.hpp>#include <type_traits>#include <utility>
-
file
find.hpp
- #include <hpx/config.hpp>#include <hpx/traits/is_iterator.hpp>#include <hpx/util/invoke.hpp>#include <hpx/parallel/algorithms/detail/dispatch.hpp>#include <hpx/parallel/algorithms/detail/predicates.hpp>#include <hpx/parallel/execution_policy.hpp>#include <hpx/parallel/traits/projected.hpp>#include <hpx/parallel/util/compare_projected.hpp>#include <hpx/parallel/util/invoke_projected.hpp>#include <hpx/parallel/util/detail/algorithm_result.hpp>#include <hpx/parallel/util/projection_identity.hpp>#include <hpx/parallel/util/loop.hpp>#include <hpx/parallel/util/partitioner.hpp>#include <algorithm>#include <cstddef>#include <iterator>#include <type_traits>#include <utility>#include <vector>
-
file
find.hpp
- #include <hpx/config.hpp>#include <hpx/traits/concepts.hpp>#include <hpx/traits/is_execution_policy.hpp>#include <hpx/traits/is_range.hpp>#include <hpx/util/range.hpp>#include <hpx/parallel/algorithms/find.hpp>#include <hpx/parallel/traits/projected.hpp>#include <hpx/parallel/traits/projected_range.hpp>#include <hpx/parallel/util/projection_identity.hpp>#include <type_traits>#include <utility>
-
file
for_each.hpp
- #include <hpx/config.hpp>#include <hpx/traits/concepts.hpp>#include <hpx/traits/is_callable.hpp>#include <hpx/traits/is_iterator.hpp>#include <hpx/traits/is_value_proxy.hpp>#include <hpx/traits/segmented_iterator_traits.hpp>#include <hpx/util/annotated_function.hpp>#include <hpx/util/identity.hpp>#include <hpx/util/invoke.hpp>#include <hpx/parallel/algorithms/detail/dispatch.hpp>#include <hpx/parallel/algorithms/detail/is_negative.hpp>#include <hpx/parallel/execution_policy.hpp>#include <hpx/parallel/traits/projected.hpp>#include <hpx/parallel/util/detail/algorithm_result.hpp>#include <hpx/parallel/util/foreach_partitioner.hpp>#include <hpx/parallel/util/loop.hpp>#include <hpx/parallel/util/projection_identity.hpp>#include <algorithm>#include <cstddef>#include <cstdint>#include <iterator>#include <type_traits>#include <utility>
-
file
for_each.hpp
- #include <hpx/config.hpp>#include <hpx/traits/concepts.hpp>#include <hpx/traits/is_range.hpp>#include <hpx/util/range.hpp>#include <hpx/parallel/algorithms/for_each.hpp>#include <hpx/parallel/traits/projected_range.hpp>#include <hpx/parallel/util/projection_identity.hpp>#include <type_traits>#include <utility>
-
file
for_loop.hpp
- #include <hpx/config.hpp>#include <hpx/traits/concepts.hpp>#include <hpx/traits/is_iterator.hpp>#include <hpx/util/annotated_function.hpp>#include <hpx/util/assert.hpp>#include <hpx/util/decay.hpp>#include <hpx/util/detail/pack.hpp>#include <hpx/util/invoke.hpp>#include <hpx/util/tuple.hpp>#include <hpx/util/unused.hpp>#include <hpx/parallel/algorithms/detail/dispatch.hpp>#include <hpx/parallel/algorithms/detail/predicates.hpp>#include <hpx/parallel/algorithms/for_loop_induction.hpp>#include <hpx/parallel/algorithms/for_loop_reduction.hpp>#include <hpx/parallel/execution_policy.hpp>#include <hpx/parallel/util/detail/algorithm_result.hpp>#include <hpx/parallel/util/loop.hpp>#include <hpx/parallel/util/partitioner.hpp>#include <algorithm>#include <cstddef>#include <cstdint>#include <iterator>#include <type_traits>#include <utility>#include <vector>
-
file
for_loop_induction.hpp
- #include <hpx/config.hpp>#include <hpx/util/decay.hpp>#include <hpx/parallel/algorithms/detail/predicates.hpp>#include <cstddef>#include <cstdlib>#include <type_traits>#include <utility>
-
file
for_loop_reduction.hpp
- #include <hpx/config.hpp>#include <hpx/runtime/get_os_thread_count.hpp>#include <hpx/runtime/get_worker_thread_num.hpp>#include <hpx/util/assert.hpp>#include <hpx/util/decay.hpp>#include <hpx/util/cache_aligned_data.hpp>#include <hpx/parallel/algorithms/detail/predicates.hpp>#include <boost/shared_array.hpp>#include <cstddef>#include <cstdlib>#include <functional>#include <type_traits>#include <utility>
-
file
generate.hpp
- #include <hpx/config.hpp>#include <hpx/traits/concepts.hpp>#include <hpx/traits/is_iterator.hpp>#include <hpx/traits/segmented_iterator_traits.hpp>#include <hpx/parallel/algorithms/detail/dispatch.hpp>#include <hpx/parallel/algorithms/detail/is_negative.hpp>#include <hpx/parallel/algorithms/for_each.hpp>#include <hpx/parallel/execution_policy.hpp>#include <hpx/parallel/util/detail/algorithm_result.hpp>#include <hpx/parallel/util/projection_identity.hpp>#include <algorithm>#include <cstddef>#include <iterator>#include <type_traits>#include <utility>
-
file
generate.hpp
- #include <hpx/config.hpp>#include <hpx/traits/concepts.hpp>#include <hpx/traits/is_range.hpp>#include <hpx/util/range.hpp>#include <hpx/parallel/algorithms/generate.hpp>#include <hpx/parallel/traits/projected_range.hpp>#include <hpx/parallel/util/projection_identity.hpp>#include <type_traits>#include <utility>
-
file
includes.hpp
- #include <hpx/config.hpp>#include <hpx/traits/is_iterator.hpp>#include <hpx/util/range.hpp>#include <hpx/parallel/algorithms/detail/dispatch.hpp>#include <hpx/parallel/algorithms/detail/predicates.hpp>#include <hpx/parallel/execution_policy.hpp>#include <hpx/parallel/util/cancellation_token.hpp>#include <hpx/parallel/util/detail/algorithm_result.hpp>#include <hpx/parallel/util/partitioner.hpp>#include <algorithm>#include <cstddef>#include <iterator>#include <type_traits>#include <utility>#include <vector>
-
file
inclusive_scan.hpp
- #include <hpx/config.hpp>#include <hpx/traits/is_iterator.hpp>#include <hpx/util/invoke.hpp>#include <hpx/util/unwrap.hpp>#include <hpx/util/zip_iterator.hpp>#include <hpx/parallel/algorithms/detail/dispatch.hpp>#include <hpx/parallel/execution_policy.hpp>#include <hpx/parallel/util/detail/algorithm_result.hpp>#include <hpx/parallel/util/loop.hpp>#include <hpx/parallel/util/partitioner.hpp>#include <hpx/parallel/util/scan_partitioner.hpp>#include <hpx/parallel/util/projection_identity.hpp>#include <hpx/util/unused.hpp>#include <algorithm>#include <cstddef>#include <iterator>#include <numeric>#include <type_traits>#include <utility>#include <vector>
-
file
is_heap.hpp
- #include <hpx/config.hpp>#include <hpx/async.hpp>#include <hpx/lcos/future.hpp>#include <hpx/traits/concepts.hpp>#include <hpx/traits/is_callable.hpp>#include <hpx/traits/is_iterator.hpp>#include <hpx/util/invoke.hpp>#include <hpx/parallel/algorithms/detail/dispatch.hpp>#include <hpx/parallel/execution_policy.hpp>#include <hpx/parallel/executors/execution.hpp>#include <hpx/parallel/traits/projected.hpp>#include <hpx/parallel/util/detail/algorithm_result.hpp>#include <hpx/parallel/util/projection_identity.hpp>#include <hpx/parallel/util/loop.hpp>#include <hpx/parallel/util/partitioner.hpp>#include <algorithm>#include <cstddef>#include <iterator>#include <list>#include <vector>#include <type_traits>#include <utility>
-
file
is_heap.hpp
- #include <hpx/config.hpp>#include <hpx/traits/concepts.hpp>#include <hpx/traits/is_range.hpp>#include <hpx/util/range.hpp>#include <hpx/parallel/algorithms/is_heap.hpp>#include <hpx/parallel/traits/projected_range.hpp>#include <hpx/parallel/util/projection_identity.hpp>#include <type_traits>#include <utility>
-
file
is_partitioned.hpp
- #include <hpx/config.hpp>#include <hpx/lcos/future.hpp>#include <hpx/traits/is_iterator.hpp>#include <hpx/util/invoke.hpp>#include <hpx/util/unused.hpp>#include <hpx/parallel/algorithms/detail/dispatch.hpp>#include <hpx/parallel/execution_policy.hpp>#include <hpx/parallel/util/cancellation_token.hpp>#include <hpx/parallel/util/detail/algorithm_result.hpp>#include <hpx/parallel/util/loop.hpp>#include <hpx/parallel/util/partitioner.hpp>#include <algorithm>#include <cstddef>#include <functional>#include <iterator>#include <type_traits>#include <utility>#include <vector>
-
file
is_sorted.hpp
- #include <hpx/config.hpp>#include <hpx/traits/is_iterator.hpp>#include <hpx/util/invoke.hpp>#include <hpx/util/range.hpp>#include <hpx/parallel/algorithms/detail/dispatch.hpp>#include <hpx/parallel/execution_policy.hpp>#include <hpx/parallel/util/cancellation_token.hpp>#include <hpx/parallel/util/detail/algorithm_result.hpp>#include <hpx/parallel/util/loop.hpp>#include <hpx/parallel/util/partitioner.hpp>#include <hpx/util/unused.hpp>#include <algorithm>#include <cstddef>#include <functional>#include <iterator>#include <type_traits>#include <utility>#include <vector>
-
file
lexicographical_compare.hpp
- #include <hpx/config.hpp>#include <hpx/traits/is_iterator.hpp>#include <hpx/util/invoke.hpp>#include <hpx/parallel/algorithms/detail/dispatch.hpp>#include <hpx/parallel/algorithms/detail/predicates.hpp>#include <hpx/parallel/algorithms/for_each.hpp>#include <hpx/parallel/algorithms/mismatch.hpp>#include <hpx/parallel/execution_policy.hpp>#include <hpx/parallel/util/detail/algorithm_result.hpp>#include <hpx/parallel/util/loop.hpp>#include <hpx/parallel/util/partitioner.hpp>#include <hpx/parallel/util/zip_iterator.hpp>#include <algorithm>#include <cstddef>#include <iterator>#include <type_traits>#include <utility>#include <vector>
-
file
merge.hpp
- #include <hpx/config.hpp>#include <hpx/traits/concepts.hpp>#include <hpx/traits/is_iterator.hpp>#include <hpx/util/assert.hpp>#include <hpx/util/invoke.hpp>#include <hpx/util/tagged_tuple.hpp>#include <hpx/parallel/algorithms/detail/dispatch.hpp>#include <hpx/parallel/algorithms/detail/is_negative.hpp>#include <hpx/parallel/algorithms/detail/predicates.hpp>#include <hpx/parallel/algorithms/detail/transfer.hpp>#include <hpx/parallel/execution_policy.hpp>#include <hpx/parallel/tagspec.hpp>#include <hpx/parallel/traits/projected.hpp>#include <hpx/parallel/util/compare_projected.hpp>#include <hpx/parallel/util/detail/algorithm_result.hpp>#include <hpx/parallel/util/detail/handle_local_exceptions.hpp>#include <hpx/parallel/util/loop.hpp>#include <hpx/parallel/util/projection_identity.hpp>#include <hpx/parallel/util/transfer.hpp>#include <algorithm>#include <cstddef>#include <exception>#include <iterator>#include <list>#include <memory>#include <type_traits>#include <utility>#include <vector>
-
file
merge.hpp
- #include <hpx/config.hpp>#include <hpx/traits/concepts.hpp>#include <hpx/traits/is_iterator.hpp>#include <hpx/traits/is_range.hpp>#include <hpx/util/range.hpp>#include <hpx/util/tagged_tuple.hpp>#include <hpx/parallel/algorithms/merge.hpp>#include <hpx/parallel/tagspec.hpp>#include <hpx/parallel/traits/projected.hpp>#include <hpx/parallel/traits/projected_range.hpp>#include <type_traits>#include <utility>
-
file
minmax.hpp
- #include <hpx/config.hpp>#include <hpx/traits/concepts.hpp>#include <hpx/traits/is_iterator.hpp>#include <hpx/traits/segmented_iterator_traits.hpp>#include <hpx/util/assert.hpp>#include <hpx/util/invoke.hpp>#include <hpx/util/tagged_pair.hpp>#include <hpx/parallel/algorithms/detail/dispatch.hpp>#include <hpx/parallel/execution_policy.hpp>#include <hpx/parallel/tagspec.hpp>#include <hpx/parallel/traits/projected.hpp>#include <hpx/parallel/util/compare_projected.hpp>#include <hpx/parallel/util/detail/algorithm_result.hpp>#include <hpx/parallel/util/loop.hpp>#include <hpx/parallel/util/partitioner.hpp>#include <hpx/parallel/util/projection_identity.hpp>#include <algorithm>#include <cstddef>#include <iterator>#include <type_traits>#include <utility>#include <vector>
-
file
minmax.hpp
- #include <hpx/config.hpp>#include <hpx/traits/concepts.hpp>#include <hpx/traits/is_range.hpp>#include <hpx/util/range.hpp>#include <hpx/util/tagged_pair.hpp>#include <hpx/parallel/algorithms/minmax.hpp>#include <hpx/parallel/traits/projected.hpp>#include <hpx/parallel/traits/projected_range.hpp>#include <type_traits>#include <utility>
-
file
mismatch.hpp
- #include <hpx/config.hpp>#include <hpx/traits/is_iterator.hpp>#include <hpx/util/invoke.hpp>#include <hpx/parallel/algorithms/detail/dispatch.hpp>#include <hpx/parallel/algorithms/detail/predicates.hpp>#include <hpx/parallel/execution_policy.hpp>#include <hpx/parallel/util/detail/algorithm_result.hpp>#include <hpx/parallel/util/loop.hpp>#include <hpx/parallel/util/partitioner.hpp>#include <hpx/parallel/util/zip_iterator.hpp>#include <algorithm>#include <cstddef>#include <iterator>#include <type_traits>#include <utility>#include <vector>
-
file
move.hpp
- #include <hpx/config.hpp>#include <hpx/traits/is_iterator.hpp>#include <hpx/parallel/algorithms/detail/dispatch.hpp>#include <hpx/parallel/algorithms/detail/transfer.hpp>#include <hpx/parallel/execution_policy.hpp>#include <hpx/parallel/util/detail/algorithm_result.hpp>#include <hpx/parallel/util/foreach_partitioner.hpp>#include <hpx/parallel/util/transfer.hpp>#include <hpx/parallel/util/zip_iterator.hpp>#include <hpx/traits/segmented_iterator_traits.hpp>#include <algorithm>#include <cstddef>#include <iterator>#include <type_traits>#include <utility>
-
file
partition.hpp
- #include <hpx/config.hpp>#include <hpx/async.hpp>#include <hpx/lcos/dataflow.hpp>#include <hpx/lcos/future.hpp>#include <hpx/lcos/local/spinlock.hpp>#include <hpx/traits/concepts.hpp>#include <hpx/traits/is_callable.hpp>#include <hpx/traits/is_iterator.hpp>#include <hpx/util/assert.hpp>#include <hpx/util/invoke.hpp>#include <hpx/util/tagged_tuple.hpp>#include <hpx/util/unused.hpp>#include <hpx/parallel/algorithms/detail/dispatch.hpp>#include <hpx/parallel/exception_list.hpp>#include <hpx/parallel/execution_policy.hpp>#include <hpx/parallel/executors/execution.hpp>#include <hpx/parallel/executors/execution_information.hpp>#include <hpx/parallel/executors/execution_parameters.hpp>#include <hpx/parallel/tagspec.hpp>#include <hpx/parallel/traits/projected.hpp>#include <hpx/parallel/util/detail/algorithm_result.hpp>#include <hpx/parallel/util/detail/handle_local_exceptions.hpp>#include <hpx/parallel/util/invoke_projected.hpp>#include <hpx/parallel/util/loop.hpp>#include <hpx/parallel/util/projection_identity.hpp>#include <hpx/parallel/util/scan_partitioner.hpp>#include <hpx/parallel/util/zip_iterator.hpp>#include <algorithm>#include <cstddef>#include <cstdint>#include <exception>#include <iterator>#include <list>#include <type_traits>#include <utility>#include <vector>#include <boost/shared_array.hpp>
-
file
partition.hpp
- #include <hpx/config.hpp>#include <hpx/traits/concepts.hpp>#include <hpx/traits/is_iterator.hpp>#include <hpx/traits/is_range.hpp>#include <hpx/util/range.hpp>#include <hpx/util/tagged_tuple.hpp>#include <hpx/parallel/algorithms/partition.hpp>#include <hpx/parallel/tagspec.hpp>#include <hpx/parallel/traits/projected.hpp>#include <hpx/parallel/traits/projected_range.hpp>#include <type_traits>#include <utility>
-
file
reduce.hpp
- #include <hpx/config.hpp>#include <hpx/traits/is_iterator.hpp>#include <hpx/util/range.hpp>#include <hpx/util/unwrap.hpp>#include <hpx/parallel/algorithms/detail/accumulate.hpp>#include <functional>#include <hpx/parallel/algorithms/detail/dispatch.hpp>#include <hpx/parallel/algorithms/detail/distance.hpp>#include <hpx/parallel/execution_policy.hpp>#include <hpx/parallel/util/detail/algorithm_result.hpp>#include <hpx/parallel/util/loop.hpp>#include <hpx/parallel/util/partitioner.hpp>#include <algorithm>#include <cstddef>#include <iterator>#include <numeric>#include <type_traits>#include <utility>#include <vector>
-
file
reduce.hpp
-
file
reduce_by_key.hpp
- #include <hpx/config.hpp>#include <hpx/parallel/executors/execution.hpp>#include <hpx/parallel/algorithms/copy.hpp>#include <hpx/parallel/algorithms/for_each.hpp>#include <hpx/parallel/algorithms/inclusive_scan.hpp>#include <hpx/parallel/algorithms/sort.hpp>#include <hpx/parallel/util/zip_iterator.hpp>#include <hpx/util/range.hpp>#include <hpx/util/transform_iterator.hpp>#include <hpx/util/identity.hpp>#include <hpx/util/iterator_adaptor.hpp>#include <hpx/util/lazy_conditional.hpp>#include <hpx/util/result_of.hpp>#include <iterator>#include <type_traits>#include <hpx/util/tuple.hpp>#include <cstdint>#include <functional>#include <utility>#include <vector>
-
file
remove.hpp
- #include <hpx/config.hpp>#include <hpx/traits/concepts.hpp>#include <hpx/traits/is_iterator.hpp>#include <hpx/util/invoke.hpp>#include <hpx/util/tagged_pair.hpp>#include <hpx/util/unused.hpp>#include <hpx/parallel/algorithms/detail/dispatch.hpp>#include <hpx/parallel/algorithms/detail/is_negative.hpp>#include <hpx/parallel/algorithms/detail/predicates.hpp>#include <hpx/parallel/algorithms/detail/transfer.hpp>#include <hpx/parallel/execution_policy.hpp>#include <hpx/parallel/tagspec.hpp>#include <hpx/parallel/traits/projected.hpp>#include <hpx/parallel/util/detail/algorithm_result.hpp>#include <hpx/parallel/util/foreach_partitioner.hpp>#include <hpx/parallel/util/invoke_projected.hpp>#include <hpx/parallel/util/loop.hpp>#include <hpx/parallel/util/projection_identity.hpp>#include <hpx/parallel/util/scan_partitioner.hpp>#include <hpx/parallel/util/transfer.hpp>#include <hpx/parallel/util/zip_iterator.hpp>#include <algorithm>#include <cstddef>#include <cstring>#include <iterator>#include <memory>#include <type_traits>#include <utility>#include <vector>#include <boost/shared_array.hpp>
-
file
remove.hpp
- #include <hpx/config.hpp>#include <hpx/traits/concepts.hpp>#include <hpx/traits/is_iterator.hpp>#include <hpx/traits/is_range.hpp>#include <hpx/util/range.hpp>#include <hpx/util/tagged_pair.hpp>#include <hpx/parallel/algorithms/remove.hpp>#include <hpx/parallel/tagspec.hpp>#include <hpx/parallel/traits/projected.hpp>#include <hpx/parallel/traits/projected_range.hpp>#include <type_traits>#include <utility>
-
file
remove_copy.hpp
- #include <hpx/config.hpp>#include <hpx/traits/concepts.hpp>#include <hpx/traits/is_iterator.hpp>#include <hpx/util/invoke.hpp>#include <hpx/util/tagged_pair.hpp>#include <hpx/parallel/algorithms/copy.hpp>#include <hpx/parallel/algorithms/detail/dispatch.hpp>#include <hpx/parallel/execution_policy.hpp>#include <hpx/parallel/tagspec.hpp>#include <hpx/parallel/util/detail/algorithm_result.hpp>#include <hpx/parallel/util/projection_identity.hpp>#include <hpx/util/unused.hpp>#include <algorithm>#include <iterator>#include <type_traits>#include <utility>
-
file
remove_copy.hpp
- #include <hpx/config.hpp>#include <hpx/traits/concepts.hpp>#include <hpx/traits/is_iterator.hpp>#include <hpx/traits/is_range.hpp>#include <hpx/util/range.hpp>#include <hpx/util/tagged_pair.hpp>#include <hpx/parallel/algorithms/remove_copy.hpp>#include <hpx/parallel/tagspec.hpp>#include <hpx/parallel/traits/projected.hpp>#include <hpx/parallel/traits/projected_range.hpp>#include <hpx/parallel/util/projection_identity.hpp>#include <type_traits>#include <utility>
-
file
replace.hpp
- #include <hpx/config.hpp>#include <hpx/traits/concepts.hpp>#include <hpx/traits/is_iterator.hpp>#include <hpx/util/invoke.hpp>#include <hpx/util/tagged_pair.hpp>#include <hpx/util/unused.hpp>#include <hpx/parallel/algorithms/detail/dispatch.hpp>#include <hpx/parallel/algorithms/for_each.hpp>#include <hpx/parallel/execution_policy.hpp>#include <hpx/parallel/tagspec.hpp>#include <hpx/parallel/traits/projected.hpp>#include <hpx/parallel/util/detail/algorithm_result.hpp>#include <hpx/parallel/util/projection_identity.hpp>#include <hpx/parallel/util/zip_iterator.hpp>#include <algorithm>#include <iterator>#include <type_traits>#include <utility>
-
file
replace.hpp
- #include <hpx/config.hpp>#include <hpx/traits/concepts.hpp>#include <hpx/traits/is_range.hpp>#include <hpx/util/range.hpp>#include <hpx/util/tagged_pair.hpp>#include <hpx/parallel/algorithms/replace.hpp>#include <hpx/parallel/tagspec.hpp>#include <hpx/parallel/traits/projected_range.hpp>#include <hpx/parallel/util/projection_identity.hpp>#include <type_traits>#include <utility>
-
file
reverse.hpp
- #include <hpx/config.hpp>#include <hpx/traits/concepts.hpp>#include <hpx/traits/is_iterator.hpp>#include <hpx/util/tagged_pair.hpp>#include <hpx/parallel/algorithms/copy.hpp>#include <hpx/parallel/algorithms/detail/dispatch.hpp>#include <hpx/parallel/algorithms/for_each.hpp>#include <hpx/parallel/execution_policy.hpp>#include <hpx/parallel/tagspec.hpp>#include <hpx/parallel/util/detail/algorithm_result.hpp>#include <hpx/parallel/util/projection_identity.hpp>#include <hpx/parallel/util/zip_iterator.hpp>#include <algorithm>#include <iterator>#include <type_traits>#include <utility>
-
file
reverse.hpp
- #include <hpx/config.hpp>#include <hpx/traits/concepts.hpp>#include <hpx/traits/is_iterator.hpp>#include <hpx/traits/is_range.hpp>#include <hpx/util/range.hpp>#include <hpx/util/tagged_pair.hpp>#include <hpx/parallel/algorithms/reverse.hpp>#include <hpx/parallel/tagspec.hpp>#include <hpx/parallel/traits/projected_range.hpp>#include <hpx/parallel/util/projection_identity.hpp>#include <type_traits>#include <utility>
-
file
rotate.hpp
- #include <hpx/config.hpp>#include <hpx/dataflow.hpp>#include <hpx/traits/concepts.hpp>#include <hpx/traits/is_iterator.hpp>#include <hpx/util/tagged_pair.hpp>#include <hpx/util/unwrap.hpp>#include <hpx/parallel/algorithms/copy.hpp>#include <hpx/parallel/algorithms/detail/dispatch.hpp>#include <hpx/parallel/algorithms/reverse.hpp>#include <hpx/parallel/execution_policy.hpp>#include <hpx/parallel/tagspec.hpp>#include <hpx/parallel/util/detail/algorithm_result.hpp>#include <hpx/parallel/util/transfer.hpp>#include <algorithm>#include <iterator>#include <type_traits>#include <utility>
-
file
rotate.hpp
- #include <hpx/config.hpp>#include <hpx/traits/concepts.hpp>#include <hpx/traits/is_iterator.hpp>#include <hpx/traits/is_range.hpp>#include <hpx/util/range.hpp>#include <hpx/util/tagged_pair.hpp>#include <hpx/parallel/algorithms/rotate.hpp>#include <hpx/parallel/tagspec.hpp>#include <hpx/parallel/traits/projected_range.hpp>#include <hpx/parallel/util/projection_identity.hpp>#include <type_traits>#include <utility>
-
file
search.hpp
- #include <hpx/config.hpp>#include <hpx/traits/is_iterator.hpp>#include <hpx/parallel/algorithms/detail/dispatch.hpp>#include <hpx/parallel/algorithms/detail/predicates.hpp>#include <hpx/parallel/algorithms/for_each.hpp>#include <hpx/parallel/execution_policy.hpp>#include <hpx/parallel/util/detail/algorithm_result.hpp>#include <hpx/parallel/util/compare_projected.hpp>#include <hpx/parallel/util/zip_iterator.hpp>#include <hpx/parallel/util/loop.hpp>#include <hpx/parallel/util/partitioner.hpp>#include <algorithm>#include <cstddef>#include <iterator>#include <type_traits>#include <utility>#include <vector>
-
file
search.hpp
- #include <hpx/config.hpp>#include <hpx/traits/is_execution_policy.hpp>#include <hpx/traits/is_range.hpp>#include <hpx/util/range.hpp>#include <hpx/parallel/algorithms/search.hpp>#include <hpx/parallel/traits/projected.hpp>#include <hpx/parallel/traits/projected_range.hpp>#include <cstddef>#include <type_traits>#include <utility>
-
file
set_difference.hpp
- #include <hpx/config.hpp>#include <hpx/traits/is_iterator.hpp>#include <hpx/util/decay.hpp>#include <hpx/parallel/algorithms/copy.hpp>#include <hpx/parallel/algorithms/detail/dispatch.hpp>#include <hpx/parallel/algorithms/detail/set_operation.hpp>#include <hpx/parallel/execution_policy.hpp>#include <hpx/parallel/util/detail/algorithm_result.hpp>#include <hpx/parallel/util/loop.hpp>#include <algorithm>#include <iterator>#include <type_traits>#include <utility>
-
file
set_intersection.hpp
- #include <hpx/config.hpp>#include <hpx/traits/is_iterator.hpp>#include <hpx/util/decay.hpp>#include <hpx/parallel/algorithms/copy.hpp>#include <hpx/parallel/algorithms/detail/dispatch.hpp>#include <hpx/parallel/algorithms/detail/set_operation.hpp>#include <hpx/parallel/execution_policy.hpp>#include <hpx/parallel/util/detail/algorithm_result.hpp>#include <hpx/parallel/util/loop.hpp>#include <algorithm>#include <iterator>#include <type_traits>#include <utility>
-
file
set_symmetric_difference.hpp
- #include <hpx/config.hpp>#include <hpx/traits/is_iterator.hpp>#include <hpx/util/decay.hpp>#include <hpx/parallel/algorithms/copy.hpp>#include <hpx/parallel/algorithms/detail/dispatch.hpp>#include <hpx/parallel/algorithms/detail/set_operation.hpp>#include <hpx/parallel/execution_policy.hpp>#include <hpx/parallel/util/detail/algorithm_result.hpp>#include <hpx/parallel/util/loop.hpp>#include <algorithm>#include <iterator>#include <type_traits>#include <utility>
-
file
set_union.hpp
- #include <hpx/config.hpp>#include <hpx/traits/is_iterator.hpp>#include <hpx/util/decay.hpp>#include <hpx/parallel/algorithms/copy.hpp>#include <hpx/parallel/algorithms/detail/dispatch.hpp>#include <hpx/parallel/algorithms/detail/set_operation.hpp>#include <hpx/parallel/execution_policy.hpp>#include <hpx/parallel/util/detail/algorithm_result.hpp>#include <hpx/parallel/util/loop.hpp>#include <algorithm>#include <iterator>#include <type_traits>#include <utility>
-
file
sort.hpp
- #include <hpx/config.hpp>#include <hpx/dataflow.hpp>#include <hpx/traits/concepts.hpp>#include <hpx/traits/is_iterator.hpp>#include <hpx/util/assert.hpp>#include <hpx/util/decay.hpp>#include <hpx/util/invoke.hpp>#include <hpx/parallel/algorithms/detail/dispatch.hpp>#include <hpx/parallel/algorithms/detail/predicates.hpp>#include <hpx/parallel/exception_list.hpp>#include <hpx/parallel/execution_policy.hpp>#include <hpx/parallel/executors/execution.hpp>#include <hpx/parallel/executors/execution_information.hpp>#include <hpx/parallel/executors/execution_parameters.hpp>#include <hpx/parallel/traits/projected.hpp>#include <hpx/parallel/util/compare_projected.hpp>#include <hpx/parallel/util/detail/algorithm_result.hpp>#include <hpx/parallel/util/projection_identity.hpp>#include <algorithm>#include <cstddef>#include <exception>#include <functional>#include <iterator>#include <list>#include <type_traits>#include <utility>
-
file
sort.hpp
- #include <hpx/config.hpp>#include <hpx/traits/concepts.hpp>#include <hpx/traits/is_range.hpp>#include <hpx/util/range.hpp>#include <hpx/parallel/algorithms/sort.hpp>#include <hpx/parallel/traits/projected_range.hpp>#include <hpx/parallel/util/projection_identity.hpp>#include <type_traits>#include <utility>
-
file
sort_by_key.hpp
- #include <hpx/config.hpp>#include <hpx/util/tagged_pair.hpp>#include <hpx/util/tuple.hpp>#include <hpx/parallel/algorithms/sort.hpp>#include <hpx/parallel/tagspec.hpp>#include <hpx/parallel/util/zip_iterator.hpp>#include <algorithm>#include <iterator>#include <type_traits>#include <utility>
-
file
swap_ranges.hpp
- #include <hpx/config.hpp>#include <hpx/traits/is_iterator.hpp>#include <hpx/parallel/algorithms/detail/dispatch.hpp>#include <hpx/parallel/algorithms/for_each.hpp>#include <hpx/parallel/execution_policy.hpp>#include <hpx/parallel/util/detail/algorithm_result.hpp>#include <hpx/parallel/util/projection_identity.hpp>#include <hpx/parallel/util/zip_iterator.hpp>#include <algorithm>#include <iterator>#include <type_traits>#include <utility>
-
file
transform.hpp
- #include <hpx/config.hpp>#include <hpx/traits/concepts.hpp>#include <hpx/traits/is_callable.hpp>#include <hpx/traits/is_iterator.hpp>#include <hpx/traits/segmented_iterator_traits.hpp>#include <hpx/util/annotated_function.hpp>#include <hpx/util/invoke.hpp>#include <hpx/util/tagged_pair.hpp>#include <hpx/util/tagged_tuple.hpp>#include <hpx/util/tuple.hpp>#include <hpx/parallel/algorithms/detail/dispatch.hpp>#include <hpx/parallel/execution_policy.hpp>#include <hpx/parallel/tagspec.hpp>#include <hpx/parallel/traits/projected.hpp>#include <hpx/parallel/util/detail/algorithm_result.hpp>#include <hpx/parallel/util/foreach_partitioner.hpp>#include <hpx/parallel/util/projection_identity.hpp>#include <hpx/parallel/util/transform_loop.hpp>#include <hpx/parallel/util/cancellation_token.hpp>#include <hpx/traits/is_execution_policy.hpp>#include <hpx/util/invoke.hpp>#include <algorithm>#include <cstddef>#include <iterator>#include <type_traits>#include <utility>#include <hpx/parallel/util/zip_iterator.hpp>#include <cstdint>
-
file
transform.hpp
- #include <hpx/config.hpp>#include <hpx/traits/concepts.hpp>#include <hpx/traits/is_iterator.hpp>#include <hpx/traits/is_range.hpp>#include <hpx/util/range.hpp>#include <hpx/util/tagged_pair.hpp>#include <hpx/util/tagged_tuple.hpp>#include <hpx/parallel/algorithms/transform.hpp>#include <hpx/traits/is_callable.hpp>#include <hpx/traits/segmented_iterator_traits.hpp>#include <hpx/util/annotated_function.hpp>#include <hpx/util/invoke.hpp>#include <hpx/util/tuple.hpp>#include <hpx/parallel/algorithms/detail/dispatch.hpp>#include <hpx/parallel/execution_policy.hpp>#include <hpx/parallel/tagspec.hpp>#include <hpx/parallel/traits/projected.hpp>#include <hpx/parallel/util/detail/algorithm_result.hpp>#include <hpx/parallel/util/foreach_partitioner.hpp>#include <hpx/parallel/util/projection_identity.hpp>#include <hpx/parallel/util/transform_loop.hpp>#include <hpx/parallel/util/zip_iterator.hpp>#include <algorithm>#include <cstddef>#include <cstdint>#include <iterator>#include <type_traits>#include <utility>#include <hpx/parallel/traits/projected_range.hpp>
-
file
transform_exclusive_scan.hpp
- #include <hpx/config.hpp>#include <hpx/traits/concepts.hpp>#include <hpx/traits/is_callable.hpp>#include <hpx/traits/is_iterator.hpp>#include <hpx/util/invoke.hpp>#include <hpx/util/result_of.hpp>#include <hpx/util/unused.hpp>#include <hpx/parallel/algorithms/detail/dispatch.hpp>#include <hpx/parallel/algorithms/transform_inclusive_scan.hpp>#include <hpx/util/invoke.hpp>#include <hpx/parallel/algorithms/inclusive_scan.hpp>#include <hpx/parallel/execution_policy.hpp>#include <hpx/parallel/util/detail/algorithm_result.hpp>#include <hpx/parallel/util/loop.hpp>#include <hpx/parallel/util/partitioner.hpp>#include <hpx/parallel/util/scan_partitioner.hpp>#include <algorithm>#include <cstddef>#include <iterator>#include <numeric>#include <type_traits>#include <utility>#include <vector>#include <hpx/parallel/execution_policy.hpp>
-
file
transform_inclusive_scan.hpp
- #include <hpx/config.hpp>#include <hpx/traits/concepts.hpp>#include <hpx/traits/is_callable.hpp>#include <hpx/traits/is_iterator.hpp>#include <hpx/util/invoke.hpp>#include <hpx/util/result_of.hpp>#include <hpx/parallel/algorithms/detail/dispatch.hpp>#include <hpx/parallel/algorithms/inclusive_scan.hpp>#include <hpx/parallel/execution_policy.hpp>#include <hpx/parallel/util/detail/algorithm_result.hpp>#include <hpx/parallel/util/loop.hpp>#include <hpx/parallel/util/partitioner.hpp>#include <hpx/parallel/util/scan_partitioner.hpp>#include <hpx/util/unused.hpp>#include <algorithm>#include <cstddef>#include <iterator>#include <numeric>#include <type_traits>#include <utility>#include <vector>
-
file
transform_reduce.hpp
- #include <hpx/config.hpp>#include <hpx/traits/concepts.hpp>#include <hpx/traits/is_callable.hpp>#include <hpx/traits/is_iterator.hpp>#include <hpx/traits/segmented_iterator_traits.hpp>#include <hpx/util/range.hpp>#include <hpx/util/result_of.hpp>#include <hpx/util/unwrap.hpp>#include <hpx/parallel/algorithms/detail/dispatch.hpp>#include <hpx/parallel/algorithms/detail/predicates.hpp>#include <hpx/parallel/execution_policy.hpp>#include <hpx/parallel/util/detail/algorithm_result.hpp>#include <hpx/parallel/util/loop.hpp>#include <hpx/parallel/util/partitioner.hpp>#include <algorithm>#include <cstddef>#include <iterator>#include <numeric>#include <type_traits>#include <utility>#include <vector>
-
file
transform_reduce_binary.hpp
- #include <hpx/config.hpp>#include <hpx/traits/concepts.hpp>#include <hpx/traits/is_callable.hpp>#include <hpx/traits/is_iterator.hpp>#include <hpx/util/invoke.hpp>#include <hpx/util/result_of.hpp>#include <hpx/util/zip_iterator.hpp>#include <hpx/parallel/algorithms/detail/dispatch.hpp>#include <hpx/parallel/execution_policy.hpp>#include <hpx/parallel/util/detail/algorithm_result.hpp>#include <hpx/parallel/util/loop.hpp>#include <hpx/parallel/util/partitioner.hpp>#include <hpx/util/unused.hpp>#include <algorithm>#include <cstddef>#include <iterator>#include <numeric>#include <type_traits>#include <utility>#include <vector>
-
file
uninitialized_copy.hpp
- #include <hpx/config.hpp>#include <hpx/traits/is_iterator.hpp>#include <hpx/parallel/algorithms/detail/dispatch.hpp>#include <hpx/parallel/algorithms/detail/is_negative.hpp>#include <hpx/parallel/execution_policy.hpp>#include <hpx/parallel/util/detail/algorithm_result.hpp>#include <hpx/parallel/util/loop.hpp>#include <hpx/parallel/util/partitioner_with_cleanup.hpp>#include <hpx/dataflow.hpp>#include <hpx/exception_list.hpp>#include <hpx/lcos/wait_all.hpp>#include <hpx/util/unused.hpp>#include <hpx/parallel/execution_policy.hpp>#include <hpx/parallel/executors/execution.hpp>#include <hpx/parallel/executors/execution_parameters.hpp>#include <hpx/parallel/util/detail/chunk_size.hpp>#include <hpx/parallel/util/detail/handle_local_exceptions.hpp>#include <hpx/parallel/util/detail/partitioner_iteration.hpp>#include <hpx/parallel/util/detail/scoped_executor_parameters.hpp>#include <hpx/parallel/util/detail/select_partitioner.hpp>#include <hpx/parallel/util/partitioner.hpp>#include <algorithm>#include <cstddef>#include <exception>#include <list>#include <memory>#include <type_traits>#include <utility>#include <vector>#include <hpx/parallel/util/zip_iterator.hpp>#include <iterator>
-
file
uninitialized_default_construct.hpp
- #include <hpx/config.hpp>#include <hpx/traits/is_iterator.hpp>#include <hpx/util/void_guard.hpp>#include <hpx/parallel/algorithms/detail/dispatch.hpp>#include <hpx/parallel/algorithms/detail/is_negative.hpp>#include <hpx/parallel/execution_policy.hpp>#include <hpx/parallel/util/detail/algorithm_result.hpp>#include <hpx/parallel/util/loop.hpp>#include <hpx/parallel/util/partitioner_with_cleanup.hpp>#include <hpx/parallel/util/zip_iterator.hpp>#include <algorithm>#include <cstddef>#include <iterator>#include <memory>#include <type_traits>#include <utility>#include <vector>
-
file
uninitialized_fill.hpp
- #include <hpx/config.hpp>#include <hpx/traits/is_iterator.hpp>#include <hpx/parallel/algorithms/detail/dispatch.hpp>#include <hpx/parallel/algorithms/detail/is_negative.hpp>#include <hpx/parallel/execution_policy.hpp>#include <hpx/parallel/util/detail/algorithm_result.hpp>#include <hpx/parallel/util/loop.hpp>#include <hpx/parallel/util/partitioner_with_cleanup.hpp>#include <hpx/parallel/util/zip_iterator.hpp>#include <algorithm>#include <cstddef>#include <iterator>#include <memory>#include <type_traits>#include <utility>#include <vector>
-
file
uninitialized_move.hpp
- #include <hpx/config.hpp>#include <hpx/traits/concepts.hpp>#include <hpx/traits/is_iterator.hpp>#include <hpx/util/tagged_pair.hpp>#include <hpx/parallel/algorithms/detail/dispatch.hpp>#include <hpx/parallel/algorithms/detail/is_negative.hpp>#include <hpx/parallel/execution_policy.hpp>#include <hpx/parallel/tagspec.hpp>#include <hpx/parallel/util/detail/algorithm_result.hpp>#include <hpx/parallel/util/loop.hpp>#include <hpx/parallel/util/partitioner_with_cleanup.hpp>#include <hpx/parallel/util/zip_iterator.hpp>#include <algorithm>#include <cstddef>#include <iterator>#include <memory>#include <type_traits>#include <utility>#include <vector>
-
file
uninitialized_value_construct.hpp
- #include <hpx/config.hpp>#include <hpx/traits/is_iterator.hpp>#include <hpx/util/void_guard.hpp>#include <hpx/parallel/algorithms/detail/dispatch.hpp>#include <hpx/parallel/algorithms/detail/is_negative.hpp>#include <hpx/parallel/execution_policy.hpp>#include <hpx/parallel/util/detail/algorithm_result.hpp>#include <hpx/parallel/util/loop.hpp>#include <hpx/parallel/util/partitioner_with_cleanup.hpp>#include <hpx/parallel/util/zip_iterator.hpp>#include <algorithm>#include <cstddef>#include <iterator>#include <memory>#include <type_traits>#include <utility>#include <vector>
-
file
unique.hpp
- #include <hpx/config.hpp>#include <hpx/traits/concepts.hpp>#include <hpx/traits/is_iterator.hpp>#include <hpx/util/invoke.hpp>#include <hpx/util/tagged_pair.hpp>#include <hpx/util/unused.hpp>#include <hpx/parallel/algorithms/detail/dispatch.hpp>#include <hpx/parallel/algorithms/detail/is_negative.hpp>#include <hpx/parallel/algorithms/detail/predicates.hpp>#include <hpx/parallel/algorithms/detail/transfer.hpp>#include <hpx/parallel/execution_policy.hpp>#include <hpx/parallel/tagspec.hpp>#include <hpx/parallel/traits/projected.hpp>#include <hpx/parallel/util/compare_projected.hpp>#include <hpx/parallel/util/detail/algorithm_result.hpp>#include <hpx/parallel/util/foreach_partitioner.hpp>#include <hpx/parallel/util/loop.hpp>#include <hpx/parallel/util/projection_identity.hpp>#include <hpx/parallel/util/scan_partitioner.hpp>#include <hpx/parallel/util/transfer.hpp>#include <hpx/parallel/util/zip_iterator.hpp>#include <algorithm>#include <cstddef>#include <cstring>#include <iterator>#include <memory>#include <type_traits>#include <utility>#include <vector>#include <boost/shared_array.hpp>
-
file
unique.hpp
- #include <hpx/config.hpp>#include <hpx/traits/concepts.hpp>#include <hpx/traits/is_iterator.hpp>#include <hpx/traits/is_range.hpp>#include <hpx/util/range.hpp>#include <hpx/util/tagged_pair.hpp>#include <hpx/parallel/algorithms/unique.hpp>#include <hpx/parallel/tagspec.hpp>#include <hpx/parallel/traits/projected.hpp>#include <hpx/parallel/traits/projected_range.hpp>#include <type_traits>#include <utility>
-
file
execution_policy.hpp
- #include <hpx/config.hpp>#include <hpx/parallel/datapar/execution_policy.hpp>#include <hpx/parallel/execution_policy_fwd.hpp>#include <hpx/parallel/executors/execution.hpp>#include <hpx/parallel/executors/execution_parameters.hpp>#include <hpx/lcos/future.hpp>#include <hpx/preprocessor/cat.hpp>#include <hpx/preprocessor/stringize.hpp>#include <hpx/runtime/serialization/base_object.hpp>#include <hpx/traits/detail/wrap_int.hpp>#include <hpx/traits/has_member_xxx.hpp>#include <hpx/traits/is_executor.hpp>#include <hpx/traits/is_executor_parameters.hpp>#include <hpx/traits/is_launch_policy.hpp>#include <hpx/util/decay.hpp>#include <hpx/util/detail/pack.hpp>#include <hpx/parallel/executors/execution_parameters_fwd.hpp>#include <boost/ref.hpp>#include <cstddef>#include <functional>#include <type_traits>#include <utility>#include <vector>#include <hpx/parallel/executors/parallel_executor.hpp>#include <hpx/parallel/executors/rebind_executor.hpp>#include <hpx/parallel/executors/execution_fwd.hpp>#include <hpx/traits/executor_traits.hpp>#include <hpx/parallel/executors/sequenced_executor.hpp>#include <hpx/async_launch_policy_dispatch.hpp>#include <hpx/runtime/threads/thread_executor.hpp>#include <hpx/sync_launch_policy_dispatch.hpp>#include <hpx/lcos/sync_fwd.hpp>#include <hpx/lcos/local/futures_factory.hpp>#include <hpx/runtime/launch_policy.hpp>#include <hpx/traits/is_action.hpp>#include <hpx/util/invoke.hpp>#include <hpx/util/deferred_call.hpp>#include <hpx/util/unwrap.hpp>#include <hpx/parallel/exception_list.hpp>#include <hpx/exception_list.hpp>#include <hpx/hpx_finalize.hpp>#include <hpx/util/assert.hpp>#include <exception>#include <iterator>#include <hpx/runtime/serialization/serialize.hpp>#include <hpx/traits/is_execution_policy.hpp>#include <memory>
-
file
auto_chunk_size.hpp
- #include <hpx/config.hpp>#include <hpx/runtime/serialization/serialize.hpp>#include <hpx/traits/is_executor_parameters.hpp>#include <hpx/util/high_resolution_clock.hpp>#include <hpx/util/steady_clock.hpp>#include <hpx/parallel/executors/execution_parameters.hpp>#include <algorithm>#include <cstddef>#include <cstdint>#include <type_traits>
-
file
dynamic_chunk_size.hpp
- #include <hpx/config.hpp>#include <hpx/runtime/serialization/serialize.hpp>#include <hpx/traits/is_executor_parameters.hpp>#include <cstddef>#include <type_traits>
-
file
execution_fwd.hpp
- #include <utility>#include <type_traits>#include <hpx/config.hpp>#include <hpx/traits/executor_traits.hpp>
-
file
execution_information_fwd.hpp
- #include <hpx/config.hpp>#include <hpx/parallel/executors/execution_fwd.hpp>#include <hpx/runtime/threads/thread_data_fwd.hpp>#include <hpx/traits/executor_traits.hpp>#include <cstddef>#include <type_traits>#include <utility>
-
file
guided_chunk_size.hpp
- #include <hpx/config.hpp>#include <hpx/runtime/serialization/serialize.hpp>#include <hpx/traits/is_executor_parameters.hpp>#include <algorithm>#include <cstddef>#include <type_traits>
-
file
parallel_executor.hpp
- #include <hpx/config.hpp>#include <hpx/async_launch_policy_dispatch.hpp>#include <hpx/lcos/future.hpp>#include <hpx/lcos/local/latch.hpp>#include <hpx/parallel/algorithms/detail/predicates.hpp>#include <hpx/parallel/executors/fused_bulk_execute.hpp>#include <hpx/parallel/executors/post_policy_dispatch.hpp>#include <hpx/parallel/executors/static_chunk_size.hpp>#include <hpx/runtime/get_worker_thread_num.hpp>#include <hpx/runtime/launch_policy.hpp>#include <hpx/runtime/serialization/serialize.hpp>#include <hpx/runtime/threads/thread_helpers.hpp>#include <hpx/traits/future_traits.hpp>#include <hpx/traits/is_executor.hpp>#include <hpx/util/assert.hpp>#include <hpx/util/bind_back.hpp>#include <hpx/util/deferred_call.hpp>#include <hpx/util/internal_allocator.hpp>#include <hpx/util/invoke.hpp>#include <hpx/util/one_shot.hpp>#include <hpx/util/range.hpp>#include <hpx/util/unwrap.hpp>#include <algorithm>#include <cstddef>#include <type_traits>#include <utility>#include <vector>
-
file
persistent_auto_chunk_size.hpp
- #include <hpx/config.hpp>#include <hpx/runtime/serialization/serialize.hpp>#include <hpx/traits/is_executor_parameters.hpp>#include <hpx/util/high_resolution_clock.hpp>#include <hpx/util/steady_clock.hpp>#include <algorithm>#include <cstddef>#include <cstdint>#include <type_traits>
-
file
sequenced_executor.hpp
- #include <hpx/config.hpp>#include <hpx/async_launch_policy_dispatch.hpp>#include <hpx/lcos/future.hpp>#include <hpx/runtime/threads/thread_executor.hpp>#include <hpx/sync_launch_policy_dispatch.hpp>#include <hpx/traits/is_executor.hpp>#include <hpx/util/deferred_call.hpp>#include <hpx/util/invoke.hpp>#include <hpx/util/unwrap.hpp>#include <hpx/parallel/exception_list.hpp>#include <cstddef>#include <iterator>#include <type_traits>#include <utility>#include <vector>
-
file
service_executors.hpp
- #include <hpx/config.hpp>#include <hpx/lcos/future.hpp>#include <hpx/parallel/executors/static_chunk_size.hpp>#include <hpx/parallel/executors/thread_execution.hpp>#include <hpx/lcos/dataflow.hpp>#include <hpx/lcos/local/futures_factory.hpp>#include <hpx/runtime/threads/thread_executor.hpp>#include <hpx/traits/future_access.hpp>#include <hpx/traits/is_launch_policy.hpp>#include <hpx/util/bind.hpp>#include <hpx/util/bind_back.hpp>#include <hpx/util/deferred_call.hpp>#include <hpx/util/detail/pack.hpp>#include <hpx/util/range.hpp>#include <hpx/util/tuple.hpp>#include <hpx/util/unwrap.hpp>#include <hpx/parallel/executors/execution.hpp>#include <algorithm>#include <type_traits>#include <utility>#include <vector>#include <hpx/runtime/threads/executors/service_executors.hpp>#include <hpx/compat/condition_variable.hpp>#include <hpx/compat/mutex.hpp>#include <hpx/exception_fwd.hpp>#include <hpx/runtime/threads/thread_enums.hpp>#include <hpx/throw_exception.hpp>#include <hpx/util/atomic_count.hpp>#include <hpx/util/steady_clock.hpp>#include <hpx/util/thread_description.hpp>#include <hpx/util/unique_function.hpp>#include <atomic>#include <chrono>#include <cstddef>#include <cstdint>#include <hpx/config/warnings_prefix.hpp>#include <hpx/config/warnings_suffix.hpp>#include <hpx/traits/executor_traits.hpp>
-
file
static_chunk_size.hpp
- #include <hpx/config.hpp>#include <hpx/runtime/serialization/serialize.hpp>#include <hpx/traits/is_executor_parameters.hpp>#include <hpx/parallel/executors/execution_parameters_fwd.hpp>#include <cstddef>#include <type_traits>
-
file
thread_pool_executors.hpp
- #include <hpx/config.hpp>#include <hpx/lcos/future.hpp>#include <hpx/parallel/executors/execution_parameters.hpp>#include <hpx/parallel/executors/thread_execution.hpp>#include <hpx/parallel/executors/thread_execution_information.hpp>#include <hpx/runtime/get_os_thread_count.hpp>#include <hpx/runtime/threads/policies/scheduler_mode.hpp>#include <hpx/runtime/threads/thread_executor.hpp>#include <hpx/runtime/threads/topology.hpp>#include <hpx/traits/is_launch_policy.hpp>#include <hpx/parallel/executors/execution_information.hpp>#include <cstddef>#include <type_traits>#include <utility>#include <hpx/parallel/executors/thread_timed_execution.hpp>#include <hpx/lcos/local/packaged_task.hpp>#include <hpx/lcos/detail/future_data.hpp>#include <hpx/lcos/local/promise.hpp>#include <hpx/throw_exception.hpp>#include <hpx/traits/is_callable.hpp>#include <hpx/util/annotated_function.hpp>#include <hpx/util/thread_description.hpp>#include <hpx/util/unique_function.hpp>#include <exception>#include <memory>#include <hpx/util/deferred_call.hpp>#include <hpx/util/steady_clock.hpp>#include <hpx/parallel/executors/timed_execution.hpp>#include <hpx/parallel/executors/timed_execution_fwd.hpp>#include <hpx/parallel/executors/execution_fwd.hpp>#include <hpx/parallel/executors/timed_executors.hpp>#include <hpx/runtime/threads/thread.hpp>#include <hpx/traits/detail/wrap_int.hpp>#include <hpx/traits/executor_traits.hpp>#include <hpx/util/bind.hpp>#include <hpx/util/decay.hpp>#include <hpx/parallel/execution_policy.hpp>#include <hpx/parallel/executors/execution.hpp>#include <hpx/parallel/executors/parallel_executor.hpp>#include <hpx/parallel/executors/sequenced_executor.hpp>#include <chrono>#include <functional>#include <hpx/traits/is_executor.hpp>#include <vector>#include <hpx/runtime/threads/executors/thread_pool_executors.hpp>#include <hpx/lcos/local/counting_semaphore.hpp>#include <hpx/lcos/local/detail/counting_semaphore.hpp>#include <hpx/lcos/local/detail/condition_variable.hpp>#include <hpx/lcos/local/spinlock.hpp>#include <hpx/util/assert.hpp>#include <hpx/util/assert_owns_lock.hpp>#include <algorithm>#include <cstdint>#include <mutex>#include <hpx/runtime/resource/detail/partitioner.hpp>#include <hpx/runtime/resource/partitioner.hpp>#include <hpx/runtime/resource/partitioner_fwd.hpp>#include <hpx/runtime/resource/detail/create_partitioner.hpp>#include <hpx/runtime/runtime_mode.hpp>#include <hpx/util/bind_back.hpp>#include <hpx/util/find_prefix.hpp>#include <hpx/preprocessor/stringize.hpp>#include <string>#include <hpx/util/function.hpp>#include <boost/program_options.hpp>#include <hpx/runtime/threads/cpu_mask.hpp>#include <hpx/runtime/threads/policies/affinity_data.hpp>#include <atomic>#include <hpx/config/warnings_prefix.hpp>#include <hpx/config/warnings_suffix.hpp>#include <hpx/util/command_line_handling.hpp>#include <hpx/hpx_init.hpp>#include <hpx/hpx_finalize.hpp>#include <hpx/hpx_suspend.hpp>#include <hpx/runtime/shutdown_function.hpp>#include <hpx/runtime/startup_function.hpp>#include <boost/program_options/options_description.hpp>#include <boost/program_options/variables_map.hpp>#include <hpx/util/manage_config.hpp>#include <hpx/util/safe_lexical_cast.hpp>#include <boost/lexical_cast.hpp>#include <map>#include <hpx/util/runtime_configuration.hpp>#include <hpx/util/tuple.hpp>#include <iosfwd>#include <hpx/runtime/threads/thread_enums.hpp>
-
file
task_block.hpp
- #include <hpx/config.hpp>#include <hpx/async.hpp>#include <hpx/exception.hpp>#include <hpx/lcos/dataflow.hpp>#include <hpx/lcos/future.hpp>#include <hpx/lcos/local/spinlock.hpp>#include <hpx/lcos/when_all.hpp>#include <hpx/traits/is_future.hpp>#include <hpx/util/bind.hpp>#include <hpx/util/bind_back.hpp>#include <hpx/util/decay.hpp>#include <hpx/parallel/exception_list.hpp>#include <hpx/parallel/execution_policy.hpp>#include <hpx/parallel/executors/execution.hpp>#include <hpx/parallel/util/detail/algorithm_result.hpp>#include <boost/utility/addressof.hpp>#include <memory>#include <exception>#include <mutex>#include <type_traits>#include <utility>#include <vector>
-
file
manage_counter_type.hpp
- #include <hpx/config.hpp>#include <hpx/error_code.hpp>#include <hpx/performance_counters/counters_fwd.hpp>#include <hpx/util/function.hpp>#include <cstddef>#include <cstdint>#include <string>#include <vector>
-
file
basic_action.hpp
- #include <hpx/config.hpp>#include <hpx/exception.hpp>#include <hpx/lcos/sync_fwd.hpp>#include <hpx/preprocessor/cat.hpp>#include <hpx/preprocessor/expand.hpp>#include <hpx/preprocessor/nargs.hpp>#include <hpx/preprocessor/stringize.hpp>#include <hpx/runtime/actions/action_support.hpp>#include <hpx/runtime/actions/basic_action_fwd.hpp>#include <hpx/runtime/actions/continuation.hpp>#include <hpx/runtime/actions/detail/action_factory.hpp>#include <hpx/runtime/actions/detail/invocation_count_registry.hpp>#include <hpx/runtime/actions/preassigned_action_id.hpp>#include <hpx/runtime/actions/transfer_action.hpp>#include <hpx/runtime/actions/transfer_continuation_action.hpp>#include <hpx/runtime/launch_policy.hpp>#include <hpx/runtime/naming/address.hpp>#include <hpx/runtime/naming/id_type.hpp>#include <hpx/runtime/parcelset/detail/per_action_data_counter_registry.hpp>#include <hpx/runtime/threads/thread_data_fwd.hpp>#include <hpx/runtime/threads/thread_enums.hpp>#include <hpx/runtime_fwd.hpp>#include <hpx/traits/action_decorate_function.hpp>#include <hpx/traits/action_priority.hpp>#include <hpx/traits/action_remote_result.hpp>#include <hpx/traits/action_stacksize.hpp>#include <hpx/traits/is_action.hpp>#include <hpx/traits/is_distribution_policy.hpp>#include <hpx/traits/promise_local_result.hpp>#include <hpx/util/detail/pack.hpp>#include <hpx/util/get_and_reset_value.hpp>#include <hpx/util/invoke_fused.hpp>#include <hpx/util/logging.hpp>#include <hpx/util/tuple.hpp>#include <boost/utility/string_ref.hpp>#include <atomic>#include <cstddef>#include <cstdint>#include <exception>#include <sstream>#include <string>#include <type_traits>#include <utility>
Defines
-
HPX_REGISTER_ACTION_DECLARATION
(...)¶ Declare the necessary component action boilerplate code.
The macro HPX_REGISTER_ACTION_DECLARATION can be used to declare all the boilerplate code which is required for proper functioning of component actions in the context of HPX.
The parameter action is the type of the action to declare the boilerplate for.
This macro can be invoked with an optional second parameter. This parameter specifies a unique name of the action to be used for serialization purposes. The second parameter has to be specified if the first parameter is not usable as a plain (non-qualified) C++ identifier, i.e. the first parameter contains special characters which cannot be part of a C++ identifier, such as ‘<’, ‘>’, or ‘:’.
namespace app { // Define a simple component exposing one action 'print_greeting' class HPX_COMPONENT_EXPORT server : public hpx::components::simple_component_base<server> { void print_greeting () { hpx::cout << "Hey, how are you?\n" << hpx::flush; } // Component actions need to be declared, this also defines the // type 'print_greeting_action' representing the action. HPX_DEFINE_COMPONENT_ACTION(server, print_greeting, print_greeting_action); }; } // Declare boilerplate code required for each of the component actions. HPX_REGISTER_ACTION_DECLARATION(app::server::print_greeting_action);
- Example:
- Note
- This macro has to be used once for each of the component actions defined using one of the HPX_DEFINE_COMPONENT_ACTION macros. It has to be visible in all translation units using the action, thus it is recommended to place it into the header file defining the component.
-
HPX_REGISTER_ACTION
(...)¶ Define the necessary component action boilerplate code.
The macro HPX_REGISTER_ACTION can be used to define all the boilerplate code which is required for proper functioning of component actions in the context of HPX.
The parameter action is the type of the action to define the boilerplate for.
This macro can be invoked with an optional second parameter. This parameter specifies a unique name of the action to be used for serialization purposes. The second parameter has to be specified if the first parameter is not usable as a plain (non-qualified) C++ identifier, i.e. the first parameter contains special characters which cannot be part of a C++ identifier, such as ‘<’, ‘>’, or ‘:’.
- Note
- This macro has to be used once for each of the component actions defined using one of the HPX_DEFINE_COMPONENT_ACTION or HPX_DEFINE_PLAIN_ACTION macros. It has to occur exactly once for each of the actions, thus it is recommended to place it into the source file defining the component.
- Note
- Only one of the forms of this macro HPX_REGISTER_ACTION or HPX_REGISTER_ACTION_ID should be used for a particular action, never both.
-
HPX_REGISTER_ACTION_ID
(action, actionname, actionid)¶ Define the necessary component action boilerplate code and assign a predefined unique id to the action.
The macro HPX_REGISTER_ACTION can be used to define all the boilerplate code which is required for proper functioning of component actions in the context of HPX.
The parameter action is the type of the action to define the boilerplate for.
The parameter actionname specifies an unique name of the action to be used for serialization purposes. The second parameter has to be usable as a plain (non-qualified) C++ identifier, it should not contain special characters which cannot be part of a C++ identifier, such as ‘<’, ‘>’, or ‘:’.
The parameter actionid specifies an unique integer value which will be used to represent the action during serialization.
- Note
- This macro has to be used once for each of the component actions defined using one of the HPX_DEFINE_COMPONENT_ACTION or global actions HPX_DEFINE_PLAIN_ACTION macros. It has to occur exactly once for each of the actions, thus it is recommended to place it into the source file defining the component.
- Note
- Only one of the forms of this macro HPX_REGISTER_ACTION or HPX_REGISTER_ACTION_ID should be used for a particular action, never both.
-
-
file
component_action.hpp
- #include <hpx/config.hpp>#include <hpx/preprocessor/cat.hpp>#include <hpx/preprocessor/expand.hpp>#include <hpx/preprocessor/nargs.hpp>#include <hpx/runtime/actions/basic_action.hpp>#include <hpx/runtime/components/pinned_ptr.hpp>#include <hpx/runtime/naming/address.hpp>#include <hpx/traits/is_future.hpp>#include <boost/utility/string_ref.hpp>#include <cstdlib>#include <sstream>#include <string>#include <type_traits>#include <utility>#include <hpx/config/warnings_prefix.hpp>#include <hpx/config/warnings_suffix.hpp>
Defines
-
HPX_DEFINE_COMPONENT_ACTION
(...)¶ Registers a member function of a component as an action type with HPX.
The macro HPX_DEFINE_COMPONENT_ACTION can be used to register a member function of a component as an action type named action_type.
The parameter component is the type of the component exposing the member function func which should be associated with the newly defined action type. The parameter
action_type
is the name of the action type to register with HPX.namespace app { // Define a simple component exposing one action 'print_greeting' class HPX_COMPONENT_EXPORT server : public hpx::components::simple_component_base<server> { void print_greeting() const { hpx::cout << "Hey, how are you?\n" << hpx::flush; } // Component actions need to be declared, this also defines the // type 'print_greeting_action' representing the action. HPX_DEFINE_COMPONENT_ACTION(server, print_greeting, print_greeting_action); }; }
- Example:
The first argument must provide the type name of the component the action is defined for.
The second argument must provide the member function name the action should wrap.
The default value for the third argument (the typename of the defined action) is derived from the name of the function (as passed as the second argument) by appending ‘_action’. The third argument can be omitted only if the second argument with an appended suffix ‘_action’ resolves to a valid, unqualified C++ type name.
- Note
- The macro HPX_DEFINE_COMPONENT_ACTION can be used with 2 or 3 arguments. The third argument is optional.
-
-
file
plain_action.hpp
- #include <hpx/config.hpp>#include <hpx/preprocessor/cat.hpp>#include <hpx/preprocessor/expand.hpp>#include <hpx/preprocessor/nargs.hpp>#include <hpx/preprocessor/strip_parens.hpp>#include <hpx/runtime/actions/basic_action.hpp>#include <hpx/runtime/naming/address.hpp>#include <hpx/traits/component_type_database.hpp>#include <hpx/util/assert.hpp>#include <boost/utility/string_ref.hpp>#include <cstdlib>#include <sstream>#include <stdexcept>#include <string>#include <utility>#include <hpx/config/warnings_prefix.hpp>#include <hpx/config/warnings_suffix.hpp>
Defines
-
HPX_DEFINE_PLAIN_ACTION
(...)¶ Defines a plain action type.
namespace app { void some_global_function(double d) { cout << d; } // This will define the action type 'app::some_global_action' which // represents the function 'app::some_global_function'. HPX_DEFINE_PLAIN_ACTION(some_global_function, some_global_action); }
- Example:
- Note
- Usually this macro will not be used in user code unless the intent is to avoid defining the action_type in global namespace. Normally, the use of the macro HPX_PLAIN_ACTION is recommended.
- Note
- The macro HPX_DEFINE_PLAIN_ACTION can be used with 1 or 2 arguments. The second argument is optional. The default value for the second argument (the typename of the defined action) is derived from the name of the function (as passed as the first argument) by appending ‘_action’. The second argument can be omitted only if the first argument with an appended suffix ‘_action’ resolves to a valid, unqualified C++ type name.
-
HPX_DECLARE_PLAIN_ACTION
(...)¶ Declares a plain action type.
-
HPX_PLAIN_ACTION
(...)¶ Defines a plain action type based on the given function func and registers it with HPX.
The macro HPX_PLAIN_ACTION can be used to define a plain action (e.g. an action encapsulating a global or free function) based on the given function func. It defines the action type name representing the given function. This macro additionally registers the newly define action type with HPX.
The parameter
func
is a global or free (non-member) function which should be encapsulated into a plain action. The parametername
is the name of the action type defined by this macro.namespace app { void some_global_function(double d) { cout << d; } } // This will define the action type 'some_global_action' which represents // the function 'app::some_global_function'. HPX_PLAIN_ACTION(app::some_global_function, some_global_action);
- Example:
- Note
- The macro HPX_PLAIN_ACTION has to be used at global namespace even if the wrapped function is located in some other namespace. The newly defined action type is placed into the global namespace as well.
- Note
- The macro HPX_PLAIN_ACTION_ID can be used with 1, 2, or 3 arguments. The second and third arguments are optional. The default value for the second argument (the typename of the defined action) is derived from the name of the function (as passed as the first argument) by appending ‘_action’. The second argument can be omitted only if the first argument with an appended suffix ‘_action’ resolves to a valid, unqualified C++ type name. The default value for the third argument is hpx::components::factory_check.
- Note
- Only one of the forms of this macro HPX_PLAIN_ACTION or HPX_PLAIN_ACTION_ID should be used for a particular action, never both.
-
HPX_PLAIN_ACTION_ID
(func, name, id)¶ Defines a plain action type based on the given function func and registers it with HPX.
The macro HPX_PLAIN_ACTION_ID can be used to define a plain action (e.g. an action encapsulating a global or free function) based on the given function func. It defines the action type actionname representing the given function. The parameter actionid
The parameter actionid specifies an unique integer value which will be used to represent the action during serialization.
The parameter
func
is a global or free (non-member) function which should be encapsulated into a plain action. The parametername
is the name of the action type defined by this macro.The second parameter has to be usable as a plain (non-qualified) C++ identifier, it should not contain special characters which cannot be part of a C++ identifier, such as ‘<’, ‘>’, or ‘:’.
namespace app { void some_global_function(double d) { cout << d; } } // This will define the action type 'some_global_action' which represents // the function 'app::some_global_function'. HPX_PLAIN_ACTION_ID(app::some_global_function, some_global_action, some_unique_id);
- Example:
- Note
- The macro HPX_PLAIN_ACTION_ID has to be used at global namespace even if the wrapped function is located in some other namespace. The newly defined action type is placed into the global namespace as well.
- Note
- Only one of the forms of this macro HPX_PLAIN_ACTION or HPX_PLAIN_ACTION_ID should be used for a particular action, never both.
-
-
file
applier_fwd.hpp
- #include <hpx/config.hpp>
-
file
basename_registration_fwd.hpp
- #include <hpx/config.hpp>#include <hpx/components_fwd.hpp>#include <hpx/lcos_fwd.hpp>#include <hpx/runtime/components/make_client.hpp>#include <hpx/runtime/naming/id_type.hpp>#include <cstddef>#include <string>#include <utility>#include <vector>
-
file
binpacking_distribution_policy.hpp
- #include <hpx/config.hpp>#include <hpx/dataflow.hpp>#include <hpx/lcos/future.hpp>#include <hpx/performance_counters/performance_counter.hpp>#include <hpx/runtime/components/client_base.hpp>#include <hpx/runtime/launch_policy.hpp>#include <hpx/util/bind_front.hpp>#include <hpx/performance_counters/counters_fwd.hpp>#include <hpx/performance_counters/stubs/performance_counter.hpp>#include <hpx/performance_counters/server/base_performance_counter.hpp>#include <hpx/lcos/base_lco_with_value.hpp>#include <hpx/performance_counters/counters.hpp>#include <hpx/performance_counters/performance_counter_base.hpp>#include <hpx/runtime/actions/component_action.hpp>#include <hpx/runtime/components/component_type.hpp>#include <hpx/runtime/components/server/component.hpp>#include <hpx/throw_exception.hpp>#include <hpx/util/atomic_count.hpp>#include <hpx/runtime/components/stubs/stub_base.hpp>#include <string>#include <utility>#include <vector>#include <hpx/runtime/find_here.hpp>#include <hpx/runtime/naming/id_type.hpp>#include <hpx/runtime/naming/name.hpp>#include <hpx/runtime/serialization/serialization_fwd.hpp>#include <hpx/runtime/serialization/string.hpp>#include <hpx/runtime/serialization/vector.hpp>#include <hpx/traits/is_distribution_policy.hpp>#include <hpx/util/assert.hpp>#include <hpx/util/bind_back.hpp>#include <hpx/util/unwrap.hpp>#include <algorithm>#include <cstddef>#include <cstdint>#include <iterator>#include <type_traits>
-
file
colocating_distribution_policy.hpp
- #include <hpx/config.hpp>#include <hpx/lcos/detail/async_colocated.hpp>#include <hpx/lcos/detail/async_colocated_callback.hpp>#include <hpx/lcos/detail/async_implementations.hpp>#include <hpx/lcos/future.hpp>#include <hpx/runtime/applier/detail/apply_colocated_callback_fwd.hpp>#include <hpx/runtime/applier/detail/apply_colocated_fwd.hpp>#include <hpx/runtime/applier/detail/apply_implementations.hpp>#include <hpx/runtime/components/client_base.hpp>#include <hpx/runtime/components/stubs/stub_base.hpp>#include <hpx/runtime/launch_policy.hpp>#include <hpx/runtime/find_here.hpp>#include <hpx/runtime/naming/id_type.hpp>#include <hpx/runtime/naming/name.hpp>#include <hpx/runtime/serialization/serialization_fwd.hpp>#include <hpx/traits/extract_action.hpp>#include <hpx/traits/is_distribution_policy.hpp>#include <hpx/traits/promise_local_result.hpp>#include <algorithm>#include <cstddef>#include <type_traits>#include <utility>#include <vector>
-
file
component_factory.hpp
Defines
-
HPX_REGISTER_COMPONENT
(type, name, mode)¶ Define a component factory for a component type.
This macro is used create and to register a minimal component factory for a component type which allows it to be remotely created using the hpx::new_<> function.
This macro can be invoked with one, two or three arguments
- Parameters
type
: The type parameter is a (fully decorated) type of the component type for which a factory should be defined.name
: The name parameter specifies the name to use to register the factory. This should uniquely (system-wide) identify the component type. The name parameter must conform to the C++ identifier rules (without any namespace). If this parameter is not given, the first parameter is used.mode
: The mode parameter has to be one of the defined enumeration values of the enumeration hpx::components::factory_state_enum. The default for this parameter is hpx::components::factory_enabled.
-
-
file
copy_component.hpp
- #include <hpx/config.hpp>#include <hpx/lcos/async.hpp>#include <hpx/lcos/detail/async_colocated.hpp>#include <hpx/lcos/future.hpp>#include <hpx/runtime/actions/plain_action.hpp>#include <hpx/runtime/components/server/copy_component.hpp>#include <hpx/runtime/naming/name.hpp>#include <hpx/traits/is_component.hpp>#include <type_traits>
-
file
default_distribution_policy.hpp
- #include <hpx/config.hpp>#include <hpx/lcos/dataflow.hpp>#include <hpx/lcos/future.hpp>#include <hpx/lcos/packaged_action.hpp>#include <hpx/runtime/actions/action_support.hpp>#include <hpx/runtime/applier/apply.hpp>#include <hpx/runtime/components/stubs/stub_base.hpp>#include <hpx/runtime/launch_policy.hpp>#include <hpx/runtime/find_here.hpp>#include <hpx/runtime/naming/id_type.hpp>#include <hpx/runtime/naming/name.hpp>#include <hpx/runtime/serialization/serialization_fwd.hpp>#include <hpx/runtime/serialization/vector.hpp>#include <hpx/runtime/serialization/shared_ptr.hpp>#include <hpx/traits/extract_action.hpp>#include <hpx/traits/is_distribution_policy.hpp>#include <hpx/traits/promise_local_result.hpp>#include <hpx/util/assert.hpp>#include <algorithm>#include <cstddef>#include <memory>#include <type_traits>#include <utility>#include <vector>
-
file
migrate_component.hpp
- #include <hpx/config.hpp>#include <hpx/lcos/async.hpp>#include <hpx/lcos/detail/async_colocated.hpp>#include <hpx/lcos/future.hpp>#include <hpx/runtime/actions/plain_action.hpp>#include <hpx/runtime/components/client_base.hpp>#include <hpx/runtime/components/server/migrate_component.hpp>#include <hpx/runtime/components/target_distribution_policy.hpp>#include <hpx/lcos/dataflow.hpp>#include <hpx/lcos/detail/async_implementations_fwd.hpp>#include <hpx/lcos/packaged_action.hpp>#include <hpx/runtime/actions/action_support.hpp>#include <hpx/runtime/agas/interface.hpp>#include <hpx/runtime/applier/detail/apply_implementations_fwd.hpp>#include <hpx/runtime/components/stubs/stub_base.hpp>#include <hpx/runtime/find_here.hpp>#include <hpx/runtime/launch_policy.hpp>#include <hpx/runtime/naming/id_type.hpp>#include <hpx/runtime/naming/name.hpp>#include <hpx/runtime/serialization/serialization_fwd.hpp>#include <hpx/traits/extract_action.hpp>#include <hpx/traits/is_distribution_policy.hpp>#include <hpx/traits/promise_local_result.hpp>#include <algorithm>#include <cstddef>#include <type_traits>#include <utility>#include <vector>#include <hpx/traits/is_component.hpp>
-
file
new.hpp
- #include <hpx/config.hpp>#include <hpx/lcos/future.hpp>#include <hpx/runtime/components/client_base.hpp>#include <hpx/runtime/components/default_distribution_policy.hpp>#include <hpx/runtime/components/server/create_component.hpp>#include <hpx/runtime/components/stubs/stub_base.hpp>#include <hpx/runtime/launch_policy.hpp>#include <hpx/runtime/naming/name.hpp>#include <hpx/traits/is_client.hpp>#include <hpx/traits/is_component.hpp>#include <hpx/traits/is_distribution_policy.hpp>#include <hpx/util/lazy_enable_if.hpp>#include <algorithm>#include <cstddef>#include <type_traits>#include <utility>#include <vector>
-
file
find_here.hpp
- #include <hpx/config.hpp>#include <hpx/exception_fwd.hpp>#include <hpx/runtime/naming/id_type.hpp>
-
file
find_localities.hpp
- #include <hpx/config.hpp>#include <hpx/exception_fwd.hpp>#include <hpx/runtime/components/component_type.hpp>#include <hpx/runtime/naming/id_type.hpp>#include <vector>
-
file
get_colocation_id.hpp
- #include <hpx/exception_fwd.hpp>#include <hpx/lcos_fwd.hpp>#include <hpx/runtime/launch_policy.hpp>#include <hpx/runtime/naming/id_type.hpp>
-
file
get_locality_id.hpp
- #include <hpx/config.hpp>#include <hpx/exception_fwd.hpp>#include <cstdint>
-
file
get_locality_name.hpp
- #include <hpx/config.hpp>#include <hpx/lcos_fwd.hpp>#include <hpx/runtime/naming/id_type.hpp>#include <string>
-
file
get_num_localities.hpp
- #include <hpx/config.hpp>#include <hpx/exception_fwd.hpp>#include <hpx/lcos_fwd.hpp>#include <hpx/runtime/launch_policy.hpp>#include <hpx/runtime/components/component_type.hpp>#include <cstdint>
-
file
get_os_thread_count.hpp
- #include <hpx/config.hpp>#include <hpx/runtime/threads/thread_data_fwd.hpp>#include <cstddef>
-
file
get_ptr.hpp
- #include <hpx/config.hpp>#include <hpx/runtime_fwd.hpp>#include <hpx/runtime/agas/gva.hpp>#include <hpx/runtime/components/client_base.hpp>#include <hpx/runtime/components/component_type.hpp>#include <hpx/runtime/get_lva.hpp>#include <hpx/runtime/launch_policy.hpp>#include <hpx/runtime/naming/address.hpp>#include <hpx/runtime/naming/name.hpp>#include <hpx/throw_exception.hpp>#include <hpx/traits/component_pin_support.hpp>#include <hpx/traits/component_type_is_compatible.hpp>#include <hpx/util/assert.hpp>#include <hpx/util/bind_back.hpp>#include <memory>
-
file
get_thread_name.hpp
- #include <hpx/config.hpp>#include <hpx/util/itt_notify.hpp>#include <string>
-
file
get_worker_thread_num.hpp
- #include <hpx/config.hpp>#include <hpx/error_code.hpp>#include <cstddef>
-
file
launch_policy.hpp
- #include <hpx/config.hpp>#include <hpx/runtime/threads/thread_enums.hpp>#include <hpx/runtime/serialization/serialization_fwd.hpp>#include <type_traits>#include <utility>
-
file
unmanaged.hpp
- #include <hpx/runtime/naming/name.hpp>
-
file
report_error.hpp
- #include <hpx/config.hpp>#include <cstddef>#include <exception>
-
file
partitioner.hpp
- #include <hpx/config.hpp>#include <hpx/runtime/resource/partitioner_fwd.hpp>#include <hpx/runtime/resource/detail/create_partitioner.hpp>#include <hpx/runtime/runtime_mode.hpp>#include <hpx/runtime/threads/policies/scheduler_mode.hpp>#include <hpx/util/function.hpp>#include <boost/program_options.hpp>#include <cstddef>#include <string>#include <utility>#include <vector>
-
file
partitioner_fwd.hpp
- #include <hpx/config.hpp>#include <hpx/runtime/threads/policies/callback_notifier.hpp>#include <hpx/runtime/threads_fwd.hpp>#include <hpx/util/function.hpp>#include <cstddef>#include <memory>#include <string>
-
file
runtime_mode.hpp
- #include <hpx/config.hpp>#include <string>
-
file
set_parcel_write_handler.hpp
- #include <hpx/config.hpp>#include <hpx/exception_fwd.hpp>#include <hpx/runtime/parcelset_fwd.hpp>#include <hpx/util/function.hpp>#include <boost/system/error_code.hpp>
-
file
shutdown_function.hpp
- #include <hpx/config.hpp>#include <hpx/util/unique_function.hpp>
-
file
startup_function.hpp
- #include <hpx/config.hpp>#include <hpx/util/unique_function.hpp>
-
file
scheduler_mode.hpp
-
file
thread_data_fwd.hpp
- #include <hpx/config.hpp>#include <hpx/exception_fwd.hpp>#include <hpx/runtime/threads/coroutines/coroutine_fwd.hpp>#include <hpx/runtime/threads/thread_enums.hpp>#include <hpx/runtime/threads/thread_id_type.hpp>#include <hpx/util_fwd.hpp>#include <hpx/util/function.hpp>#include <hpx/util/unique_function.hpp>#include <cstddef>#include <cstdint>#include <utility>#include <memory>
-
file
thread_enums.hpp
- #include <hpx/config.hpp>#include <hpx/runtime/threads/detail/combined_tagged_state.hpp>#include <cstddef>#include <cstdint>
-
file
thread_helpers.hpp
- #include <hpx/config.hpp>#include <hpx/exception_fwd.hpp>#include <hpx/runtime/naming_fwd.hpp>#include <hpx/runtime/threads_fwd.hpp>#include <hpx/runtime/thread_pool_helpers.hpp>#include <hpx/runtime/threads/policies/scheduler_mode.hpp>#include <hpx/runtime/threads/thread_data_fwd.hpp>#include <hpx/runtime/threads/thread_enums.hpp>#include <hpx/util_fwd.hpp>#include <hpx/util/unique_function.hpp>#include <hpx/util/register_locks.hpp>#include <hpx/util/steady_clock.hpp>#include <hpx/util/thread_description.hpp>#include <atomic>#include <chrono>#include <cstddef>#include <cstdint>#include <type_traits>#include <utility>
-
file
thread_pool_base.hpp
- #include <hpx/config.hpp>#include <hpx/compat/barrier.hpp>#include <hpx/compat/condition_variable.hpp>#include <hpx/compat/mutex.hpp>#include <climits>#include <cstddef>#include <hpx/config/warnings_prefix.hpp>#include <hpx/config/warnings_suffix.hpp>#include <hpx/compat/thread.hpp>#include <hpx/error_code.hpp>#include <hpx/exception_fwd.hpp>#include <hpx/lcos/future.hpp>#include <hpx/lcos/local/no_mutex.hpp>#include <hpx/lcos/local/spinlock.hpp>#include <hpx/runtime/thread_pool_helpers.hpp>#include <hpx/runtime/threads/cpu_mask.hpp>#include <hpx/runtime/threads/policies/affinity_data.hpp>#include <hpx/runtime/threads/policies/callback_notifier.hpp>#include <hpx/runtime/threads/policies/scheduler_mode.hpp>#include <hpx/runtime/threads/thread_executor.hpp>#include <hpx/runtime/threads/thread_init_data.hpp>#include <hpx/runtime/threads/topology.hpp>#include <hpx/state.hpp>#include <hpx/util/steady_clock.hpp>#include <hpx/util_fwd.hpp>#include <cstdint>#include <exception>#include <functional>#include <iosfwd>#include <memory>#include <mutex>#include <string>#include <vector>
-
file
trigger_lco.hpp
- #include <hpx/config.hpp>#include <hpx/lcos_fwd.hpp>#include <hpx/runtime/actions/continuation_fwd.hpp>#include <hpx/runtime/actions/action_priority.hpp>#include <hpx/runtime/applier/detail/apply_implementations_fwd.hpp>#include <hpx/runtime/naming/address.hpp>#include <hpx/runtime/naming/name.hpp>#include <hpx/util/assert.hpp>#include <hpx/util/decay.hpp>#include <exception>#include <type_traits>#include <utility>
-
file
runtime_fwd.hpp
- #include <hpx/config.hpp>#include <hpx/exception_fwd.hpp>#include <hpx/runtime/basename_registration_fwd.hpp>#include <hpx/runtime/config_entry.hpp>#include <hpx/runtime/find_localities.hpp>#include <hpx/runtime/get_colocation_id.hpp>#include <hpx/runtime/get_locality_id.hpp>#include <hpx/runtime/get_locality_name.hpp>#include <hpx/runtime/get_num_localities.hpp>#include <hpx/runtime/get_os_thread_count.hpp>#include <hpx/runtime/get_thread_name.hpp>#include <hpx/runtime/get_worker_thread_num.hpp>#include <hpx/runtime/naming_fwd.hpp>#include <hpx/runtime/report_error.hpp>#include <hpx/runtime/runtime_fwd.hpp>#include <hpx/runtime/runtime_mode.hpp>#include <hpx/runtime/set_parcel_write_handler.hpp>#include <hpx/runtime/shutdown_function.hpp>#include <hpx/runtime/startup_function.hpp>#include <hpx/util/function.hpp>#include <hpx/util_fwd.hpp>#include <cstddef>#include <cstdint>#include <string>
-
file
throw_exception.hpp
- #include <hpx/config.hpp>#include <hpx/error.hpp>#include <hpx/exception_fwd.hpp>#include <hpx/preprocessor/cat.hpp>#include <hpx/preprocessor/expand.hpp>#include <hpx/preprocessor/nargs.hpp>#include <boost/current_function.hpp>#include <boost/system/error_code.hpp>#include <exception>#include <string>#include <hpx/config/warnings_prefix.hpp>#include <hpx/config/warnings_suffix.hpp>
Defines
-
HPX_THROW_EXCEPTION
(errcode, f, msg)¶ Throw a hpx::exception initialized from the given parameters.
The macro HPX_THROW_EXCEPTION can be used to throw a hpx::exception. The purpose of this macro is to prepend the source file name and line number of the position where the exception is thrown to the error message. Moreover, this associates additional diagnostic information with the exception, such as file name and line number, locality id and thread id, and stack backtrace from the point where the exception was thrown.
The parameter
errcode
holds the hpx::error code the new exception should encapsulate. The parameterf
is expected to hold the name of the function exception is thrown from and the parametermsg
holds the error message the new exception should encapsulate.void raise_exception() { // Throw a hpx::exception initialized from the given parameters. // Additionally associate with this exception some detailed // diagnostic information about the throw-site. HPX_THROW_EXCEPTION(hpx::no_success, "raise_exception", "simulated error"); }
- Example:
-
HPX_THROWS_IF
(ec, errcode, f, msg)¶ Either throw a hpx::exception or initialize hpx::error_code from the given parameters.
The macro HPX_THROWS_IF can be used to either throw a hpx::exception or to initialize a hpx::error_code from the given parameters. If &ec == &hpx::throws, the semantics of this macro are equivalent to HPX_THROW_EXCEPTION. If &ec != &hpx::throws, the hpx::error_code instance
ec
is initialized instead.The parameter
errcode
holds the hpx::error code from which the new exception should be initialized. The parameterf
is expected to hold the name of the function exception is thrown from and the parametermsg
holds the error message the new exception should encapsulate.
-
-
file
is_execution_policy.hpp
- #include <hpx/config.hpp>#include <hpx/util/decay.hpp>#include <type_traits>
-
file
checkpoint.hpp
- #include <hpx/dataflow.hpp>#include <hpx/lcos/future.hpp>#include <hpx/runtime/serialization/serialize.hpp>#include <hpx/runtime/serialization/vector.hpp>#include <cstddef>#include <fstream>#include <iosfwd>#include <sstream>#include <string>#include <type_traits>#include <utility>#include <vector>
This header defines the save_checkpoint and restore_checkpoint functions. These functions are designed to help HPX application developer’s checkpoint their applications. Save_checkpoint serializes one or more objects and saves them as a byte stream. Restore_checkpoint converts the byte stream back into instances of the objects.
-
file
debugging.hpp
- #include <hpx/config.hpp>
-
file
invoke.hpp
- #include <hpx/config.hpp>#include <hpx/util/result_of.hpp>#include <hpx/util/void_guard.hpp>#include <boost/ref.hpp>#include <functional>#include <type_traits>#include <utility>
-
file
invoke_fused.hpp
- #include <hpx/config.hpp>#include <hpx/util/detail/pack.hpp>#include <hpx/util/invoke.hpp>#include <hpx/util/result_of.hpp>#include <hpx/util/tuple.hpp>#include <hpx/util/void_guard.hpp>#include <cstddef>#include <type_traits>#include <utility>
-
file
pack_traversal.hpp
- #include <hpx/util/detail/pack_traversal_impl.hpp>#include <hpx/util/tuple.hpp>#include <type_traits>#include <utility>
-
file
pack_traversal_async.hpp
- #include <hpx/util/detail/pack_traversal_async_impl.hpp>#include <utility>
-
file
unwrap.hpp
- #include <hpx/config.hpp>#include <hpx/util/detail/unwrap_impl.hpp>#include <cstddef>#include <utility>
-
file
unwrapped.hpp
- #include <hpx/config.hpp>
-
dir
/hpx/source/hpx/runtime/actions
-
dir
/hpx/source/hpx/parallel/algorithms
-
dir
/hpx/source/hpx/components/component_storage
-
dir
/hpx/source/hpx/components
-
dir
/hpx/source/hpx/runtime/components
-
dir
/hpx/source/hpx/parallel/container_algorithms
-
dir
/hpx/source/hpx/parallel/executors
-
dir
/hpx/source/hpx
-
dir
/hpx/source/hpx/lcos
-
dir
/hpx/source/hpx/runtime/naming
-
dir
/hpx/source/hpx/parallel
-
dir
/hpx/source/hpx/performance_counters
-
dir
/hpx/source/hpx/runtime/threads/policies
-
dir
/hpx/source/hpx/runtime/resource
-
dir
/hpx/source/hpx/runtime
-
dir
/hpx/source
-
dir
/hpx/source/hpx/runtime/threads
-
dir
/hpx/source/hpx/traits
-
dir
/hpx/source/hpx/util
Modules reference¶
preprocessor¶
#include <hpx/preprocessor.hpp>¶
#include <hpx/preprocessor/expand.hpp>¶
Defines
-
HPX_PP_EXPAND
(X)¶ The HPX_PP_EXPAND macro performs a double macro-expansion on its argument.
This macro can be used to produce a delayed preprocessor expansion.
- Parameters
X
: Token to be expanded twice
Example:
#define MACRO(a, b, c) (a)(b)(c) #define ARGS() (1, 2, 3) HPX_PP_EXPAND(MACRO ARGS()) // expands to (1)(2)(3)
#include <hpx/preprocessor/cat.hpp>¶
Defines
-
HPX_PP_CAT
(A, B)¶ Concatenates the tokens
A
andB
into a single token. Evaluates toAB
- Parameters
A
: First tokenB
: Second token
#include <hpx/preprocessor/strip_parens.hpp>¶
Defines
-
HPX_PP_STRIP_PARENS
(X)¶ For any symbol
X
, this macro returns the same symbol from which potential outer parens have been removed. If no outer parens are found, this macros evaluates toX
itself without error.The original implementation of this macro is from Steven Watanbe as shown in http://boost.2283326.n4.nabble.com/preprocessor-removing-parentheses-td2591973.html#a2591976
HPX_PP_STRIP_PARENS(no_parens) HPX_PP_STRIP_PARENS((with_parens))
- Example Usage:
- Parameters
X
: Symbol to strip parens from
This produces the following output
no_parens with_parens
#include <hpx/preprocessor/stringize.hpp>¶
Defines
-
HPX_PP_STRINGIZE
(X)¶ The HPX_PP_STRINGIZE macro stringizes its argument after it has been expanded.
The passed argument
X
will expand to"X"
. Note that the stringizing operator (#) prevents arguments from expanding. This macro circumvents this shortcoming.- Parameters
X
: The text to be converted to a string literal
Contributing to HPX¶
HPX development happens on Github. The following sections are a collection of useful information related to HPX development.
Release procedure for HPX¶
Below is a step-wise procedure for making an HPX release. We aim to produce two releases per year: one in March-April, and one in September-October.
This is a living document and may not be totally current or accurate. It is an attempt to capture current practice in making an HPX release. Please update it as appropriate.
One way to use this procedure is to print a copy and check off the lines as they are completed to avoid confusion.
Notify developers that a release is imminent.
Make a list of examples and benchmarks that should not go into the release. Build all examples and benchmarks that will go in the release and make sure they build and run as expected.
- Make sure all examples and benchmarks have example input files, and usage documentation, either in the form of comments or a readme.
Send the list of examples and benchmarks that will be included in the release to hpx-users@stellar.cct.lsu.edu and stellar@cct.lsu.edu, and ask for feedback. Update the list as necessary.
Write release notes in
docs/sphinx/releases/whats_new_$VERSION.rst
. Keep adding merged PRs and closed issues to this until just before the release is made. Add the new release notes to the table of contents indocs/sphinx/releases.rst
.Build the docs, and proof-read them. Update any documentation that may have changed, and correct any typos. Pay special attention to:
$HPX_SOURCE/README.rst
- Update grant information
docs/sphinx/releases/whats_new_$VERSION.rst
docs/sphinx/about_hpx/people.rst
- Update collaborators
- Update grant information
This step does not apply to patch releases. For both APEX and hpxMP:
If there have been any commits to the release branch since the last release create a tag from the old release branch before deleting the old release branch in the next step.
Unprotect the release branch in the github repository settings so that it can be deleted and recreated.
Delete the old release branch, and create a new one by branching a stable point from master. If you are creating a patch release, branch from the release tag for which you want to create a patch release.
git push origin --delete release
git branch -D release
git checkout [stable point in master]
git branch release
git push origin release
git branch --set-upstream-to=origin/release release
Protect the release branch again to disable deleting and force pushes.
Check out the release branch.
Make sure
HPX_VERSION_MAJOR/MINOR/SUBMINOR
inCMakeLists.txt
contain the correct values. Change them if needed.Remove the examples and benchmarks that will not go into the release from the release branch.
This step does not apply to patch releases. Remove features which have been deprecated for at least 2 releases. This involves removing build options which enable those features from the main CMakeLists.txt and also deleting all related code and tests from the main source tree.
The general deprecation policy involves a three-step process we have to go through in order to introduce a breaking change
- First release cycle: add a build option which allows to explicitly disable any old (now deprecated) code.
- Second release cycle: turn this build option OFF by default.
- Third release cycle: completely remove the old code.
The main CMakeLists.txt contains a comment indicating for which version the breaking change was introduced first.
Switch Buildbot over to test the release branch
https://github.com/STEllAR-GROUP/hermione-buildbot/blob/rostam/master/master.cfg
branch
field inc['change_source'] = GitPoller
Repeat the following steps until satisfied with the release.
- Change
HPX_VERSION_TAG
inCMakeLists.txt
to-rcN
, whereN
is the current iteration of this step. Start with-rc1
. - Tag a release candidate from the release branch, where tag name is the
version to be released with a
-rcN
suffix and description is “HPX V$VERSION: The C++ Standards Library for Parallelism and Concurrency”.git tag -a [tag name] -m '[description]'
git push origin [tag name]
- Create a pre-release on GitHub
- This step is not necessary for patch releases. Notify
hpx-users@stellar.cct.lsu.edu
andstellar@cct.lsu.edu
of the availability of the release candidate. Ask users to test the candidate by checking out the release candidate tag. - Allow at least a week for testing of the release candidate.
- Use
git merge
when possible, and fall back togit cherry-pick
when needed. For patch releasesgit cherry-pick
is most likely your only choice if there have been significant unrelated changes on master since the previous release. - Go back to the first step when enough patches have been added.
- If there are no more patches continue to make the final release.
- Use
- Change
Update any occurrences of the latest stable release to refer to the version about to be released. For example,
quickstart.rst
contains instructions to check out the latest stable tag. Make sure that refers to the new version.Add a new entry to the RPM changelog (
cmake/packaging/rpm/Changelog.txt
) with the new version number and a link to the corresponding changelog.Change
HPX_VERSION_TAG
inCMakeLists.txt
to an empty string.Add the release date to the caption of the current “What’s New” section in the docs, and change the value of
HPX_VERSION_DATE
inCMakeLists.txt
.Tag the release from the release branch, where tag name is the version to be released and description is “HPX V$VERSION: The C++ Standards Library for Parallelism and Concurrency”. Sign the release tag with the
contact@stellar-group.org
key by adding the-s
flag togit tag
. Make sure you change git to sign with thecontact@stellar-group.org
key, rather than your own key if you use one. You also need to change the name and email used for commits. Change them toSTE||AR Group
andcontact@stellar-group.org
, respectively. Finally, thecontact@stellar-group.org
email address needs to be added to your GitHub account for the tag to show up as verified.git tag -s -a [tag name] -m '[description]'
git push origin [tag name]
Create a release on GitHub
- Refer to the ‘What’s New’ section in the documentation you uploaded in the notes for the Github release (see previous releases for a hint).
- A DOI number using Zenodo is automatically assigned once the release is created as such on github.
- Verify on Zenodo (https://zenodo.org/) that release was uploaded. Logging into zenodo using the github credentials might be necessary to see the new release as it usually takes a while for it to propagate to the search engine used on zenodo.
Roll a release candidate using
tools/roll_release.sh
(from root directory), and add the hashsums generated by the script to the “downloads” page of the website.Upload the packages the website. Use the following formats:
http://stellar.cct.lsu.edu/files/hpx_#.#.#.zip http://stellar.cct.lsu.edu/files/hpx_#.#.#.tar.gz http://stellar.cct.lsu.edu/files/hpx_#.#.#.tar.bz2 http://stellar.cct.lsu.edu/files/hpx_#.#.#.7z
Update the websites (stellar-group.org and stellar.cct.lsu.edu) with the following:
- Download links on the download page
- Documentation links on the docs page (link to generated documentation on GitHub Pages)
- A new blog post announcing the release, which links to downloads and the “What’s New” section in the documentation (see previous releases for examples)
Merge release branch into master.
This step does not apply to patch releases. Create a new branch from master, and check that branch out (name it for example by the next version number). Bump the HPX version to the next release target. The following files contain version info:
CMakeLists.txt
- Grep for old version number
- Create a new “What’s New” section for the docs of the next anticipated release. Set the date to “unreleased”.
- Update
$HPX_SOURCE/README.rst
- Update version (to the about-to-be-released version)
- Update links to documentation
- Fix zenodo reference number
- Merge new branch containing next version numbers to master, resolve conflicts if necessary.
Switch Buildbot back to test the main branch
https://github.com/STEllAR-GROUP/hermione-buildbot/blob/rostam/master/master.cfg
branch
field inc['change_source'] = GitPoller
Update Vcpkg (
https://github.com/Microsoft/vcpkg
) to pull from latest release.- Update version number in CONTROL
- Update tag and SHA512 to that of the new release
Announce the release on hpx-users@stellar.cct.lsu.edu, stellar@cct.lsu.edu, allcct@cct.lsu.edu, faculty@csc.lsu.edu, faculty@ece.lsu.edu, xpress@crest.iu.edu, the HPX Slack channel, the IRC channel, Sonia Sachs, our list of external collaborators, isocpp.org, reddit.com, HPC Wire, Inside HPC, Heise Online, and a CCT press release.
Beer and pizza.
Testing HPX¶
To ensure correctness of HPX we ship a large variety of unit and regression tests. The tests are driven by the CTest tool and are executed automatically by buildbot (see HPX Buildbot Website) on each commit to the HPX Github repository. In addition, it is encouraged to run the test suite manually to ensure proper operation on your target system. If a test fails for your platform, we highly recommend submitting an issue on our HPX Issues tracker with detailed information about the target system.
Running tests manually¶
Running the tests manually is as easy as typing make tests && make test
.
This will build all tests and run them once the tests are built successfully.
After the tests have been built, you can invoke separate tests with the help of
the ctest
command. You can list all available test targets using make help
| grep tests
. Please see the CTest Documentation for further details.
Issue tracker¶
If you stumble over a bug or missing feature missing feature in HPX please submit an issue to our HPX Issues. For more information on how to submit support requests or other means of getting in contact with the developers please see the Support Website.
Continuous testing¶
In addition to manual testing, we run automated tests on various platforms. You can see the status of the current master head by visiting the HPX Buildbot Website. We also run tests on all pull requests using both CircleCI and a combination of CDash and pycicle. You can see the dashboards here: CircleCI HPX dashboard and CDash HPX dashboard .
Using docker for development¶
Although it can often be useful to set up a local development environment with system-provided or self-built dependencies, Docker provides a convenient alternative to quickly get all the dependencies needed to start development of HPX. Our testing setup on CircleCI uses a docker image to run all tests.
To get started you need to install Docker using whatever means is most convenient on your system. Once you have Docker installed you can pull or directly run the docker image. The image is based on Debian and Clang, and can be found on Docker Hub. To start a container using the HPX build environment run:
docker run --interactive --tty stellargroup/build_env:ubuntu bash
You are now in an environment where all the HPX build and runtime dependencies are present. You can install additional packages according to your own needs. Please see the Docker Documentation for more information on using Docker.
Warning
All changes made within the container are lost when the container is closed. If you want files to persist (e.g. the HPX source tree) after closing the container you can bind directories from the host system into the container (see Docker Documentation (Bind mounts)).
Documentation¶
This documentation is built using Sphinx, and an automatically generated API reference using Doxygen and Breathe.
We always welcome suggestions on how to improve our documentation, as well as pull requests with corrections and additions.
Building documentation¶
Please see the documentation prerequisites
section for details on what you need in order to build the HPX documentation.
Enable building of the documentation by setting HPX_WITH_DOCUMENTATION=ON
during CMake configuration. To build the documentation build the docs
target using your build tool. The default output format is HTML documentation.
You can choose alternative output formats (single-page HTML, PDF, and man) with
the HPX_WITH_DOCUMENTATION_OUTPUT_FORMATS
CMake option.
Note
If you add new source files to the Sphinx documentation you have to run CMake again to have the files included in the build.
Style guide¶
The documentation is written using reStructuredText. These are the conventions used for formatting the documentation:
- Use at most 80 characters per line.
- Top-level headings use over- and underlines with
=
. - Sub-headings use only underlines with characters in decreasing level of
importance:
=
,-
and.
. - Use sentence case in headings.
- Refer to common terminology using
:term:`Component`
. - Indent content of directives (
.. directive::
) by three spaces. - For C++ code samples at the end of paragraphs, use
::
and indent the code sample by 4 spaces.- For other languages (or if you don’t want a colon at the end of the
paragraph) use
.. code-block:: language
and indent by three spaces as with other directives.
- For other languages (or if you don’t want a colon at the end of the
paragraph) use
- Use
.. list-table::
to wrap tables with a lot of text in cells.
Releases¶
HPX V1.3.0 (May 23, 2019)¶
General changes¶
- Performance improvements: the schedulers have significantly reduced overheads from removing false sharing and the parallel executor has been updated to create fewer futures.
- HPX now defaults to not turning on networking when running on one locality. This means that you can run multiple instances on the same system without adding command line options.
- Multiple issues reported by Clang sanitizers have been fixed.
- We have added (back) single-page HTML documentation and PDF documentation.
- We have started modularizing the HPX library. This is useful both for developers and users. In the long term users will be able to consume only parts of the HPX libraries if they do not require all the functionality that HPX currently provides.
- We have added an implementation of
function_ref
. - The
barrier
andlatch
classes have gained a few additional member functions.
Breaking changes¶
- Executable and library targets are now created without the
_exe
and_lib
suffix respectively. For example, the target1d_stencil_1_exe
is now simply called1d_stencil_1
. - We have removed the following deprecated functionality:
queue
,scoped_unlock
, and support for input iterators in algorithms. - We have turned off the compatibility layer for
unwrapped
by default. The functionality will be removed in the next release. The option can still be turned on using the CMake optionHPX_WITH_UNWRAPPED_SUPPORT
. Likewise,inclusive_scan
compatibility overloads have been turned off by default. They can still be turned on withHPX_WITH_INCLUSIVE_SCAN_COMPATIBILITY
. - The minimum compiler and dependency versions have been updated. We now support GCC from version 5 onwards, Clang from version 4 onwards, and Boost from version 1.61.0 onwards.
- The headers for preprocessor macros have moved as a result of the
functionality being moved to a separate module. The old headers are deprecated
and will be removed in a future version of HPX. You can turn off the warnings
by setting
HPX_PREPROCESSOR_WITH_DEPRECATION_WARNINGS=OFF
or turn off the compatibility headers completely withHPX_PREPROCESSOR_WITH_COMPATIBILITY_HEADERS=OFF
.
Closed issues¶
- Issue #3863 - shouldn’t “-faligned-new” be a usage requirement?
- Issue #3841 - Build error with msvc 19 caused by SFINAE and C++17
- Issue #3836 - master branch does not build with idle rate counters enabled
- Issue #3819 - Add debug suffix to modules built in debug mode
- Issue #3817 -
HPX_INCLUDE_DIRS
contains non-existent directory - Issue #3810 - Source groups are not created for files in modules
- Issue #3805 - HPX won’t compile with
-DHPX_WITH_APEX=TRUE
- Issue #3792 - Barrier Hangs When Locality Zero not included
- Issue #3778 - Replace
throw()
withnoexcept
- Issue #3763 - configurable sort limit per task
- Issue #3758 - dataflow doesn’t convert
future<future<T>>
tofuture<T>
- Issue #3757 - When compiling undefined reference to
hpx::hpx_check_version_1_2
HPX V1.2.1, Ubuntu 18.04.01 Server Edition - Issue #3753 -
--hpx:list-counters=full
crashes - Issue #3746 - Detection of MPI with pmix
- Issue #3744 - Separate spinlock from same cacheline as internal data for all LCOs
- Issue #3743 - hpxcxx’s shebang doesn’t specify the python version
- Issue #3738 - Unable to debug parcelport on a single node
- Issue #3735 - Latest master: Can’t compile in MSVC
- Issue #3731 -
util::bound
seems broken on Clang with older libstdc++ - Issue #3724 - Allow to pre-set command line options through environment
- Issue #3723 - examples/resource_partitioner build issue on master branch / ubuntu 18
- Issue #3721 - faced a building error
- Issue #3720 - Hello World example fails to link
- Issue #3719 - pkg-config produces invalid output:
-l-pthread
- Issue #3718 - Please make the python executable configurable through cmake
- Issue #3717 - interested to contribute to the organisation
- Issue #3699 - Remove ‘HPX runtime’ executable
- Issue #3698 - Ignore all locks while handling asserts
- Issue #3689 - Incorrect and inconsistent website structure http://stellar.cct.lsu.edu/downloads/.
- Issue #3681 - Broken links on http://stellar.cct.lsu.edu/2015/05/hpx-archives-now-on-gmane/
- Issue #3676 - HPX master built from source, cmake fails to link main.cpp example in docs
- Issue #3673 - HPX build fails with
std::atomic
missing error - Issue #3670 - Generate PDF again from documention (with Sphinx)
- Issue #3643 - Warnings when compiling HPX 1.2.1 with gcc 9
- Issue #3641 - Trouble with using ranges-v3 and
hpx::parallel::reduce
- Issue #3639 -
util::unwrapping
does not work well with member functions - Issue #3634 - The build fails if
shared_future<>::then
is called with a thread executor - Issue #3622 - VTune Amplifier 2019 not working with
use_itt_notify=1
- Issue #3616 - HPX Fails to Build with CUDA 10
- Issue #3612 - False sharing of scheduling counters
- Issue #3609 - executor_parameters timeout with gcc <= 7 and Debug mode
- Issue #3601 - Missleading error message on power pc for rdtsc and rdtscp
- Issue #3598 - Build of some examples fails when using Vc
- Issue #3594 - Error: The number of OS threads requested (20) does not match the number of threads to bind (12): HPX(bad_parameter)
- Issue #3592 - Undefined Reference Error
- Issue #3589 - include could not find load file: HPX_Utils.cmake
- Issue #3587 - HPX won’t compile on POWER8 with Clang 7
- Issue #3583 - Fedora and openSUSE instructions missing on “Distribution Packages” page
- Issue #3578 - Build error when configuring with
HPX_HAVE_ALGORITHM_INPUT_ITERATOR_SUPPORT=ON
- Issue #3575 - Merge openSUSE reproducible patch
- Issue #3570 - Update HPX to work with the latest VC version
- Issue #3567 - Build succeed and make failed for
hpx:cout
- Issue #3565 - Polymorphic simple component destructor not getting called
- Issue #3559 - 1.2.0 is missing from download page
- Issue #3554 - Clang 6.0 warning of hiding overloaded virtual function
- Issue #3510 - Build on ppc64 fails
- Issue #3482 - Improve error message when
HPX_WITH_MAX_CPU_COUNT
is too low for given system - Issue #3453 - Two HPX applications can’t run at the same time.
- Issue #3452 - Scaling issue on the change to 2 NUMA domains
- Issue #3442 - HPX set_difference, set_intersection failure cases
- Issue #3437 - Ensure parent_task pointer when child task is created and child/parent are on same locality
- Issue #3255 - Suspension with lock for
--hpx:list-component-types
- Issue #3034 - Use C++17 structured bindings for serialization
- Issue #2999 - Change thread scheduling use of
size_t
for thread indexing
Closed pull requests¶
- PR #3865 - adds hpx_target_compile_option_if_available
- PR #3864 - Helper functions that are useful in numa binding and testing of allocator
- PR #3862 - Temporary fix to local_dataflow_boost_small_vector test
- PR #3860 - Add cache line padding to intermediate results in for loop reduction
- PR #3859 - Remove HPX_TLL_PUBLIC and HPX_TLL_PRIVATE from CMake files
- PR #3858 - Add compile flags and definitions to modules
- PR #3851 - update hpxmp release tag to v0.2.0
- PR #3849 - Correct BOOST_ROOT variable name in quick start guide
- PR #3847 - Fix attach_debugger configuration option
- PR #3846 - Add tests for libs header tests
- PR #3844 - Fixing source_groups in preprocessor module to properly handle compatibility headers
- PR #3843 - This fixes the launch_process/launched_process pair of tests
- PR #3842 - Fix macro call with ITTNOTIFY enabled
- PR #3840 - Fixing SLURM environment parsing
- PR #3837 - Fixing misplaced #endif
- PR #3835 - make all latch members protected for consistency
- PR #3834 - Disable transpose_block_numa example on CircleCI
- PR #3833 - make latch counter_ protected for deriving latch in hpxmp
- PR #3831 - Fix CircleCI config for modules
- PR #3830 - minor fix: option HPX_WITH_TEST was not working correctly
- PR #3828 - Avoid for binaries that depend on HPX to directly link against internal modules
- PR #3827 - Adding shortcut for
hpx::get_ptr<>(sync, id)
for a local, non-migratable objects - PR #3826 - Fix and update modules documentation
- PR #3825 - Updating default APEX version to 2.1.3 with HPX
- PR #3823 - Fix pkgconfig libs handling
- PR #3822 - Change includes in hpx_wrap.cpp to more specific includes
- PR #3821 - Disable barrier_3792 test when networking is disabled
- PR #3820 - Assorted CMake fixes
- PR #3815 - Removing left-over debug output
- PR #3814 - Allow setting default scheduler mode via the configuration database
- PR #3813 - Make the deprecation warnings issued by the old pp headers optional
- PR #3812 - Windows requires to handle symlinks to directories differently from those linking files
- PR #3811 - Clean up PP module and library skeleton
- PR #3806 - Moving include path configuration to before APEX
- PR #3804 - Fix latch
- PR #3803 - Update hpxcxx to look at lib64 and use python3
- PR #3802 - Numa binding allocator
- PR #3801 - Remove duplicated includes
- PR #3800 - Attempt to fix Posix context switching after lazy init changes
- PR #3798 - count and count_if accepts different iterator types
- PR #3797 - Adding a couple of
override
keywords to overloaded virtual functions - PR #3796 - Re-enable testing all schedulers in shutdown_suspended_test
- PR #3795 - Change
std::terminate
to std::abort inSIGSEGV
handler - PR #3794 - Fixing #3792
- PR #3793 - Extending migrate_polymorphic_component unit test
- PR #3791 - Change
throw()
tonoexcept
- PR #3790 - Remove deprecated options for 1.3.0 release
- PR #3789 - Remove Boost filesystem compatibility header
- PR #3788 - Disabled even more spots that should not execute if networking is disabled
- PR #3787 - Bump minimal boost supported version to 1.61.0
- PR #3786 - Bump minimum required versions for 1.3.0 release
- PR #3785 - Explicitly set number of jobs for all ninja invocations on CircleCI
- PR #3784 - Fix leak and address sanitizer problems
- PR #3783 - Disabled even more spots that should not execute is networking is disabled
- PR #3782 - Cherry-picked tuple and thread_init_data fixes from #3701
- PR #3781 - Fix generic context coroutines after lazy stack allocation changes
- PR #3780 - Rename hello world examples
- PR #3776 - Sort algorithms now use the supplied chunker to determine the required minimal chunk size
- PR #3775 - Disable Boost auto-linking
- PR #3774 - Tag and push stable builds
- PR #3773 - Enable migration of polymorphic components
- PR #3771 - Fix link to stackoverflow in documentation
- PR #3770 - Replacing constexpr if in brace-serialization code
- PR #3769 - Fix SIGSEGV handler
- PR #3768 - Adding flags to scheduler allowing to control thread stealing and idle back-off
- PR #3767 - Fix help formatting in hpxrun.py
- PR #3765 - Fix a couple of bugs in the thread test
- PR #3764 - Workaround for SFINAE regression in msvc14.2
- PR #3762 - Prevent MSVC from prematurely instantiating things
- PR #3761 - Update python scripts to work with python 3
- PR #3760 - Fix callable vtable for GCC4.9
- PR #3759 - Rename
PAGE_SIZE
toPAGE_SIZE_
because AppleClang - PR #3755 - Making sure locks are not held during suspension
- PR #3754 - Disable more code if networking is not available/not enabled
- PR #3752 - Move
util::format
implementation to source file - PR #3751 - Fixing problems with
lcos::barrier
and iostreams - PR #3750 - Change error message to take into account
use_guard_page
setting - PR #3749 - Fix lifetime problem in
run_as_hpx_thread
- PR #3748 - Fixed unusable behavior of the clang code analyzer.
- PR #3747 - Added
PMIX_RANK
to the defaults ofHPX_WITH_PARCELPORT_MPI_ENV
. - PR #3745 - Introduced
cache_aligned_data
andcache_line_data
helper structure - PR #3742 - Remove more unused functionality from util/logging
- PR #3740 - Fix includes in partitioned vector tests
- PR #3739 - More fixes to make sure that
std::flush
really flushes all output - PR #3737 - Fix potential shutdown problems
- PR #3736 - Fix
guided_pool_executor
after dataflow changes caused compilation fail - PR #3734 - Limiting executor
- PR #3732 - More constrained bound constructors
- PR #3730 - Attempt to fix deadlocks during component loading
- PR #3729 - Add latch member function
count_up
and reset, requested by hpxMP - PR #3728 - Send even empty buffers on
hpx::endl
andhpx::flush
- PR #3727 - Adding example demonstrating how to customize the memory management for a component
- PR #3726 - Adding support for passing command line options through the
HPX_COMMANDLINE_OPTIONS
environment variable - PR #3722 - Document known broken OpenMPI builds
- PR #3716 - Add barrier reset function, requested by hpxMP for reusing barrier
- PR #3715 - More work on functions and vtables
- PR #3714 - Generate single-page HTML, PDF, manpage from documentation
- PR #3713 - Updating default APEX version to 2.1.2
- PR #3712 - Update release procedure
- PR #3710 - Fix the C++11 build, after #3704
- PR #3709 - Move some component_registry functionality to source file
- PR #3708 - Ignore all locks while handling assertions
- PR #3707 - Remove obsolete hpx runtime executable
- PR #3705 - Fix and simplify
make_ready_future
overload sets - PR #3704 - Reduce use of binders
- PR #3703 - Ini
- PR #3702 - Fixing CUDA compiler errors
- PR #3700 - Added
barrier::increment
function to increase total number of thread - PR #3697 - One more attempt to fix migration…
- PR #3694 - Fixing component migration
- PR #3693 - Print thread state when getting disallowed value in set_thread_state
- PR #3692 - Only disable
constexpr
with clang-cuda, not nvcc+gcc - PR #3691 - Link with libsupc++ if needed for thread_local
- PR #3690 - Remove thousands separators in set_operations_3442 to comply with C++11
- PR #3688 - Decouple serialization from function vtables
- PR #3687 - Fix a couple of test failures
- PR #3686 - Make sure tests.unit.build are run after install on CircleCI
- PR #3685 - Revise quickstart CMakeLists.txt explanation
- PR #3684 - Provide concept emulation for Ranges-TS concepts
- PR #3683 - Ignore uninitialized chunks
- PR #3682 - Ignore unitialized chunks. Check proper indices.
- PR #3680 - Ignore unitialized chunks. Check proper range indices
- PR #3679 - Simplify basic action implementations
- PR #3678 - Making sure
HPX_HAVE_LIBATOMIC
is unset before checking - PR #3677 - Fix generated full version number to be usable in expressions
- PR #3674 - Reduce functional utilities call depth
- PR #3672 - Change new build system to use existing macros related to pseudo dependencies
- PR #3669 - Remove indirection in
function_ref
when thread description is disabled - PR #3668 - Unbreaking
async_*cb*
tests - PR #3667 - Generate version.hpp
- PR #3665 - Enabling MPI parcelport for gitlab runners
- PR #3664 - making clang-tidy work properly again
- PR #3662 - Attempt to fix exception handling
- PR #3661 - Move
lcos::latch
to source file - PR #3660 - Fix accidentally explicit gid_type default constructor
- PR #3659 - Parallel executor latch
- PR #3658 - Fixing execution_parameters
- PR #3657 - Avoid dangling references in wait_all
- PR #3656 - Avoiding lifetime problems with sync_put_parcel
- PR #3655 - Fixing nullptr dereference inside of function
- PR #3652 - Attempt to fix
thread_map_type
definition with C++11 - PR #3650 - Allowing for end iterator being different from begin iterator
- PR #3649 - Added architecture identification to cmake to be able to detect timestamp support
- PR #3645 - Enabling sanitizers on gitlab runner
- PR #3644 - Attempt to tackle timeouts during startup
- PR #3642 - Cleanup parallel partitioners
- PR #3640 - Dataflow now works with functions that return a reference
- PR #3637 - Merging the executor-enabled overloads of
shared_future<>::then
- PR #3633 - Replace deprecated boost endian macros
- PR #3632 - Add instructions on getting HPX to documentation
- PR #3631 - Simplify parcel creation
- PR #3630 - Small additions and fixes to release procedure
- PR #3629 - Modular pp
- PR #3627 - Implement
util::function_ref
- PR #3626 - Fix cancelable_action_client example
- PR #3625 - Added automatic serialization for simple structs (see #3034)
- PR #3624 - Updating the default order of priority for
thread_description
- PR #3621 - Update copyright year and other small formatting fixes
- PR #3620 - Adding support for gitlab runner
- PR #3619 - Store debug logs and core dumps on CircleCI
- PR #3618 - Various optimizations
- PR #3617 - Fix link to the gpg key (#2)
- PR #3615 - Fix unused variable warnings with networking off
- PR #3614 - Restructuring counter data in scheduler to reduce false sharing
- PR #3613 - Adding support for gitlab runners
- PR #3610 - Don’t wait for
stop_condition
in main thread - PR #3608 - Add inline keyword to
invalid_thread_id
definition for nvcc - PR #3607 - Adding configuration key that allows to explicitly add a directory to the component search path
- PR #3606 - Add nvcc to exclude constexpress since is it not supported by nvcc
- PR #3605 - Add
inline
to definition of checkpoint stream operators to fix link error - PR #3604 - Use format for string formatting
- PR #3603 - Improve the error message for using to less
MAX_CPU_COUNT
- PR #3602 - Improve the error message for to small values of
MAX_CPU_COUNT
- PR #3600 - Parallel executor aggregated
- PR #3599 - Making sure networking is disabled for default one-locality-runs
- PR #3596 - Store thread exit functions in
forward_list
instead ofdeque
to avoid allocations - PR #3590 - Fix typo/mistake in thread queue
cleanup_terminated
- PR #3588 - Fix formatting errors in launching_and_configuring_hpx_applications.rst
- PR #3586 - Make bind propagate value category
- PR #3585 - Extend Cmake for building hpx as distribution packages (refs #3575)
- PR #3584 - Untangle function storage from object pointer
- PR #3582 - Towards Modularized HPX
- PR #3580 - Remove extra
||
in merge.hpp - PR #3577 - Partially revert “Remove vtable empty flag”
- PR #3576 - Make sure empty startup/shutdown functions are not being used
- PR #3574 - Make sure
DATAPAR
settings are conveyed to depending projects - PR #3573 - Make sure HPX is usable with latest released version of Vc (V1.4.1)
- PR #3572 - Adding test ensuring ticket 3565 is fixed
- PR #3571 - Make empty
[unique_]function
vtable non-dependent - PR #3566 - Fix compilation with dynamic bitset for CPU masks
- PR #3563 - Drop
util::[unique_]function
target_type - PR #3562 - Removing the target suffixes
- PR #3561 - Replace executor traits return type deduction (keep non-SFINAE)
- PR #3557 - Replace the last usages of boost::atomic
- PR #3556 - Replace
boost::scoped_array
withstd::unique_ptr
- PR #3552 - (Re)move APEX readme
- PR #3548 - Replace
boost::scoped_ptr
withstd::unique_ptr
- PR #3547 - Remove last use of Boost.Signals2
- PR #3544 - Post 1.2.0 version bumps
- PR #3543 - added Ubuntu dependency list to readme
- PR #3531 - Warnings, warnings…
- PR #3527 - Add CircleCI filter for building all tags
- PR #3525 - Segmented algorithms
- PR #3517 - Replace
boost::regex
with C++11<regex>
- PR #3514 - Cleaning up the build system
- PR #3505 - Fixing type attribute warning for
transfer_action
- PR #3504 - Add support for rpm packaging
- PR #3499 - Improving spinlock pools
- PR #3498 - Remove thread specific ptr
- PR #3486 - Fix comparison for expect_connecting_localities config entry
- PR #3469 - Enable (existing) code for extracting stack pointer on Power platform
HPX V1.2.1 (Feb 19, 2019)¶
General changes¶
This is a bugfix release. It contains the following changes:
- Fix compilation on ARM, s390x and 32-bit architectures.
- Fix a critical bug in the
future
implementation. - Fix several problems in the CMake configuration which affects external projects.
- Add support for Boost 1.69.0.
Closed issues¶
- Issue #3638 - Build HPX 1.2 with boost 1.69
- Issue #3635 - Non-deterministic crashing on Stampede2
- Issue #3550 - 1>e:000workhpxsrcthrow_exception.cpp(54): error C2440: ‘<function-style-cast>’: cannot convert from ‘boost::system::error_code’ to ‘hpx::exception’
- Issue #3549 - HPX 1.2.0 does not build on i686, but release candidate did
- Issue #3511 - Build on s390x fails
- Issue #3509 - Build on armv7l fails
Closed pull requests¶
- PR #3695 - Don’t install CMake templates and packaging files
- PR #3666 - Fixing yet another race in future_data
- PR #3663 - Fixing race between setting and getting the value inside future_data
- PR #3648 - Adding timestamp option for S390x platform
- PR #3647 - Blind attempt to fix warnings issued by gcc V9
- PR #3611 - Include GNUInstallDirs earlier to have it available for subdirectories
- PR #3595 - Use GNUInstallDirs lib path in pkgconfig config file
- PR #3593 - Add include(GNUInstallDirs) to HPXMacros.cmake
- PR #3591 - Fix compilation error on arm7 architecture. Compiles and runs on Fedora 29 on Pi 3.
- PR #3558 - Adding constructor exception(boost::system::error_code const&)
- PR #3555 - cmake: make install locations configurable
- PR #3551 - Fix uint64_t causing compilation fail on i686
HPX V1.2.0 (Nov 12, 2018)¶
General changes¶
Here are some of the main highlights and changes for this release:
- Thanks to the work of our Google Summer of Code student, Nikunj Gupta, we now
have a new implementation of
hpx_main.hpp
on supported platforms (Linux, BSD and MacOS). This is intended to be a less fragile drop-in replacement for the old implementation relying on preprocessor macros. The new implementation does not require changes if you are using the CMake or pkg-config. The old behaviour can be restored by settingHPX_WITH_DYNAMIC_HPX_MAIN=OFF
during CMake configuration. The implementation on Windows is unchanged. - We have added functionality to allow passing scheduling hints to our schedulers. These will allow us to create executors that for example target a specific NUMA domain or allow for HPX threads to be pinned to a particular worker thread.
- We have significantly improved the performance of our futures implementation by making the shared state atomic.
- We have replaced Boostbook by Sphinx for our documentation. This means the documentation is easier to navigate with built-in search and table of contents. We have also added a quick start section and restructured the documentation to be easier to follow for new users.
- We have added a new option to the
--hpx:threads
command line option. It is now possible to usecores
to tell HPX to only use one worker thread per core, unlike the existing optionall
which uses one worker thread per processing unit (processing unit can be a hyperthread if hyperthreads are available). The default value of--hpx:threads
has also been changed tocores
as this leads to better performance in most cases. - All command line options can now be passed alongside configuration options when initializing HPX. This means that some options that were previously only available on the command line can now be set as configuration options.
- HPXMP is a portable, scalable, and flexible application programming interface
using the OpenMP specification that supports multi-platform shared memory
multiprocessing programming in C and C++. HPXMP can be enabled within HPX by
setting
DHPX_WITH_HPXMP=ON
during CMake configuration. - Two new performance counters were added for measuring the time spent doing
background work.
/threads/time/background-work-duration
returns the time spent doing background on a given thread or locality, while/threads/time/background-overhead
returns the fraction of time spent doing background work with respect to the overall time spent running the scheduler. The new performance counters are disabled by default and can be turned on by settingHPX_WITH_BACKGROUND_THREAD_COUNTERS=ON
during CMake configuration. - The idling behaviour of HPX has been tweaked to allow for faster idling.
This is useful in interactive applications where the HPX worker threads may
not have work all the time. This behaviour can be tweaked and turned off as
before with
HPX_WITH_THREAD_MANAGER_IDLE_BACKOFF=OFF
during CMake configuration. - It is now possible to register callback functions for HPX worker thread events. Callbacks can be registered for starting and stopping worker threads, and for when errors occur.
Breaking changes¶
- The implementation of
hpx_main.hpp
has changed. If you are using custom Makefiles you will need to make changes. Please see the documentation on using Makefiles for more details. - The default value of
--hpx:threads
has changed fromall
tocores
. The new optioncores
only starts one worker thread per core. - We have dropped support for Boost 1.56 and 1.57. The minimal version of Boost we now test is 1.58.
- Our
boost::format
-based formatting implementation has been revised and replaced with a custom implementation. This changes the formatting syntax and requires changes if you are relying onhpx::util::format
orhpx::util::format_to
. The pull request for this change contains more information: PR #3266. - The following deprecated options have now been completely removed:
HPX_WITH_ASYNC_FUNCTION_COMPATIBILITY
,HPX_WITH_LOCAL_DATAFLOW
,HPX_WITH_GENERIC_EXECUTION_POLICY
,HPX_WITH_BOOST_CHRONO_COMPATIBILITY
,HPX_WITH_EXECUTOR_COMPATIBILITY
,HPX_WITH_EXECUTION_POLICY_COMPATIBILITY
, andHPX_WITH_TRANSFORM_REDUCE_COMPATIBILITY
.
Closed issues¶
- Issue #3538 - numa handling incorrect for hwloc 2
- Issue #3533 - Cmake version 3.5.1does not work (git ff26b35 2018-11-06)
- Issue #3526 - Failed building hpx-1.2.0-rc1 on Ubuntu16.04 x86-64 Virtualbox VM
- Issue #3512 - Build on aarch64 fails
- Issue #3475 - HPX fails to link if the MPI parcelport is enabled
- Issue #3462 - CMake configuration shows a minor and inconsequential failure to create a symlink
- Issue #3461 - Compilation Problems with the most recent Clang
- Issue #3460 - Deadlock when create_partitioner fails (assertion fails) in debug mode
- Issue #3455 - HPX build failing with HWLOC errors on POWER8 with hwloc 1.8
- Issue #3438 - HPX no longer builds on IBM POWER8
- Issue #3426 - hpx build failed on MacOS
- Issue #3424 - CircleCI builds broken for forked repositories
- Issue #3422 - Benchmarks in tests.performance.local are not run nightly
- Issue #3408 - CMake Targets for HPX
- Issue #3399 - processing unit out of bounds
- Issue #3395 - Floating point bug in hpx/runtime/threads/policies/scheduler_base.hpp
- Issue #3378 - compile error with lcos::communicator
- Issue #3376 - Failed to build HPX with APEX using clang
- Issue #3366 - Adapted Safe_Object example fails for –hpx:threads > 1
- Issue #3360 - Segmentation fault when passing component id as parameter
- Issue #3358 - HPX runtime hangs after multiple (~thousands) start-stop sequences
- Issue #3352 - Support TCP provider in libfabric ParcelPort
- Issue #3342 - undefined reference to __atomic_load_16
- Issue #3339 - setting command line options/flags from init cfg is not obvious
- Issue #3325 - AGAS migrates components prematurely
- Issue #3321 - hpx bad_parameter handling is awful
- Issue #3318 - Benchmarks fail to build with C++11
- Issue #3304 - hpx::threads::run_as_hpx_thread does not properly handle exceptions
- Issue #3300 - Setting pu step or offset results in no threads in default pool
- Issue #3297 - Crash with APEX when running Phylanx lra_csv with > 1 thread
- Issue #3296 - Building HPX with APEX configuration gives compiler warnings
- Issue #3290 - make tests failing at hello_world_component
- Issue #3285 - possible compilation error when “using namespace std;” is defined before including “hpx” headers files
- Issue #3280 - HPX fails on OSX
- Issue #3272 - CircleCI does not upload generated docker image any more
- Issue #3270 - Error when compiling CUDA examples
- Issue #3267 -
tests.unit.host_.block_allocator
fails occasionally - Issue #3264 - Possible move to Sphinx for documentation
- Issue #3263 - Documentation improvements
- Issue #3259 -
set_parcel_write_handler
test fails occasionally - Issue #3258 - Links to source code in documentation are broken
- Issue #3247 - Rare
tests.unit.host_.block_allocator
test failure on 1.1.0-rc1 - Issue #3244 - Slowing down and speeding up an interval_timer
- Issue #3215 - Cannot build both tests and examples on MSVC with pseudo-dependencies enabled
- Issue #3195 - Unnecessary customization point route causing performance penalty
- Issue #3088 - A strange thing in parallel::sort.
- Issue #2650 - libfabric support for passive endpoints
- Issue #1205 - TSS is broken
Closed pull requests¶
- PR #3542 - Fix numa lookup from pu when using hwloc 2.x
- PR #3541 - Fixing the build system of the MPI parcelport
- PR #3540 - Updating HPX people section
- PR #3539 - Splitting test to avoid OOM on CircleCI
- PR #3537 - Fix guided exec
- PR #3536 - Updating grants which support the LSU team
- PR #3535 - Fix hiding of docker credentials
- PR #3534 - Fixing #3533
- PR #3532 - fixing minor doc typo –hpx:print-counter-at arg
- PR #3530 - Changing APEX default tag to v2.1.0
- PR #3529 - Remove leftover security options and documentation
- PR #3528 - Fix hwloc version check
- PR #3524 - Do not build guided pool examples with older GCC compilers
- PR #3523 - Fix logging regression
- PR #3522 - Fix more warnings
- PR #3521 - Fixing argument handling in induction and reduction clauses for parallel::for_loop
- PR #3520 - Remove docs symlink and versioned docs folders
- PR #3519 - hpxMP release
- PR #3518 - Change all steps to use new docker image on CircleCI
- PR #3516 - Drop usage of deprecated facilities removed in C++17
- PR #3515 - Remove remaining uses of Boost.TypeTraits
- PR #3513 - Fixing a CMake problem when trying to use libfabric
- PR #3508 - Remove memory_block component
- PR #3507 - Propagating the MPI compile definitions to all relevant targets
- PR #3503 - Update documentation colors and logo
- PR #3502 - Fix bogus `throws` bindings in scheduled_thread_pool_impl
- PR #3501 - Split parallel::remove_if tests to avoid OOM on CircleCI
- PR #3500 - Support NONAMEPREFIX in add_hpx_library()
- PR #3497 - Note that cuda support requires cmake 3.9
- PR #3495 - Fixing dataflow
- PR #3493 - Remove deprecated options for 1.2.0 part 2
- PR #3492 - Add CUDA_LINK_LIBRARIES_KEYWORD to allow PRIVATE keyword in linkage t…
- PR #3491 - Changing Base docker image
- PR #3490 - Don’t create tasks immediately with hpx::apply
- PR #3489 - Remove deprecated options for 1.2.0
- PR #3488 - Revert “Use BUILD_INTERFACE generator expression to fix cmake flag exports”
- PR #3487 - Revert “Fixing type attribute warning for transfer_action”
- PR #3485 - Use BUILD_INTERFACE generator expression to fix cmake flag exports
- PR #3483 - Fixing type attribute warning for transfer_action
- PR #3481 - Remove unused variables
- PR #3480 - Towards a more lightweigh transfer action
- PR #3479 - Fix FLAGS - Use correct version of target_compile_options
- PR #3478 - Making sure the application’s exit code is properly propagated back to the OS
- PR #3476 - Don’t print docker credentials as part of the environment.
- PR #3473 - Fixing invalid cmake code if no jemalloc prefix was given
- PR #3472 - Attempting to work around recent clang test compilation failures
- PR #3471 - Enable jemalloc on windows
- PR #3470 - Updates readme
- PR #3468 - Avoid hang if there is an exception thrown during startup
- PR #3467 - Add compiler specific fallthrough attributes if C++17 attribute is not available
- PR #3466 - - bugfix : fix compilation with llvm-7.0
- PR #3465 - This patch adds various optimizations extracted from the thread_local_allocator work
- PR #3464 - Check for forked repos in CircleCI docker push step
- PR #3463 - - cmake : create the parent directory before symlinking
- PR #3459 - Remove unused/incomplete functionality from util/logging
- PR #3458 - Fix a problem with scope of CMAKE_CXX_FLAGS and hpx_add_compile_flag
- PR #3457 - Fixing more size_t -> int16_t (and similar) warnings
- PR #3456 - Add #ifdefs to topology.cpp to support old hwloc versions again
- PR #3454 - Fixing warnings related to silent conversion of size_t –> int16_t
- PR #3451 - Add examples as unit tests
- PR #3450 - Constexpr-fying bind and other functional facilities
- PR #3446 - Fix some thread suspension timeouts
- PR #3445 - Fix various warnings
- PR #3443 - Only enable service pool config options if pools are enabled
- PR #3441 - Fix missing closing brackets in documentation
- PR #3439 - Use correct MPI CXX libraries for MPI parcelport
- PR #3436 - Add projection function to find_* (and fix very bad bug)
- PR #3435 - Fixing 1205
- PR #3434 - Fix threads cores
- PR #3433 - Add Heise Online to release announcement list
- PR #3432 - Don’t track task dependencies for distributed runs
- PR #3431 - Circle CI setting changes for hpxMP
- PR #3430 - Fix unused params warning
- PR #3429 - One thread per core
- PR #3428 - This suppresses a deprecation warning that is being issued by MSVC 19.15.26726
- PR #3427 - Fixes #3426
- PR #3425 - Use source cache and workspace between job steps on CircleCI
- PR #3421 - Add CDash timing output to future overhead test (for graphs)
- PR #3420 - Add guided_pool_executor
- PR #3419 - Fix typo in CircleCI config
- PR #3418 - Add sphinx documentation
- PR #3415 - Scheduler NUMA hint and shared priority scheduler
- PR #3414 - Adding step to synchronize the APEX release
- PR #3413 - Fixing multiple defines of APEX_HAVE_HPX
- PR #3412 - Fixes linking with libhpx_wrap error with BSD and Windows based systems
- PR #3410 - Fix typo in CMakeLists.txt
- PR #3409 - Fix brackets and indentation in existing_performance_counters.qbk
- PR #3407 - Fix unused param and extra ; warnings emitted by gcc 8.x
- PR #3406 - Adding thread local allocator and use it for future shared states
- PR #3405 - Adding DHPX_HAVE_THREAD_LOCAL_STORAGE=ON to builds
- PR #3404 - fixing multiple difinition of main() in linux
- PR #3402 - Allow debug option to be enabled only for Linux systems with dynamic main on
- PR #3401 - Fix cuda_future_helper.h when compiling with C++11
- PR #3400 - Fix floating point exception scheduler_base idle backoff
- PR #3398 - Atomic future state
- PR #3397 - Fixing code for older gcc versions
- PR #3396 - Allowing to register thread event functions (start/stop/error)
- PR #3394 - Fix small mistake in primary_namespace_server.cpp
- PR #3393 - Explicitly instantiate configured schedulers
- PR #3392 - Add performance counters background overhead and background work duration
- PR #3391 - Adapt integration of HPXMP to latest build system changes
- PR #3390 - Make AGAS measurements optional
- PR #3389 - Fix deadlock during shutdown
- PR #3388 - Add several functionalities allowing to optimize synchronous action invocation
- PR #3387 - Add cmake option to opt out of fail-compile tests
- PR #3386 - Adding support for boost::container::small_vector to dataflow
- PR #3385 - Adds Debug option for hpx initializing from main
- PR #3384 - This hopefully fixes two tests that occasionally fail
- PR #3383 - Making sure thread local storage is enable for hpxMP
- PR #3382 - Fix usage of HPX_CAPTURE together with default value capture [=]
- PR #3381 - Replace undefined instantiations of uniform_int_distribution
- PR #3380 - Add missing semicolons to uses of HPX_COMPILER_FENCE
- PR #3379 - Fixing #3378
- PR #3377 - Adding build system support to integrate hpxmp into hpx at the user’s machine
- PR #3375 - Replacing wrapper for __libc_start_main with main
- PR #3374 - Adds hpx_wrap to HPX_LINK_LIBRARIES which links only when specified.
- PR #3373 - Forcing cache settings in HPXConfig.cmake to guarantee updated values
- PR #3372 - Fix some more c++11 build problems
- PR #3371 - Adds HPX_LINKER_FLAGS to HPX applications without editing their source codes
- PR #3370 - util::format: add type_specifier<> specializations for %!s(MISSING) and %!l(MISSING)s
- PR #3369 - Adding configuration option to allow explicit disable of the new hpx_main feature on Linux
- PR #3368 - Updates doc with recent hpx_wrap implementation
- PR #3367 - Adds Mac OS implementation to hpx_main.hpp
- PR #3365 - Fix order of hpx libs in HPX_CONF_LIBRARIES.
- PR #3363 - Apex fixing null wrapper
- PR #3361 - Making sure all parcels get destroyed on an HPX thread (TCP pp)
- PR #3359 - Feature/improveerrorforcompiler
- PR #3357 - Static/dynamic executable implementation
- PR #3355 - Reverting changes introduced by #3283 as those make applications hang
- PR #3354 - Add external dependencies to HPX_LIBRARY_DIR
- PR #3353 - Fix libfabric tcp
- PR #3351 - Move obsolete header to tests directory.
- PR #3350 - Renaming two functions to avoid problem described in #3285
- PR #3349 - Make idle backoff exponential with maximum sleep time
- PR #3347 - Replace simple_component* with component* in the Documentation
- PR #3346 - Fix CMakeLists.txt example in quick start
- PR #3345 - Fix automatic setting of HPX_MORE_THAN_64_THREADS
- PR #3344 - Reduce amount of information printed for unknown command line options
- PR #3343 - Safeguard HPX against destruction in global contexts
- PR #3341 - Allowing for all command line options to be used as configuration settings
- PR #3340 - Always convert inspect results to JUnit XML
- PR #3336 - Only run docker push on master on CircleCI
- PR #3335 - Update description of hpx.os_threads config parameter.
- PR #3334 - Making sure early logging settings don’t get mixed with others
- PR #3333 - Update CMake links and versions in documentation
- PR #3332 - Add notes on target suffixes to CMake documentation
- PR #3331 - Add quickstart section to documentation
- PR #3330 - Rename resource_partitioner test to avoid conflicts with pseudodependencies
- PR #3328 - Making sure object is pinned while executing actions, even if action returns a future
- PR #3327 - Add missing std::forward to tuple.hpp
- PR #3326 - Make sure logging is up and running while modules are being discovered.
- PR #3324 - Replace C++14 overload of std::equal with C++11 code.
- PR #3323 - Fix a missing apex thread data (wrapper) initialization
- PR #3320 - Adding support for -std=c++2a (define HPX_WITH_CXX2A=On)
- PR #3319 - Replacing C++14 feature with equivalent C++11 code
- PR #3317 - Fix compilation with VS 15.7.1 and /std:c++latest
- PR #3316 - Fix includes for 1d_stencil_*_omp examples
- PR #3314 - Remove some unused parameter warnings
- PR #3313 - Fix pu-step and pu-offset command line options
- PR #3312 - Add conversion of inspect reports to JUnit XML
- PR #3311 - Fix escaping of closing braces in format specification syntax
- PR #3310 - Don’t overwrite user settings with defaults in registration database
- PR #3309 - Fixing potential stack overflow for dataflow
- PR #3308 - This updates the .clang-format configuration file to utilize newer features
- PR #3306 - Marking migratable objects in their gid to allow not handling migration in AGAS
- PR #3305 - Add proper exception handling to run_as_hpx_thread
- PR #3303 - Changed std::rand to a better inbuilt PRNG Generator
- PR #3302 - All non-migratable (simple) components now encode their lva and component type in their gid
- PR #3301 - Add nullptr_t overloads to resource partitioner
- PR #3298 - Apex task wrapper memory bug
- PR #3295 - Fix mistakes after merge of CircleCI config
- PR #3294 - Fix partitioned vector include in partitioned_vector_find tests
- PR #3293 - Adding emplace support to promise and make_ready_future
- PR #3292 - Add new cuda kernel synchronization with hpx::future demo
- PR #3291 - Fixes #3290
- PR #3289 - Fixing Docker image creation
- PR #3288 - Avoid allocating shared state for wait_all
- PR #3287 - Fixing /scheduler/utilization/instantaneous performance counter
- PR #3286 - dataflow() and future::then() use sync policy where possible
- PR #3284 - Background thread can use relaxed atomics to manipulate thread state
- PR #3283 - Do not unwrap ready future
- PR #3282 - Fix virtual method override warnings in static schedulers
- PR #3281 - Disable set_area_membind_nodeset for OSX
- PR #3279 - Add two variations to the future_overhead benchmark
- PR #3278 - Fix circleci workspace
- PR #3277 - Support external plugins
- PR #3276 - Fix missing parenthesis in hello_compute.cu.
- PR #3274 - Reinit counters synchronously in reinit_counters test
- PR #3273 - Splitting tests to avoid compiler OOM
- PR #3271 - Remove leftover code from context_generic_context.hpp
- PR #3269 - Fix bulk_construct with count = 0
- PR #3268 - Replace constexpr with HPX_CXX14_CONSTEXPR and HPX_CONSTEXPR
- PR #3266 - Replace boost::format with custom sprintf-based implementation
- PR #3265 - Split parallel tests on CircleCI
- PR #3262 - Making sure documentation correctly links to source files
- PR #3261 - Apex refactoring fix rebind
- PR #3260 - Isolate performance counter parser into a separate TU
- PR #3256 - Post 1.1.0 version bumps
- PR #3254 - Adding trait for actions allowing to make runtime decision on whether to execute it directly
- PR #3253 - Bump minimal supported Boost to 1.58.0
- PR #3251 - Adds new feature: changing interval used in interval_timer (issue 3244)
- PR #3239 - Changing std::rand() to a better inbuilt PRNG generator.
- PR #3234 - Disable background thread when networking is off
- PR #3232 - Clean up suspension tests
- PR #3230 - Add optional scheduler mode parameter to create_thread_pool function
- PR #3228 - Allow suspension also on static schedulers
- PR #3163 - libfabric parcelport w/o HPX_PARCELPORT_LIBFABRIC_ENDPOINT_RDM
- PR #3036 - Switching to CircleCI 2.0
HPX V1.1.0 (Mar 24, 2018)¶
General changes¶
Here are some of the main highlights and changes for this release (in no particular order):
- We have changed the way HPX manages the processing units on a node. We do
not longer implicitly bind all available cores to a single thread pool. The
user has now full control over what processing units are bound to what thread
pool, each with a separate scheduler. It is now also possible to create your
own scheduler implementation and control what processing units this scheduler
should use. We added the
hpx::resource::partitioner
that manages all available processing units and assigns resources to the used thread pools. Thread pools can be now be suspended/resumed independently. This functionality helps in running HPX concurrently to code that is directly relying on OpenMP and/or MPI. - We have continued to implement various parallel algorithms. HPX now almost
completely implements all of the parallel algorithms as specified by the
C++17 Standard. We have also continued to implement these algorithms for the
distributed use case (for segmented data structures, such as
hpx::partitioned_vector
). - Added a compatibility layer for
std::thread
,std::mutex
, andstd::condition_variable
allowing for the code to use those facilities where available and to fall back to the corresponding Boost facilities otherwise. The CMake configuration option-DHPX_WITH_THREAD_COMPATIBILITY=On
can be used to force using the Boost equivalents. - The parameter sequence for the
hpx::parallel::transform_inclusive_scan
overload taking one iterator range has changed (again) to match the changes this algorithm has undergone while being moved to C++17. The old overloads can be still enabled at configure time by passing-DHPX_WITH_TRANSFORM_REDUCE_COMPATIBILITY=On
to CMake. - The parameter sequence for the
hpx::parallel::inclusive_scan
overload taking one iterator range has changed to match the changes this algorithm has undergone while being moved to C++17. The old overloads can be still enabled at configure time by passing-DHPX_WITH_INCLUSIVE_SCAN_COMPATIBILITY=On
to CMake. - Added a helper facility
hpx::local_new
which is equivalent tohpx::new_
except that it creates components locally only. As a consequence, the used component constructor may accept non-serializable argument types and/or non-const references or pointers. - Removed the (broken) component type
hpx::lcos::queue<T>
. The old type is still available at configure time by passing-DHPX_WITH_QUEUE_COMPATIBILITY=On
to CMake. - The parallel algorithms adopted for C++17 restrict the iterator categories
usable with those to at least forward iterators. Our implementation of the
parallel algorithms was supporting input iterators (and output iterators) as
well by simply falling back to sequential execution. We have now made our
implementations conforming by requiring at least forward iterators. In order
to enable the old behavior use the the compatibility option
-DHPX_WITH_ALGORITHM_INPUT_ITERATOR_SUPPORT=On
on the CMake command line. - We have added the functionalities allowing for LCOs being implemented using (simple) components. Before LCOs had to always be implemented using managed components.
- User defined components don’t have to be default-constructible anymore. Return types from actions don’t have to be default-constructible anymore either. Our serialization layer now in general supports non-default-constructible types.
- We have added a new launch policy
hpx::launch::lazy
that allows to defer the decision on what launch policy to use to the point of execution. This policy is initialized with a function (object) that – when invoked – is expected to produce the desired launch policy.
Breaking changes¶
- We have dropped support for the gcc compiler version V4.8. The minimal gcc version we now test on is gcc V4.9. The minimally required version of CMake is now V3.3.2.
- We have dropped support for the Visual Studio 2013 compiler version. The minimal Visual Studio version we now test on is Visual Studio 2015.5.
- We have dropped support for the Boost V1.51-V1.54. The minimal version of Boost we now test is Boost V1.55.
- We have dropped support for the
hpx::util::unwrapped
API.hpx::util::unwrapped
will stay functional to some degree, until it finally gets removed in a later version of HPX. The functional usage ofhpx::util::unwrapped
should be changed to the newhpx::util::unwrapping
function whereas the immediate usage should be replaced tohpx::util::unwrap
. - The performance counter names referring to properties as exposed by the threading subsystem have changes as those now additionally have to specify the thread-pool. See the corresponding documentation for more details.
- The overloads of
hpx::async
that invoke an action do not perform implicit unwrapping of the returned future anymore in case the invoked function does return a future in the first place. In this casehpx::async
now returns ahpx::future<future<T>>
making its behavior conforming to its local counterpart. - We have replaced the use of
boost::exception_ptr
in our APIs with the equivalentstd::exception_ptr
. Please change your codes accordingly. No compatibility settings are provided. - We have removed the compatibility settings for
HPX_WITH_COLOCATED_BACKWARDS_COMPATIBILITY
andHPX_WITH_COMPONENT_GET_GID_COMPATIBILITY
as their life-cycle has reached its end. - We have removed the experimental thread schedulers hierarchy_scheduler, periodic_priority_scheduler and throttling_scheduler in an effort to clean up and consolidate our thread schedulers.
Bug fixes (closed tickets)¶
Here is a list of the important tickets we closed for this release.
- PR #3250 - Apex refactoring with guids
- PR #3249 - Updating People.qbk
- PR #3246 - Assorted fixes for CUDA
- PR #3245 - Apex refactoring with guids
- PR #3242 - Modify task counting in thread_queue.hpp
- PR #3240 - Fixed typos
- PR #3238 - Readding accidently removed std::abort
- PR #3237 - Adding Pipeline example
- PR #3236 - Fixing memory_block
- PR #3233 - Make schedule_thread take suspended threads into account
- Issue #3226 - memory_block is breaking, signaling SIGSEGV on a thread on creation and freeing
- PR #3225 - Applying quick fix for hwloc-2.0
- Issue #3224 - HPX counters crashing the application
- PR #3223 - Fix returns when setting config entries
- Issue #3222 - Errors linking libhpx.so
- Issue #3221 - HPX on Mac OS X with HWLoc 2.0.0 fails to run
- PR #3216 - Reorder a variadic array to satisfy VS 2017 15.6
- PR #3214 - Changed prerequisites.qbk to avoid confusion while building boost
- PR #3213 - Relax locks for thread suspension to avoid holding locks when yielding
- PR #3212 - Fix check in sequenced_executor test
- PR #3211 - Use preinit_array to set argc/argv in init_globally example
- PR #3210 - Adapted parallel::{search | search_n} for Ranges TS (see #1668)
- PR #3209 - Fix locking problems during shutdown
- Issue #3208 - init_globally throwing a run-time error
- PR #3206 - Addition of new arithmetic performance counter “Count”
- PR #3205 - Fixing return type calculation for bulk_then_execute
- PR #3204 - Changing std::rand() to a better inbuilt PRNG generator
- PR #3203 - Resolving problems during shutdown for VS2015
- PR #3202 - Making sure resource partitioner is not accessed if its not valid
- PR #3201 - Fixing optional::swap
- Issue #3200 - hpx::util::optional fails
- PR #3199 - Fix sliding_semaphore test
- PR #3198 - Set pre_main status before launching run_helper
- PR #3197 - Update README.rst
- PR #3194 - parallel::{fill|fill_n} updated for Ranges TS
- PR #3193 - Updating Runtime.cpp by adding correct description of Performance counters during register
- PR #3191 - Fix sliding_semaphore_2338 test
- PR #3190 - Topology improvements
- PR #3189 - Deleting one include of median from BOOST library to arithmetics_counter file
- PR #3188 - Optionally disable printing of diagnostics during terminate
- PR #3187 - Suppressing cmake warning issued by cmake > V3.11
- PR #3185 - Remove unused scoped_unlock, unlock_guard_try
- PR #3184 - Fix nqueen example
- PR #3183 - Add runtime start/stop, resume/suspend and OpenMP benchmarks
- Issue #3182 - bulk_then_execute has unexpected return type/does not compile
- Issue #3181 - hwloc 2.0 breaks topo class and cannot be used
- Issue #3180 - Schedulers that don’t support suspend/resume are unusable
- PR #3179 - Various minor changes to support FLeCSI
- PR #3178 - Fix #3124
- PR #3177 - Removed allgather
- PR #3176 - Fixed Documentation for “using_hpx_pkgconfig”
- PR #3174 - Add hpx::iostreams::ostream overload to format_to
- PR #3172 - Fix lifo queue backend
- PR #3171 - adding the missing unset() function to cpu_mask() for case of more than 64 threads
- PR #3170 - Add cmake flag -DHPX_WITH_FAULT_TOLERANCE=ON (OFF by default)
- PR #3169 - Adapted parallel::{count|count_if} for Ranges TS (see #1668)
- PR #3168 - Changing used namespace for seq execution policy
- Issue #3167 - Update GSoC projects
- Issue #3166 - Application (Octotiger) gets stuck on hpx::finalize when only using one thread
- Issue #3165 - Compilation of parallel algorithms with HPX_WITH_DATAPAR is broken
- PR #3164 - Fixing component migration
- PR #3162 - regex_from_pattern: escape regex special characters to avoid misinterpretation
- Issue #3161 - Building HPX with hwloc 2.0.0 fails
- PR #3160 - Fixing the handling of quoted command line arguments.
- PR #3158 - Fixing a race with timed suspension (second attempt)
- PR #3157 - Revert “Fixing a race with timed suspension”
- PR #3156 - Fixing serialization of classes with incompatible serialize signature
- PR #3154 - More refactorings based on clang-tidy reports
- PR #3153 - Fixing a race with timed suspension
- PR #3152 - Documentation for runtime suspension
- PR #3151 - Use small_vector only from boost version 1.59 onwards
- PR #3150 - Avoiding more stack overflows
- PR #3148 - Refactoring component_base and base_action/transfer_base_action
- PR #3147 - Move yield_while out of detail namespace and into own file
- PR #3145 - Remove a leftover of the cxx11 std array cleanup
- PR #3144 - Minor changes to how actions are executed
- PR #3143 - Fix stack overhead
- PR #3142 - Fix typo in config.hpp
- PR #3141 - Fixing small_vector compatibility with older boost version
- PR #3140 - is_heap_text fix
- Issue #3139 - Error in is_heap_tests.hpp
- PR #3138 - Partially reverting #3126
- PR #3137 - Suspend speedup
- PR #3136 - Revert “Fixing #2325”
- PR #3135 - Improving destruction of threads
- Issue #3134 - HPX_SERIALIZATION_SPLIT_FREE does not stop compiler from looking for serialize() method
- PR #3133 - Make hwloc compulsory
- PR #3132 - Update CXX14 constexpr feature test
- PR #3131 - Fixing #2325
- PR #3130 - Avoid completion handler allocation
- PR #3129 - Suspend runtime
- PR #3128 - Make docbook dtd and xsl path names consistent
- PR #3127 - Add hpx::start nullptr overloads
- PR #3126 - Cleaning up coroutine implementation
- PR #3125 - Replacing nullptr with hpx::threads::invalid_thread_id
- Issue #3124 - Add hello_world_component to CI builds
- PR #3123 - Add new constructor.
- PR #3122 - Fixing #3121
- Issue #3121 - HPX_SMT_PAUSE is broken on non-x86 platforms when __GNUC__ is defined
- PR #3120 - Don’t use boost::intrusive_ptr for thread_id_type
- PR #3119 - Disable default executor compatibility with V1 executors
- PR #3118 - Adding performance_counter::reinit to allow for dynamically changing counter sets
- PR #3117 - Replace uses of boost/experimental::optional with util::optional
- PR #3116 - Moving background thread APEX timer #2980
- PR #3115 - Fixing race condition in channel test
- PR #3114 - Avoid using util::function for thread function wrappers
- PR #3113 - cmake V3.10.2 has changed the variable names used for MPI
- PR #3112 - Minor fixes to exclusive_scan algorithm
- PR #3111 - Revert “fix detection of cxx11_std_atomic”
- PR #3110 - Suspend thread pool
- PR #3109 - Fixing thread scheduling when yielding a thread id
- PR #3108 - Revert “Suspend thread pool”
- PR #3107 - Remove UB from thread::id relational operators
- PR #3106 - Add cmake test for std::decay_t to fix cuda build
- PR #3105 - Fixing refcount for async traversal frame
- PR #3104 - Local execution of direct actions is now actually performed directly
- PR #3103 - Adding support for generic counter_raw_values performance counter type
- Issue #3102 - Introduce generic performance counter type returning an array of values
- PR #3101 - Revert “Adapting stack overhead limit for gcc 4.9”
- PR #3100 - Fix #3068 (condition_variable deadlock)
- PR #3099 - Fixing lock held during suspension in papi counter component
- PR #3098 - Unbreak broadcast_wait_for_2822 test
- PR #3097 - Adapting stack overhead limit for gcc 4.9
- PR #3096 - fix detection of cxx11_std_atomic
- PR #3095 - Add ciso646 header to get _LIBCPP_VERSION for testing inplace merge
- PR #3094 - Relax atomic operations on performance counter values
- PR #3093 - Short-circuit all_of/any_of/none_of instantiations
- PR #3092 - Take advantage of C++14 lambda capture initialization syntax, where possible
- PR #3091 - Remove more references to Boost from logging code
- PR #3090 - Unify use of yield/yield_k
- PR #3089 - Fix a strange thing in parallel::detail::handle_exception. (Fix #2834.)
- Issue #3088 - A strange thing in parallel::sort.
- PR #3087 - Fixing assertion in default_distribution_policy
- PR #3086 - Implement parallel::remove and parallel::remove_if
- PR #3085 - Addressing breaking changes in Boost V1.66
- PR #3084 - Ignore build warnings round 2
- PR #3083 - Fix typo HPX_WITH_MM_PREFECTH
- PR #3081 - Pre-decay template arguments early
- PR #3080 - Suspend thread pool
- PR #3079 - Ignore build warnings
- PR #3078 - Don’t test inplace_merge with libc++
- PR #3076 - Fixing 3075: Part 1
- PR #3074 - Fix more build warnings
- PR #3073 - Suspend thread cleanup
- PR #3072 - Change existing symbol_namespace::iterate to return all data instead of invoking a callback
- PR #3071 - Fixing pack_traversal_async test
- PR #3070 - Fix dynamic_counters_loaded_1508 test by adding dependency to memory_component
- PR #3069 - Fix scheduling loop exit
- Issue #3068 - hpx::lcos::condition_variable could be suspect to deadlocks
- PR #3067 - #ifdef out random_shuffle deprecated in later c++
- PR #3066 - Make coalescing test depend on coalescing library to ensure it gets built
- PR #3065 - Workaround for minimal_timed_async_executor_test compilation failures, attempts to copy a deferred call (in unevaluated context)
- PR #3064 - Fixing wrong condition in wrapper_heap
- PR #3062 - Fix exception handling for execution::seq
- PR #3061 - Adapt MSVC C++ mode handling to VS15.5
- PR #3060 - Fix compiler problem in MSVC release mode
- PR #3059 - Fixing #2931
- Issue #3058 - minimal_timed_async_executor_test_exe fails to compile on master (d6f505c)
- PR #3057 - Fix stable_merge_2964 compilation problems
- PR #3056 - Fix some build warnings caused by unused variables/unnecessary tests
- PR #3055 - Update documentation for running tests
- Issue #3054 - Assertion failure when using bulk hpx::new_ in asynchronous mode
- PR #3052 - Do not bind test running to cmake test build rule
- PR #3051 - Fix HPX-Qt interaction in Qt example.
- Issue #3048 - nqueen example fails occasionally
- PR #3047 - Fixing #3044
- PR #3046 - Add OS thread suspension
- PR #3042 - PyCicle - first attempt at a build toold for checking PR’s
- PR #3041 - Fix a problem about asynchronous execution of parallel::merge and parallel::partition.
- PR #3040 - Fix a mistake about exception handling in asynchronous execution of scan_partitioner.
- PR #3039 - Consistently use executors to schedule work
- PR #3038 - Fixing local direct function execution and lambda actions perfect forwarding
- PR #3035 - Make parallel unit test names match build target/folder names
- PR #3033 - Fix setting of default build type
- Issue #3032 - Fix partitioner arg copy found in #2982
- Issue #3031 - Errors linking libhpx.so due to missing references (master branch, commit 6679a8882)
- PR #3030 - Revert “implement executor then interface with && forwarding reference”
- PR #3029 - Run CI inspect checks before building
- PR #3028 - Added range version of parallel::move
- Issue #3027 - Implement all scheduling APIs in terms of executors
- PR #3026 - implement executor then interface with && forwarding reference
- PR #3025 - Fix typo unitialized to uninitialized
- PR #3024 - Inspect fixes
- PR #3023 - P0356 Simplified partial function application
- PR #3022 - Master fixes
- PR #3021 - Segfault fix
- PR #3020 - Disable command-line aliasing for applications that use user_main
- PR #3019 - Adding enable_elasticity option to pool configuration
- PR #3018 - Fix stack overflow detection configuration in header files
- PR #3017 - Speed up local action execution
- PR #3016 - Unify stack-overflow detection options, remove reference to libsigsegv
- PR #3015 - Speeding up accessing the resource partitioner and the topology info
- Issue #3014 - HPX does not compile on POWER8 with gcc 5.4
- Issue #3013 - hello_world occasionally prints multiple lines from a single OS-thread
- PR #3012 - Silence warning about casting away qualifiers in itt_notify.hpp
- PR #3011 - Fix cpuset leak in hwloc_topology_info.cpp
- PR #3010 - Remove useless decay_copy
- PR #3009 - Fixing 2996
- PR #3008 - Remove unused internal function
- PR #3007 - Fixing wrapper_heap alignment problems
- Issue #3006 - hwloc memory leak
- PR #3004 - Silence C4251 (needs to have dll-interface) for future_data_void
- Issue #3003 - Suspension of runtime
- PR #3001 - Attempting to avoid data races in async_traversal while evaluating dataflow()
- PR #3000 - Adding hpx::util::optional as a first step to replace experimental::optional
- PR #2998 - Cleanup up and Fixing component creation and deletion
- Issue #2996 - Build fails with HPX_WITH_HWLOC=OFF
- PR #2995 - Push more future_data functionality to source file
- PR #2994 - WIP: Fix throttle test
- PR #2993 - Making sure –hpx:help does not throw for required (but missing) arguments
- PR #2992 - Adding non-blocking (on destruction) service executors
- Issue #2991 - run_as_os_thread locks up
- Issue #2990 - –help will not work until all required options are provided
- PR #2989 - Improve error messages caused by misuse of dataflow
- PR #2988 - Improve error messages caused by misuse of .then
- Issue #2987 - stack overflow detection producing false positives
- PR #2986 - Deduplicate non-dependent thread_info logging types
- PR #2985 - Adapted parallel::{all_of|any_of|none_of} for Ranges TS (see #1668)
- PR #2984 - Refactor one_size_heap code to simplify code
- PR #2983 - Fixing local_new_component
- PR #2982 - Clang tidy
- PR #2981 - Simplify allocator rebinding in pack traversal
- PR #2979 - Fixing integer overflows
- PR #2978 - Implement parallel::inplace_merge
- Issue #2977 - Make hwloc compulsory instead of optional
- PR #2976 - Making sure client_base instance that registered the component does not unregister it when being destructed
- PR #2975 - Change version of pulled APEX to master
- PR #2974 - Fix domain not being freed at the end of scheduling loop
- PR #2973 - Fix small typos
- PR #2972 - Adding uintstd.h header
- PR #2971 - Fall back to creating local components using local_new
- PR #2970 - Improve is_tuple_like trait
- PR #2969 - Fix HPX_WITH_MORE_THAN_64_THREADS default value
- PR #2968 - Cleaning up dataflow overload set
- PR #2967 - Make parallel::merge is stable. (Fix #2964.)
- PR #2966 - Fixing a couple of held locks during exception handling
- PR #2965 - Adding missing #include
- Issue #2964 - parallel merge is not stable
- PR #2963 - Making sure any function object passed to dataflow is released after being invoked
- PR #2962 - Partially reverting #2891
- PR #2961 - Attempt to fix the gcc 4.9 problem with the async pack traversal
- Issue #2959 - Program terminates during error handling
- Issue #2958 - HPX_PLAIN_ACTION breaks due to missing include
- PR #2957 - Fixing errors generated by mixing different attribute syntaxes
- Issue #2956 - Mixing attribute syntaxes leads to compiler errors
- Issue #2955 - Fix OS-Thread throttling
- PR #2953 - Making sure any hpx.os_threads=N supplied through a –hpx::config file is taken into account
- PR #2952 - Removing wrong call to cleanup_terminated_locked
- PR #2951 - Revert “Make sure the function vtables are initialized before use”
- PR #2950 - Fix a namespace compilation error when some schedulers are disabled
- Issue #2949 - master branch giving lockups on shutdown
- Issue #2947 - hpx.ini is not used correctly at initialization
- PR #2946 - Adding explicit feature test for thread_local
- PR #2945 - Make sure the function vtables are initialized before use
- PR #2944 - Attempting to solve affinity problems on CircleCI
- PR #2943 - Changing channel actions to be direct
- PR #2942 - Adding split_future for std::vector
- PR #2941 - Add a feature test to test for CXX11 override
- Issue #2940 - Add split_future for future<vector<T>>
- PR #2939 - Making error reporting during problems with setting affinity masks more verbose
- PR #2938 - Fix this various executors
- PR #2937 - Fix some typos in documentation
- PR #2934 - Remove the need for “complete” SFINAE checks
- PR #2933 - Making sure parallel::for_loop is executed in parallel if requested
- PR #2932 - Classify chunk_size_iterator to input iterator tag. (Fix #2866)
- Issue #2931 - –hpx:help triggers unusual error with clang build
- PR #2930 - Add #include files needed to set _POSIX_VERSION for debug check
- PR #2929 - Fix a couple of deprecated c++ features
- PR #2928 - Fixing execution parameters
- Issue #2927 - CMake warning: … cycle in constraint graph
- PR #2926 - Default pool rename
- Issue #2925 - Default pool cannot be renamed
- Issue #2924 - hpx:attach-debugger=startup does not work any more
- PR #2923 - Alloc membind
- PR #2922 - This fixes CircleCI errors when running with –hpx:bind=none
- PR #2921 - Custom pool executor was missing priority and stacksize options
- PR #2920 - Adding test to trigger problem reported in #2916
- PR #2919 - Make sure the resource_partitioner is properly destructed on hpx::finalize
- Issue #2918 - hpx::init calls wrong (first) callback when called multiple times
- PR #2917 - Adding util::checkpoint
- Issue #2916 - Weird runtime failures when using a channel and chained continuations
- PR #2915 - Introduce executor parameters customization points
- Issue #2914 - Task assignment to current Pool has unintended consequences
- PR #2913 - Fix rp hang
- PR #2912 - Update contributors
- PR #2911 - Fixing CUDA problems
- PR #2910 - Improve error reporting for process component on POSIX systems
- PR #2909 - Fix typo in include path
- PR #2908 - Use proper container according to iterator tag in benchmarks of parallel algorithms
- PR #2907 - Optionaly force-delete remaining channel items on close
- PR #2906 - Making sure generated performance counter names are correct
- Issue #2905 - collecting idle-rate performance counters on multiple localities produces an error
- Issue #2904 - build broken for Intel 17 compilers
- PR #2903 - Documentation Updates– Adding New People
- PR #2902 - Fixing service_executor
- PR #2901 - Fixing partitioned_vector creation
- PR #2900 - Add numa-balanced mode to hpx::bind, spread cores over numa domains
- Issue #2899 - hpx::bind does not have a mode that balances cores over numa domains
- PR #2898 - Adding missing #include and missing guard for optional code section
- PR #2897 - Removing dependency on Boost.ICL
- Issue #2896 - Debug build fails without -fpermissive with GCC 7.1 and Boost 1.65
- PR #2895 - Fixing SLURM environment parsing
- PR #2894 - Fix incorrect handling of compile definition with value 0
- Issue #2893 - Disabling schedulers causes build errors
- PR #2892 - added list serializer
- PR #2891 - Resource Partitioner Fixes
- Issue #2890 - Destroying a non-empty channel causes an assertion failure
- PR #2889 - Add check for libatomic
- PR #2888 - Fix compilation problems if HPX_WITH_ITT_NOTIFY=ON
- PR #2887 - Adapt broadcast() to non-unwrapping async<Action>
- PR #2886 - Replace Boost.Random with C++11 <random>
- Issue #2885 - regression in broadcast?
- Issue #2884 - linking
-latomic
is not portable - PR #2883 - Explicitly set -pthread flag if available
- PR #2882 - Wrap boost::format uses
- Issue #2881 - hpx not compiling with
HPX_WITH_ITTNOTIFY=On
- Issue #2880 - hpx::bind scatter/balanced give wrong pu masks
- PR #2878 - Fix incorrect pool usage masks setup in RP/thread manager
- PR #2877 - Require
std::array
by default - PR #2875 - Deprecate use of BOOST_ASSERT
- PR #2874 - Changed serialization of boost.variant to use variadic templates
- Issue #2873 - building with parcelport_mpi fails on cori
- PR #2871 - Adding missing support for throttling scheduler
- PR #2870 - Disambiguate use of base_lco_with_value macros with channel
- Issue #2869 - Difficulty compiling
HPX_REGISTER_CHANNEL_DECLARATION(double)
- PR #2868 - Removing uneeded assert
- PR #2867 - Implement parallel::unique
- Issue #2866 - The chunk_size_iterator violates multipass guarantee
- PR #2865 - Only use sched_getcpu on linux machines
- PR #2864 - Create redistribution archive for successful builds
- PR #2863 - Replace casts/assignments with hard-coded memcpy operations
- Issue #2862 - sched_getcpu not available on MacOS
- PR #2861 - Fixing unmatched header defines and recursive inclusion of threadmanager
- Issue #2860 - Master program fails with assertion ‘type == data_type_address’ failed: HPX(assertion_failure)
- Issue #2852 - Support for ARM64
- PR #2858 - Fix misplaced #if #endif’s that cause build failure without THREAD_CUMULATIVE_COUNTS
- PR #2857 - Fix some listing in documentation
- PR #2856 - Fixing component handling for lcos
- PR #2855 - Add documentation for coarrays
- PR #2854 - Support ARM64 in timestamps
- PR #2853 - Update Table 17. Non-modifying Parallel Algorithms in Documentation
- PR #2851 - Allowing for non-default-constructible component types
- PR #2850 - Enable returning future<R> from actions where R is not default-constructible
- PR #2849 - Unify serialization of non-default-constructable types
- Issue #2848 - Components have to be default constructible
- Issue #2847 - Returning a future<R> where R is not default-constructable broken
- Issue #2846 - Unify serialization of non-default-constructible types
- PR #2845 - Add Visual Studio 2015 to the tested toolchains in Appveyor
- Issue #2844 - Change the appveyor build to use the minimal required MSVC version
- Issue #2843 - multi node hello_world hangs
- PR #2842 - Correcting Spelling mistake in docs
- PR #2841 - Fix usage of std::aligned_storage
- PR #2840 - Remove constexpr from a void function
- Issue #2839 - memcpy buffer overflow: load_construct_data() and std::complex members
- Issue #2835 -
constexpr
functions withvoid
return type break compilation with CUDA 8.0 - Issue #2834 - One suspicion in parallel::detail::handle_exception
- PR #2833 - Implement parallel::merge
- PR #2832 - Fix a strange thing in parallel::util::detail::handle_local_exceptions. (Fix #2818)
- PR #2830 - Break the debugger when a test failed
- Issue #2831 -
parallel/executors/execution_fwd.hpp
causes compilation failure in C++11 mode. - PR #2829 - Implement an API for asynchronous pack traversal
- PR #2828 - Split unit test builds on CircleCI to avoid timeouts
- Issue #2827 - failure to compile hello_world example with -Werror
- PR #2824 - Making sure promises are marked as started when used as continuations
- PR #2823 - Add documentation for partitioned_vector_view
- Issue #2822 - Yet another issue with wait_for similar to #2796
- PR #2821 - Fix bugs and improve that about HPX_HAVE_CXX11_AUTO_RETURN_VALUE of CMake
- PR #2820 - Support C++11 in benchmark codes of parallel::partition and parallel::partition_copy
- PR #2819 - Fix compile errors in unit test of container version of parallel::partition
- Issue #2818 - A strange thing in parallel::util::detail::handle_local_exceptions
- Issue #2815 - HPX fails to compile with HPX_WITH_CUDA=ON and the new CUDA 9.0 RC
- Issue #2814 - Using ‘gmakeN’ after ‘cmake’ produces error in src/CMakeFiles/hpx.dir/runtime/agas/addressing_service.cpp.o
- PR #2813 - Properly support [[noreturn]] attribute if available
- Issue #2812 - Compilation fails with gcc 7.1.1
- PR #2811 - Adding hpx::launch::lazy and support for async, dataflow, and future::then
- PR #2810 - Add option allowing to disable deprecation warning
- PR #2809 - Disable throttling scheduler if HWLOC is not found/used
- PR #2808 - Fix compile errors on some environments of parallel::partition
- Issue #2807 - Difficulty building with
HPX_WITH_HWLOC=Off
- PR #2806 - Partitioned vector
- PR #2805 - Serializing collections with non-default constructible data
- PR #2802 - Fix FreeBSD 11
- Issue #2801 - Rate limiting techniques in io_service
- Issue #2800 - New Launch Policy: async_if
- PR #2799 - Fix a unit test failure on GCC in tuple_cat
- PR #2798 - bump minimum required cmake to 3.0 in test
- PR #2797 - Making sure future::wait_for et.al. work properly for action results
- Issue #2796 - wait_for does always in “deferred” state for calls on remote localities
- Issue #2795 - Serialization of types without default constructor
- PR #2794 - Fixing test for partitioned_vector iteration
- PR #2792 - Implemented segmented find and its variations for partitioned vector
- PR #2791 - Circumvent scary warning about placement new
- PR #2790 - Fix OSX build
- PR #2789 - Resource partitioner
- PR #2788 - Adapt parallel::is_heap and parallel::is_heap_until to Ranges TS
- PR #2787 - Unwrap hotfixes
- PR #2786 - Update CMake Minimum Version to 3.3.2 (refs #2565)
- Issue #2785 - Issues with masks and cpuset
- PR #2784 - Error with reduce and transform reduce fixed
- PR #2783 - StackOverflow integration with libsigsegv
- PR #2782 - Replace boost::atomic with std::atomic (where possible)
- PR #2781 - Check for and optionally use [[deprecated]] attribute
- PR #2780 - Adding empty (but non-trivial) destructor to circumvent warnings
- PR #2779 - Exception info tweaks
- PR #2778 - Implement parallel::partition
- PR #2777 - Improve error handling in gather_here/gather_there
- PR #2776 - Fix a bug in compiler version check
- PR #2775 - Fix compilation when HPX_WITH_LOGGING is OFF
- PR #2774 - Removing dependency on Boost.Date_Time
- PR #2773 - Add sync_images() method to spmd_block class
- PR #2772 - Adding documentation for PAPI counters
- PR #2771 - Removing boost preprocessor dependency
- PR #2770 - Adding test, fixing deadlock in config registry
- PR #2769 - Remove some other warnings and errors detected by clang 5.0
- Issue #2768 - Is there iterator tag for HPX?
- PR #2767 - Improvements to continuation annotation
- PR #2765 - gcc split stack support for HPX threads #620
- PR #2764 - Fix some uses of begin/end, remove unnecessary includes
- PR #2763 - Bump minimal Boost version to 1.55.0
- PR #2762 - hpx::partitioned_vector serializer
- PR #2761 - Adding configuration summary to cmake output and –hpx:info
- PR #2760 - Removing 1d_hydro example as it is broken
- PR #2758 - Remove various warnings detected by clang 5.0
- Issue #2757 - In case of a “raw thread” is needed per core for implementing parallel algorithm, what is good practice in HPX?
- PR #2756 - Allowing for LCOs to be simple components
- PR #2755 - Removing make_index_pack_unrolled
- PR #2754 - Implement parallel::unique_copy
- PR #2753 - Fixing detection of [[fallthrough]] attribute
- PR #2752 - New thread priority names
- PR #2751 - Replace boost::exception with proposed exception_info
- PR #2750 - Replace boost::iterator_range
- PR #2749 - Fixing hdf5 examples
- Issue #2748 - HPX fails to build with enabled hdf5 examples
- Issue #2747 - Inherited task priorities break certain DAG optimizations
- Issue #2746 - HPX segfaulting with valgrind
- PR #2745 - Adding extended arithmetic performance counters
- PR #2744 - Adding ability to statistics counters to reset base counter
- Issue #2743 - Statistics counter does not support reseting
- PR #2742 - Making sure Vc V2 builds without additional HPX configuration flags
- PR #2741 - Deprecate unwrapped and implement unwrap and unwrapping
- PR #2740 - Coroutine stackoverflow detection for linux/posix; Issue #2408
- PR #2739 - Add files via upload
- PR #2738 - Appveyor support
- PR #2737 - Fixing 2735
- Issue #2736 - 1d_hydro example does’t work
- Issue #2735 - partitioned_vector_subview test failing
- PR #2734 - Add C++11 range utilities
- PR #2733 - Adapting iterator requirements for parallel algorithms
- PR #2732 - Integrate C++ Co-arrays
- PR #2731 - Adding on_migrated event handler to migratable component instances
- Issue #2729 - Add on_migrated() event handler to migratable components
- Issue #2728 - Why Projection is needed in parallel algorithms?
- PR #2727 - Cmake files for StackOverflow Detection
- PR #2726 - CMake for Stack Overflow Detection
- PR #2725 - Implemented segmented algorithms for partitioned vector
- PR #2724 - Fix examples in Action documentation
- PR #2723 - Enable lcos::channel<T>::register_as
- Issue #2722 - channel register_as() failing on compilation
- PR #2721 - Mind map
- PR #2720 - reorder forward declarations to get rid of C++14-only auto return types
- PR #2719 - Add documentation for partitioned_vector and add features in pack.hpp
- Issue #2718 - Some forward declarations in execution_fwd.hpp aren’t C++11-compatible
- PR #2717 - Config support for fallthrough attribute
- PR #2716 - Implement parallel::partition_copy
- PR #2715 - initial import of icu string serializer
- PR #2714 - initial import of valarray serializer
- PR #2713 - Remove slashes before CMAKE_FILES_DIRECTORY variables
- PR #2712 - Fixing wait for 1751
- PR #2711 - Adjust code for minimal supported GCC having being bumped to 4.9
- PR #2710 - Adding code of conduct
- PR #2709 - Fixing UB in destroy tests
- PR #2708 - Add inline to prevent multiple definition issue
- Issue #2707 - Multiple defined symbols for task_block.hpp in VS2015
- PR #2706 - Adding .clang-format file
- PR #2704 - Add a synchronous mapping API
- Issue #2703 - Request: Add the .clang-format file to the repository
- Issue #2702 - STEllAR-GROUP/Vc slower than VCv1 possibly due to wrong instructions generated
- Issue #2701 - Datapar with STEllAR-GROUP/Vc requires obscure flag
- Issue #2700 - Naming inconsistency in parallel algorithms
- Issue #2699 - Iterator requirements are different from standard in parallel copy_if.
- PR #2698 - Properly releasing parcelport write handlers
- Issue #2697 - Compile error in addressing_service.cpp
- Issue #2696 - Building and using HPX statically: undefined references from runtime_support_server.cpp
- Issue #2695 - Executor changes cause compilation failures
- PR #2694 - Refining C++ language mode detection for MSVC
- PR #2693 - P0443 r2
- PR #2692 - Partially reverting changes to parcel_await
- Issue #2689 - HPX build fails when HPX_WITH_CUDA is enabled
- PR #2688 - Make Cuda Clang builds pass
- PR #2687 - Add an is_tuple_like trait for sequenceable type detection
- PR #2686 - Allowing throttling scheduler to be used without idle backoff
- PR #2685 - Add support of std::array to hpx::util::tuple_size and tuple_element
- PR #2684 - Adding new statistics performance counters
- PR #2683 - Replace boost::exception_ptr with std::exception_ptr
- Issue #2682 - HPX does not compile with HPX_WITH_THREAD_MANAGER_IDLE_BACKOFF=OFF
- PR #2681 - Attempt to fix problem in managed_component_base
- PR #2680 - Fix bad size during archive creation
- Issue #2679 - Mismatch between size of archive and container
- Issue #2678 - In parallel algorithm, other tasks are executed to the end even if an exception occurs in any task.
- PR #2677 - Adding include check for std::addressof
- PR #2676 - Adding parallel::destroy and destroy_n
- PR #2675 - Making sure statistics counters work as expected
- PR #2674 - Turning assertions into exceptions
- PR #2673 - Inhibit direct conversion from future<future<T>> –> future<void>
- PR #2672 - C++17 invoke forms
- PR #2671 - Adding uninitialized_value_construct and uninitialized_value_construct_n
- PR #2670 - Integrate spmd multidimensionnal views for partitioned_vectors
- PR #2669 - Adding uninitialized_default_construct and uninitialized_default_construct_n
- PR #2668 - Fixing documentation index
- Issue #2667 - Ambiguity of nested hpx::future<void>’s
- Issue #2666 - Statistics Performance counter is not working
- PR #2664 - Adding uninitialized_move and uninitialized_move_n
- Issue #2663 - Seg fault in managed_component::get_base_gid, possibly cause by util::reinitializable_static
- Issue #2662 - Crash in managed_component::get_base_gid due to problem with util::reinitializable_static
- PR #2665 - Hide the
detail
namespace in doxygen per default - PR #2660 - Add documentation to hpx::util::unwrapped and hpx::util::unwrapped2
- PR #2659 - Improve integration with vcpkg
- PR #2658 - Unify access_data trait for use in both, serialization and de-serialization
- PR #2657 - Removing hpx::lcos::queue<T>
- PR #2656 - Reduce MAX_TERMINATED_THREADS default, improve memory use on manycore cpus
- PR #2655 - Mainteinance for emulate-deleted macros
- PR #2654 - Implement parallel is_heap and is_heap_until
- PR #2653 - Drop support for VS2013
- PR #2652 - This patch makes sure that all parcels in a batch are properly handled
- PR #2649 - Update docs (Table 18) - move transform to end
- Issue #2647 - hpx::parcelset::detail::parcel_data::has_continuation_ is unitialized
- Issue #2644 - Some .vcxproj in the HPX.sln fail to build
- Issue #2641 -
hpx::lcos::queue
should be deprecated - PR #2640 - A new throttling policy with public APIs to suspend/resume
- PR #2639 - Fix a tiny typo in tutorial.
- Issue #2638 - Invalid return type ‘void’ of constexpr function
- PR #2636 - Add and use HPX_MSVC_WARNING_PRAGMA for #pragma warning
- PR #2633 - Distributed define_spmd_block
- PR #2632 - Making sure container serialization uses size-compatible types
- PR #2631 - Add lcos::local::one_element_channel
- PR #2629 - Move unordered_map out of parcelport into hpx/concurrent
- PR #2628 - Making sure that shutdown does not hang
- PR #2627 - Fix serialization
- PR #2626 - Generate
cmake_variables.qbk
andcmake_toolchains.qbk
outside of the source tree - PR #2625 - Supporting -std=c++17 flag
- PR #2624 - Fixing a small cmake typo
- PR #2622 - Update CMake minimum required version to 3.0.2 (closes #2621)
- Issue #2621 - Compiling hpx master fails with /usr/bin/ld: final link failed: Bad value
- PR #2620 - Remove warnings due to some captured variables
- PR #2619 - LF multiple parcels
- PR #2618 - Some fixes to libfabric that didn’t get caught before the merge
- PR #2617 - Adding
hpx::local_new
- PR #2616 - Documentation: Extract all entities in order to autolink functions correctly
- Issue #2615 - Documentation: Linking functions is broken
- PR #2614 - Adding serialization for std::deque
- PR #2613 - We need to link with boost.thread and boost.chrono if we use boost.context
- PR #2612 - Making sure for_loop_n(par, …) is actually executed in parallel
- PR #2611 - Add documentation to invoke_fused and friends NFC
- PR #2610 - Added reduction templates using an identity value
- PR #2608 - Fixing some unused vars in inspect
- PR #2607 - Fixed build for mingw
- PR #2606 - Supporting generic context for boost >= 1.61
- PR #2605 - Parcelport libfabric3
- PR #2604 - Adding allocator support to promise and friends
- PR #2603 - Barrier hang
- PR #2602 - Changes to scheduler to steal from one high-priority queue
- Issue #2601 - High priority tasks are not executed first
- PR #2600 - Compat fixes
- PR #2599 - Compatibility layer for threading support
- PR #2598 - V1.1
- PR #2597 - Release V1.0
- PR #2592 - First attempt to introduce spmd_block in hpx
- PR #2586 - local_segment in segmented_iterator_traits
- Issue #2584 - Add allocator support to promise, packaged_task and friends
- PR #2576 - Add missing dependencies of cuda based tests
- PR #2575 - Remove warnings due to some captured variables
- Issue #2574 - MSVC 2015 Compiler crash when building HPX
- Issue #2568 - Remove throttle_scheduler as it has been abandoned
- Issue #2566 - Add an inline versioning namespace before 1.0 release
- Issue #2565 - Raise minimal cmake version requirement
- PR #2556 - Fixing scan partitioner
- PR #2546 - Broadcast async
- Issue #2543 - make install fails due to a non-existing .so file
- PR #2495 - wait_or_add_new returning thread_id_type
- Issue #2480 - Unable to register new performance counter
- Issue #2471 - no type named ‘fcontext_t’ in namespace
- Issue #2456 - Re-implement hpx::util::unwrapped
- Issue #2455 - Add more arithmetic performance counters
- PR #2454 - Fix a couple of warnings and compiler errors
- PR #2453 - Timed executor support
- PR #2447 - Implementing new executor API (P0443)
- Issue #2439 - Implement executor proposal
- Issue #2408 - Stackoverflow detection for linux, e.g. based on libsigsegv
- PR #2377 - Add a customization point for put_parcel so we can override actions
- Issue #2368 - HPX_ASSERT problem
- Issue #2324 - Change default number of threads used to the maximum of the system
- Issue #2266 - hpx_0.9.99 make tests fail
- PR #2195 - Support for code completion in VIM
- Issue #2137 - Hpx does not compile over osx
- Issue #2092 - make tests should just build the tests
- Issue #2026 - Build HPX with Apple’s clang
- Issue #1932 - hpx with PBS fails on multiple localities
- PR #1914 - Parallel heap algorithm implementations WIP
- Issue #1598 - Disconnecting a locality results in segfault using heartbeat example
- Issue #1404 - unwrapped doesn’t work with movable only types
- Issue #1400 - hpx::util::unwrapped doesn’t work with non-future types
- Issue #1205 - TSS is broken
- Issue #1126 - vector<future<T> > does not work gracefully with dataflow, when_all and unwrapped
- Issue #1056 - Thread manager cleanup
- Issue #863 - Futures should not require a default constructor
- Issue #856 - Allow runtimemode_connect to be used with security enabled
- Issue #726 - Valgrind
- Issue #701 - Add RCR performance counter component
- Issue #528 - Add support for known failures and warning count/comparisons to hpx_run_tests.py
HPX V1.0.0 (Apr 24, 2017)¶
General changes¶
Here are some of the main highlights and changes for this release (in no particular order):
- Added the facility
hpx::split_future
which allows to convert afuture<tuple<Ts...>>
into atuple<future<Ts>...>
. This functionality is not available when compiling HPX with VS2012. - Added a new type of performance counter which allows to return a list of values for each invocation. We also added a first counter of this type which collects a histogram of the times between parcels being created.
- Added new LCOs:
hpx::lcos::channel
andhpx::lcos::local::channel
which are very similar to the well known channel constructs used in the Go language. - Added new performance counters reporting the amount of data handled by the networking layer on a action-by-action basis (please see PR #2289 for more details).
- Added a new facility
hpx::lcos::barrier
, replacing the equally named older one. The new facility has a slightly changed API and is much more efficient. Most notable, the new facility exposes a (global) functionhpx::lcos::barrier::synchronize()
which represents a global barrier across all localities. - We have started to add support for vectorization to our parallel algorithm implementations. This support depends on using an external library, currently either Vc Library or Boost.SIMD. Please see Issue #2333 for a list of currently supported algorithms. This is an experimental feature and its implementation and/or API might change in the future. Please see this blog-post for more information.
- The parameter sequence for the
hpx::parallel::transform_reduce
overload taking one iterator range has changed to match the changes this algorithm has undergone while being moved to C++17. The old overload can be still enabled at configure time by specifying-DHPX_WITH_TRANSFORM_REDUCE_COMPATIBILITY=On
to CMake. - The algorithm
hpx::parallel::inner_product
has been renamed tohpx::parallel::transform_reduce
to match the changes this algorithm has undergone while being moved to C++17. The old inner_product names can be still enabled at configure time by specifying-DHPX_WITH_TRANSFORM_REDUCE_COMPATIBILITY=On
to CMake. - Added versions of
hpx::get_ptr
taking client side representations for component instances as their parameter (instead of a global id). - Added the helper utility
hpx::performance_counters::performance_counter_set
helping to encapsulate a set of performance counters to be managed concurrently. - All execution policies and related classes have been renamed to be consistent
with the naming changes applied for C++17. All policies now live in the
namespace
hpx::parallel::execution
. The ols names can be still enabled at configure time by specifying-DHPX_WITH_EXECUTION_POLICY_COMPATIBILITY=On
to CMake. - The thread scheduling subsystem has undergone a major refactoring which
results in significant performance improvements. We have also imroved the
performance of creating
hpx::future
and of various facilities handling those. - We have consolidated all of the code in HPX.Compute related to the integration
of CUDA.
hpx::partitioned_vector
has been enabled to be usable withhpx::compute::vector
which allows to place the partitions on one or more GPU devices. - Added new performance counters exposing various internals of the thread scheduling subsystem, such as the current idle- and busy-loop counters and instantaneous scheduler utilization.
- Extended and improved the use of the ITTNotify hooks allowing to collect performance counter data and function annotation information from within the Intel Amplifier tool.
Breaking changes¶
- We have dropped support for the gcc compiler versions V4.6 and 4.7. The minimal gcc version we now test on is gcc V4.8.
- We have removed (default) support for
boost::chrono
in interfaces, uses of it have been replaced withstd::chrono
. This facility can be still enabled at configure time by specifying-DHPX_WITH_BOOST_CHRONO_COMPATIBILITY=On
to CMake. - The parameter sequence for the
hpx::parallel::transform_reduce
overload taking one iterator range has changed to match the changes this algorithm has undergone while being moved to C++17. - The algorithm
hpx::parallel::inner_product
has been renamed tohpx::parallel::transform_reduce
to match the changes this algorithm has undergone while being moved to C++17. - the build options
HPX_WITH_COLOCATED_BACKWARDS_COMPATIBILITY
andHPX_WITH_COMPONENT_GET_GID_COMPATIBILITY
are now disabled by default. Please change your code still depending on the deprecated interfaces.
Bug fixes (closed tickets)¶
Here is a list of the important tickets we closed for this release.
- PR #2596 - Adding apex data
- PR #2595 - Remove obsolete file
- Issue #2594 - FindOpenCL.cmake mismatch with the official cmake module
- PR #2592 - First attempt to introduce spmd_block in hpx
- Issue #2591 - Feature request: continuation (then) which does not require the callable object to take a future<R> as parameter
- PR #2588 - Daint fixes
- PR #2587 - Fixing transfer_(continuation)_action::schedule
- PR #2585 - Work around MSVC having an ICE when compiling with -Ob2
- PR #2583 - chaning 7zip command to 7za in roll_release.sh
- PR #2582 - First attempt to introduce spmd_block in hpx
- PR #2581 - Enable annotated function for parallel algorithms
- PR #2580 - First attempt to introduce spmd_block in hpx
- PR #2579 - Make thread NICE level setting an option
- PR #2578 - Implementing enqueue instead of busy wait when no sender is available
- PR #2577 - Retrieve -std=c++11 consistent nvcc flag
- PR #2576 - Add missing dependencies of cuda based tests
- PR #2575 - Remove warnings due to some captured variables
- PR #2573 - Attempt to resolve resolve_locality
- PR #2572 - Adding APEX hooks to background thread
- PR #2571 - Pick up hpx.ignore_batch_env from config map
- PR #2570 - Add commandline options –hpx:print-counters-locally
- PR #2569 - Fix computeapi unit tests
- PR #2567 - This adds another barrier::synchronize before registering performance counters
- PR #2564 - Cray static toolchain support
- PR #2563 - Fixed unhandled exception during startup
- PR #2562 - Remove partitioned_vector.cu from build tree when nvcc is used
- Issue #2561 - octo-tiger crash with commit 6e921495ff6c26f125d62629cbaad0525f14f7ab
- PR #2560 - Prevent -Wundef warnings on Vc version checks
- PR #2559 - Allowing CUDA callback to set the future directly from an OS thread
- PR #2558 - Remove warnings due to float precisions
- PR #2557 - Removing bogus handling of compile flags for CUDA
- PR #2556 - Fixing scan partitioner
- PR #2554 - Add more diagnostics to error thrown from find_appropriate_destination
- Issue #2555 - No valid parcelport configured
- PR #2553 - Add cmake cuda_arch option
- PR #2552 - Remove incomplete datapar bindings to libflatarray
- PR #2551 - Rename hwloc_topology to hwloc_topology_info
- PR #2550 - Apex api updates
- PR #2549 - Pre-include defines.hpp to get the macro HPX_HAVE_CUDA value
- PR #2548 - Fixing issue with disconnect
- PR #2546 - Some fixes around cuda clang partitioned_vector example
- PR #2545 - Fix uses of the Vc2 datapar flags; the value, not the type, should be passed to functions
- PR #2542 - Make HPX_WITH_MALLOC easier to use
- PR #2541 - avoid recompiles when enabling/disabling examples
- PR #2540 - Fixing usage of target_link_libraries()
- PR #2539 - fix RPATH behaviour
- Issue #2538 - HPX_WITH_CUDA corrupts compilation flags
- PR #2537 - Add output of a Bazel Skylark extension for paths and compile options
- PR #2536 - Add counter exposing total available memory to Windows as well
- PR #2535 - Remove obsolete support for security
- Issue #2534 - Remove command line option
--hpx:run-agas-server
- PR #2533 - Pre-cache locality endpoints during bootstrap
- PR #2532 - Fixing handling of GIDs during serialization preprocessing
- PR #2531 - Amend uses of the term “functor”
- PR #2529 - added counter for reading available memory
- PR #2527 - Facilities to create actions from lambdas
- PR #2526 - Updated docs: HPX_WITH_EXAMPLES
- PR #2525 - Remove warnings related to unused captured variables
- Issue #2524 - CMAKE failed because it is missing: TCMALLOC_LIBRARY TCMALLOC_INCLUDE_DIR
- PR #2523 - Fixing compose_cb stack overflow
- PR #2522 - Instead of unlocking, ignore the lock while creating the message handler
- PR #2521 - Create
LPROGRESS_
logging macro to simplify progress tracking and timings - PR #2520 - Intel 17 support
- PR #2519 - Fix components example
- PR #2518 - Fixing parcel scheduling
- Issue #2517 - Race condition during Parcel Coalescing Handler creation
- Issue #2516 - HPX locks up when using at least 256 localities
- Issue #2515 - error: Install cannot find “/lib/hpx/libparcel_coalescing.so.0.9.99” but I can see that file
- PR #2514 - Making sure that all continuations of a shared_future are invoked in order
- PR #2513 - Fixing locks held during suspension
- PR #2512 - MPI Parcelport improvements and fixes related to the background work changes
- PR #2511 - Fixing bit-wise (zero-copy) serialization
- Issue #2509 - Linking errors in hwloc_topology
- PR #2508 - Added documentation for debugging with core files
- PR #2506 - Fixing background work invocations
- PR #2505 - Fix tuple serialization
- Issue #2504 - Ensure continuations are called in the order they have been attached
- PR #2503 - Adding serialization support for Vc v2 (datapar)
- PR #2502 - Resolve various, minor compiler warnings
- PR #2501 - Some other fixes around cuda examples
- Issue #2500 - nvcc / cuda clang issue due to a missing -DHPX_WITH_CUDA flag
- PR #2499 - Adding support for std::array to wait_all and friends
- PR #2498 - Execute background work as HPX thread
- PR #2497 - Fixing configuration options for spinlock-deadlock detection
- PR #2496 - Accounting for different compilers in CrayKNL toolchain file
- PR #2494 - Adding component base class which ties a component instance to a given executor
- PR #2493 - Enable controlling amount of pending threads which must be available to allow thread stealing
- PR #2492 - Adding new command line option –hpx:print-counter-reset
- PR #2491 - Resolve ambiguities when compiling with APEX
- PR #2490 - Resuming threads waiting on future with higher priority
- Issue #2489 - nvcc issue because -std=c++11 appears twice
- PR #2488 - Adding performance counters exposing the internal idle and busy-loop counters
- PR #2487 - Allowing for plain suspend to reschedule thread right away
- PR #2486 - Only flag HPX code for CUDA if HPX_WITH_CUDA is set
- PR #2485 - Making thread-queue parameters runtime-configurable
- PR #2484 - Added atomic counter for parcel-destinations
- PR #2483 - Added priority-queue lifo scheduler
- PR #2482 - Changing scheduler to steal only if more than a minimal number of tasks are available
- PR #2481 - Extending command line option –hpx:print-counter-destination to support value ‘none’
- PR #2479 - Added option to disable signal handler
- PR #2478 - Making sure the sine performance counter module gets loaded only for the corresponding example
- Issue #2477 - Breaking at a throw statement
- PR #2476 - Annotated function
- PR #2475 - Ensure that using %osthread% during logging will not throw for non-hpx threads
- PR #2474 - Remove now superficial non_direct actions from base_lco and friends
- PR #2473 - Refining support for ITTNotify
- PR #2472 - Some fixes around hpx compute
- Issue #2470 - redefinition of boost::detail::spinlock
- Issue #2469 - Dataflow performance issue
- PR #2468 - Perf docs update
- PR #2466 - Guarantee to execute remote direct actions on HPX-thread
- PR #2465 - Improve demo : Async copy and fixed device handling
- PR #2464 - Adding performance counter exposing instantaneous scheduler utilization
- PR #2463 - Downcast to future<void>
- PR #2462 - Fixed usage of ITT-Notify API with Intel Amplifier
- PR #2461 - Cublas demo
- PR #2460 - Fixing thread bindings
- PR #2459 - Make -std=c++11 nvcc flag consistent for in-build and installed versions
- Issue #2457 - Segmentation fault when registering a partitioned vector
- PR #2452 - Properly releasing global barrier for unhandled exceptions
- PR #2451 - Fixing long shutdown times
- PR #2450 - Attempting to fix initialization errors on newer platforms (Boost V1.63)
- PR #2449 - Replace BOOST_COMPILER_FENCE with an HPX version
- PR #2448 - This fixes a possible race in the migration code
- PR #2445 - Fixing dataflow et.al. for futures or future-ranges wrapped
- into ref()
- PR #2444 - Fix segfaults
- PR #2443 - Issue 2442
- Issue #2442 - Mismatch between #if/#endif and namespace scope brackets in this_thread_executers.hpp
- Issue #2441 - undeclared identifier BOOST_COMPILER_FENCE
- PR #2440 - Knl build
- PR #2438 - Datapar backend
- PR #2437 - Adapt algorithm parameter sequence changes from C++17
- PR #2436 - Adapt execution policy name changes from C++17
- Issue #2435 - Trunk broken, undefined reference to hpx::thread::interrupt(hpx::thread::id, bool)
- PR #2434 - More fixes to resource manager
- PR #2433 - Added versions of
hpx::get_ptr
taking client side representations - PR #2432 - Warning fixes
- PR #2431 - Adding facility representing set of performance counters
- PR #2430 - Fix parallel_executor thread spawning
- PR #2429 - Fix attribute warning for gcc
- Issue #2427 - Seg fault running octo-tiger with latest HPX commit
- Issue #2426 - Bug in 9592f5c0bc29806fce0dbe73f35b6ca7e027edcb causes immediate crash in Octo-tiger
- PR #2425 - Fix nvcc errors due to constexpr specifier
- Issue #2424 - Async action on component present on hpx::find_here is executing synchronously
- PR #2423 - Fix nvcc errors due to constexpr specifier
- PR #2422 - Implementing hpx::this_thread thread data functions
- PR #2421 - Adding benchmark for wait_all
- Issue #2420 - Returning object of a component client from another component action fails
- PR #2419 - Infiniband parcelport
- Issue #2418 - gcc + nvcc fails to compile code that uses partitioned_vector
- PR #2417 - Fixing context switching
- PR #2416 - Adding fixes and workarounds to allow compilation with nvcc/msvc (VS2015up3)
- PR #2415 - Fix errors coming from hpx compute examples
- PR #2414 - Fixing msvc12
- PR #2413 - Enable cuda/nvcc or cuda/clang when using add_hpx_executable()
- PR #2412 - Fix issue in HPX_SetupTarget.cmake when cuda is used
- PR #2411 - This fixes the core compilation issues with MSVC12
- Issue #2410 -
undefined reference to opal_hwloc191_hwloc_.....
- PR #2409 - Fixing locking for channel and receive_buffer
- PR #2407 - Solving #2402 and #2403
- PR #2406 - Improve guards
- PR #2405 - Enable parallel::for_each for iterators returning proxy types
- PR #2404 - Forward the explicitly given result_type in the hpx invoke
- Issue #2403 - datapar_execution + zip iterator: lambda arguments aren’t references
- Issue #2402 - datapar algorithm instantiated with wrong type #2402
- PR #2401 - Added support for imported libraries to HPX_Libraries.cmake
- PR #2400 - Use CMake policy CMP0060
- Issue #2399 - Error trying to push back vector of futures to vector
- PR #2398 - Allow config #defines to be written out to custom config/defines.hpp
- Issue #2397 - CMake generated config defines can cause tedious rebuilds category
- Issue #2396 - BOOST_ROOT paths are not used at link time
- PR #2395 - Fix target_link_libraries() issue when HPX Cuda is enabled
- Issue #2394 - Template compilation error using HPX_WITH_DATAPAR_LIBFLATARRAY
- PR #2393 - Fixing lock registration for recursive mutex
- PR #2392 - Add keywords in target_link_libraries in hpx_setup_target
- PR #2391 - Clang goroutines
- Issue #2390 - Adapt execution policy name changes from C++17
- PR #2389 - Chunk allocator and pool are not used and are obsolete
- PR #2388 - Adding functionalities to datapar needed by octotiger
- PR #2387 - Fixing race condition for early parcels
- Issue #2386 - Lock registration broken for recursive_mutex
- PR #2385 - Datapar zip iterator
- PR #2384 - Fixing race condition in for_loop_reduction
- PR #2383 - Continuations
- PR #2382 - add LibFlatArray-based backend for datapar
- PR #2381 - remove unused typedef to get rid of compiler warnings
- PR #2380 - Tau cleanup
- PR #2379 - Can send immediate
- PR #2378 - Renaming copy_helper/copy_n_helper/move_helper/move_n_helper
- Issue #2376 - Boost trunk’s spinlock initializer fails to compile
- PR #2375 - Add support for minimal thread local data
- PR #2374 - Adding API functions set_config_entry_callback
- PR #2373 - Add a simple utility for debugging that gives supended task backtraces
- PR #2372 - Barrier Fixes
- Issue #2370 - Can’t wait on a wrapped future
- PR #2369 - Fixing stable_partition
- PR #2367 - Fixing find_prefixes for Windows platforms
- PR #2366 - Testing for experimental/optional only in C++14 mode
- PR #2364 - Adding set_config_entry
- PR #2363 - Fix papi
- PR #2362 - Adding missing macros for new non-direct actions
- PR #2361 - Improve cmake output to help debug compiler incompatibility check
- PR #2360 - Fixing race condition in condition_variable
- PR #2359 - Fixing shutdown when parcels are still in flight
- Issue #2357 - failed to insert console_print_action into typename_to_id_t registry
- PR #2356 - Fixing return type of get_iterator_tuple
- PR #2355 - Fixing compilation against Boost 1 62
- PR #2354 - Adding serialization for mask_type if CPU_COUNT > 64
- PR #2353 - Adding hooks to tie in APEX into the parcel layer
- Issue #2352 - Compile errors when using intel 17 beta (for KNL) on edison
- PR #2351 - Fix function vtable get_function_address implementation
- Issue #2350 - Build failure - master branch (4de09f5) with Intel Compiler v17
- PR #2349 - Enabling zero-copy serialization support for std::vector<>
- PR #2348 - Adding test to verify #2334 is fixed
- PR #2347 - Bug fixes for hpx.compute and hpx::lcos::channel
- PR #2346 - Removing cmake “find” files that are in the APEX cmake Modules
- PR #2345 - Implemented parallel::stable_partition
- PR #2344 - Making hpx::lcos::channel usable with basename registration
- PR #2343 - Fix a couple of examples that failed to compile after recent api changes
- Issue #2342 - Enabling APEX causes link errors
- PR #2341 - Removing cmake “find” files that are in the APEX cmake Modules
- PR #2340 - Implemented all existing datapar algorithms using Boost.SIMD
- PR #2339 - Fixing 2338
- PR #2338 - Possible race in sliding semaphore
- PR #2337 - Adjust osu_latency test to measure window_size parcels in flight at once
- PR #2336 - Allowing remote direct actions to be executed without spawning a task
- PR #2335 - Making sure multiple components are properly initialized from arguments
- Issue #2334 - Cannot construct component with large vector on a remote locality
- PR #2332 - Fixing hpx::lcos::local::barrier
- PR #2331 - Updating APEX support to include OTF2
- PR #2330 - Support for data-parallelism for parallel algorithms
- Issue #2329 - Coordinate settings in cmake
- PR #2328 - fix LibGeoDecomp builds with HPX + GCC 5.3.0 + CUDA 8RC
- PR #2326 - Making scan_partitioner work (for now)
- Issue #2323 - Constructing a vector of components only correctly initializes the first component
- PR #2322 - Fix problems that bubbled up after merging #2278
- PR #2321 - Scalable barrier
- PR #2320 - Std flag fixes
- Issue #2319 - -std=c++14 and -std=c++1y with Intel can’t build recent Boost builds due to insufficient C++14 support; don’t enable these flags by default for Intel
- PR #2318 - Improve handling of –hpx:bind=<bind-spec>
- PR #2317 - Making sure command line warnings are printed once only
- PR #2316 - Fixing command line handling for default bind mode
- PR #2315 - Set id_retrieved if set_id is present
- Issue #2314 - Warning for requested/allocated thread discrepancy is printed twice
- Issue #2313 - –hpx:print-bind doesn’t work with –hpx:pu-step
- Issue #2312 - –hpx:bind range specifier restrictions are overly restrictive
- Issue #2311 - hpx_0.9.99 out of project build fails
- PR #2310 - Simplify function registration
- PR #2309 - Spelling and grammar revisions in documentation (and some code)
- PR #2306 - Correct minor typo in the documentation
- PR #2305 - Cleaning up and fixing parcel coalescing
- PR #2304 - Inspect checks for stream related includes
- PR #2303 - Add functionality allowing to enumerate threads of given state
- PR #2301 - Algorithm overloads fix for VS2013
- PR #2300 - Use <cstdint>, add inspect checks
- PR #2299 - Replace boost::[c]ref with std::[c]ref, add inspect checks
- PR #2297 - Fixing compilation with no hw_loc
- PR #2296 - Hpx compute
- PR #2295 - Making sure for_loop(execution::par, 0, N, …) is actually executed in parallel
- PR #2294 - Throwing exceptions if the runtime is not up and running
- PR #2293 - Removing unused parcel port code
- PR #2292 - Refactor function vtables
- PR #2291 - Fixing 2286
- PR #2290 - Simplify algorithm overloads
- PR #2289 - Adding performance counters reporting parcel related data on a per-action basis
- Issue #2288 - Remove dormant parcelports
- Issue #2286 - adjustments to parcel handling to support parcelports that do not need a connection cache
- PR #2285 - add CMake option to disable package export
- PR #2283 - Add more inspect checks for use of deprecated components
- Issue #2282 - Arithmetic exception in executor static chunker
- Issue #2281 - For loop doesn’t parallelize
- PR #2280 - Fixing 2277: build failure with PAPI
- PR #2279 - Child vs parent stealing
- Issue #2277 - master branch build failure (53c5b4f) with papi
- PR #2276 - Compile time launch policies
- PR #2275 - Replace boost::chrono with std::chrono in interfaces
- PR #2274 - Replace most uses of Boost.Assign with initializer list
- PR #2273 - Fixed typos
- PR #2272 - Inspect checks
- PR #2270 - Adding test verifying -Ihpx.os_threads=all
- PR #2269 - Added inspect check for now obsolete boost type traits
- PR #2268 - Moving more code into source files
- Issue #2267 - Add inspect support to deprecate Boost.TypeTraits
- PR #2265 - Adding channel LCO
- PR #2264 - Make support for std::ref mandatory
- PR #2263 - Constrain tuple_member forwarding constructor
- Issue #2262 - Test hpx.os_threads=all
- Issue #2261 - OS X: Error: no matching constructor for initialization of ‘hpx::lcos::local::condition_variable_any’
- Issue #2260 - Make support for std::ref mandatory
- PR #2259 - Remove most of Boost.MPL, Boost.EnableIf and Boost.TypeTraits
- PR #2258 - Fixing #2256
- PR #2257 - Fixing launch process
- Issue #2256 - Actions are not registered if not invoked
- PR #2255 - Coalescing histogram
- PR #2254 - Silence explicit initialization in copy-constructor warnings
- PR #2253 - Drop support for GCC 4.6 and 4.7
- PR #2252 - Prepare V1.0
- PR #2251 - Convert to 0.9.99
- PR #2249 - Adding iterator_facade and iterator_adaptor
- Issue #2248 - Need a feature to yield to a new task immediately
- PR #2246 - Adding split_future
- PR #2245 - Add an example for handing over a component instance to a dynamically launched locality
- Issue #2243 - Add example demonstrating AGAS symbolic name registration
- Issue #2242 - pkgconfig test broken on CentOS 7 / Boost 1.61
- Issue #2241 - Compilation error for partitioned vector in hpx_compute branch
- PR #2240 - Fixing termination detection on one locality
- Issue #2239 - Create a new facility lcos::split_all
- Issue #2236 - hpx::cout vs. std::cout
- PR #2232 - Implement local-only primary namespace service
- Issue #2147 - would like to know how much data is being routed by particular actions
- Issue #2109 - Warning while compiling hpx
- Issue #1973 - Setting INTERFACE_COMPILE_OPTIONS for hpx_init in CMake taints Fortran_FLAGS
- Issue #1864 - run_guarded using bound function ignores reference
- Issue #1754 - Running with TCP parcelport causes immediate crash or freeze
- Issue #1655 - Enable zip_iterator to be used with Boost traversal iterator categories
- Issue #1591 - Optimize AGAS for shared memory only operation
- Issue #1401 - Need an efficient infiniband parcelport
- Issue #1125 - Fix the IPC parcelport
- Issue #839 - Refactor ibverbs and shmem parcelport
- Issue #702 - Add instrumentation of parcel layer
- Issue #668 - Implement ispc task interface
- Issue #533 - Thread queue/deque internal parameters should be runtime configurable
- Issue #475 - Create a means of combining performance counters into querysets
HPX V0.9.99 (Jul 15, 2016)¶
General changes¶
As the version number of this release hints, we consider this release to be a preview for the upcoming HPX V1.0. All of the functionalities we set out to implement for V1.0 are in place; all of the features we wanted to have exposed are ready. We are very happy with the stability and performance of HPX and we would like to present this release to the community in order for us to gather broad feedback before releasing V1.0. We still expect for some minor details to change, but on the whole this release represents what we would like to have in a V1.0.
Overall, since the last release we have had almost 1600 commits while closing almost 400 tickets. These numbers reflect the incredible development activity we have seen over the last couple of months. We would like to express a big ‘Thank you!’ to all contributors and those who helped to make this release happen.
The most notable addition in terms of new functionality available with this release is the full implementation of object migration (i.e. the ability to transparently move HPX components to a different compute node). Additionally, this release of HPX cleans up many minor issues and some API inconsistencies.
Here are some of the main highlights and changes for this release (in no particular order):
- We have fixed a couple of issues in AGAS and the parcel layer which have caused hangs, segmentation faults at exit, and a slowdown of applications over time. Fixing those has significantly increased the overall stability and performance of distributed runs.
- We have started to add parallel algorithm overloads based on the C++ Extensions for Ranges (N4560) proposal. This also includes the addition of projections to the existing algorithms. Please see Issue #1668 for a list of algorithms which have been adapted to N4560.
- We have implemented index-based parallel for-loops based on a corresponding standardization proposal (P0075R1). Please see Issue #2016 for a list of available algorithms.
- We have added implementations for more parallel algorithms as proposed for the upcoming C++ 17 Standard. See Issue #1141 for an overview of which algorithms are available by now.
- We have started to implement a new prototypical functionality with HPX.Compute which uniformly exposes some of the higher level APIs to heterogeneous architectures (currently CUDA). This functionality is an early preview and should not be considered stable. It may change considerably in the future.
- We have pervasively added (optional) executor arguments to all API functions which schedule new work. Executors are now used throughout the code base as the main means of executing tasks.
- Added
hpx::make_future<R>(future<T> &&)
allowing to convert a future of any typeT
into a future of any other typeR
, either based on default conversion rules of the embedded types or using a given explicit conversion function. - We finally finished the implementation of transparent migration of components to another locality. It is now possible to trigger a migration operation without ‘stopping the world’ for the object to migrate. HPX will make sure that no work is being performed on an object before it is migrated and that all subsequently scheduled work for the migrated object will be transparently forwarded to the new locality. Please note that the global id of the migrated object does not change, thus the application will not have to be changed in any way to support this new functionality. Please note that this feature is currently considered experimental. See Issue #559 and PR #1966 for more details.
- The
hpx::dataflow
facility is now usable with actions. Similarly tohpx::async
, actions can be specified as an explicit template argument (hpx::dataflow<Action>(target, ...)
) or as the first argument (hpx::dataflow(Action(), target, ...)
). We have also enabled the use of distribution policies as the target for dataflow invocations. Please see Issue #1265 and PR #1912 for more information. - Adding overloads of
gather_here
andgather_there
to accept the plain values of the data to gather (in addition to the existing overloads expecting futures). - We have cleaned up and refactored large parts of the code base. This helped reducing compile and link times of HPX itself and also of applications depending on it. We have further decreased the dependency of HPX on the Boost libraries by replacing part of those with facilities available from the standard libraries.
- Wherever possible we have removed dependencies of our API on Boost by replacing those with the equivalent facility from the C++11 standard library.
- We have added new performance counters for parcel coalescing, file-IO, the AGAS cache, and overall scheduler time. Resetting performance counters has been overhauled and fixed.
- We have introduced a generic client type
hpx::components::client<>
and added support for using it withhpx::async
. This removes the necessity to implement specific client types for every component type without losing type safety. This deemphasizes the need for using the low levelhpx::id_type
for referencing (possibly remote) component instances. The plan is to deprecate the direct use ofhpx::id_type
in user code in the future. - We have added a special iterator which supports automatic prefetching of one
or more arrays for speeding up loop-like code (see
hpx::parallel::util::make_prefetcher_context()
). - We have extended the interfaces exposed from executors (as proposed by N4406) to accept an arbitrary number of arguments.
Breaking changes¶
- In order to move the dataflow facility to
namespace hpx
we added a definition ofhpx::dataflow
which might create ambiguities in existing codes. The previous definition of this facility (hpx::lcos::local::dataflow
) has been deprecated and is available only if the constant-DHPX_WITH_LOCAL_DATAFLOW_COMPATIBILITY=On
to CMake is defined at configuration time. Please explicitly qualify all uses of the dataflow facility if you enable this compatibility setting and encounter ambiguities. - The adaptation of the C++ Extensions for Ranges (N4560) proposal imposes some breaking changes related to the return types of some of the parallel algorithms. Please see Issue #1668 for a list of algorithms which have already been adapted.
- The facility
hpx::lcos::make_future_void()
has been replaced byhpx::make_future<void>()
. - We have removed support for Intel V13 and gcc 4.4.x.
- We have removed (default) support for the generic
hpx::parallel::execution_poliy
because it was removed from the Parallelism TS (__cpp11_n4104__) while it was being added to the upcoming C++17 Standard. This facility can be still enabled at configure time by specifying-DHPX_WITH_GENERIC_EXECUTION_POLICY=On
to CMake. - Uses of
boost::shared_ptr
and related facilities have been replaced withstd::shared_ptr
and friends. Uses ofboost::unique_lock
,boost::lock_guard
etc. have also been replaced by the equivalent (and equally named) tools available from the C++11 standard library. - Facilities that used to expect an explicit
boost::unique_lock
now take anstd::unique_lock
. Additionally,condition_variable
no longer aliasescondition_variable_any
; its interface now only works withstd::unique_lock<local::mutex>
. - Uses of
boost::function
,boost::bind
,boost::tuple
have been replaced by the corresponding facilities in HPX (hpx::util::function
,hpx::util::bind
, andhpx::util::tuple
, respectively).
Bug fixes (closed tickets)¶
Here is a list of the important tickets we closed for this release.
- PR #2250 - change default chunker of parallel executor to static one
- PR #2247 - HPX on ppc64le
- PR #2244 - Fixing MSVC problems
- PR #2238 - Fixing small typos
- PR #2237 - Fixing small typos
- PR #2234 - Fix broken add test macro when extra args are passed in
- PR #2231 - Fixing possible race during future awaiting in serialization
- PR #2230 - Fix stream nvcc
- PR #2229 - Fixed run_as_hpx_thread
- PR #2228 - On prefetching_test branch : adding prefetching_iterator and related tests used for prefetching containers within lambda functions
- PR #2227 - Support for HPXCL’s opencl::event
- PR #2226 - Preparing for release of V0.9.99
- PR #2225 - fix issue when compiling components with hpxcxx
- PR #2224 - Compute alloc fix
- PR #2223 - Simplify promise
- PR #2222 - Replace last uses of boost::function by util::function_nonser
- PR #2221 - Fix config tests
- PR #2220 - Fixing gcc 4.6 compilation issues
- PR #2219 - nullptr support for
[unique_]function
- PR #2218 - Introducing clang tidy
- PR #2216 - Replace NULL with nullptr
- Issue #2214 - Let inspect flag use of NULL, suggest nullptr instead
- PR #2213 - Require support for nullptr
- PR #2212 - Properly find jemalloc through pkg-config
- PR #2211 - Disable a couple of warnings reported by Intel on Windows
- PR #2210 - Fixed host::block_allocator::bulk_construct
- PR #2209 - Started to clean up new sort algorithms, made things compile for sort_by_key
- PR #2208 - A couple of fixes that were exposed by a new sort algorithm
- PR #2207 - Adding missing includes in /hpx/include/serialization.hpp
- PR #2206 - Call package_action::get_future before package_action::apply
- PR #2205 - The indirect_packaged_task::operator() needs to be run on a HPX thread
- PR #2204 - Variadic executor parameters
- PR #2203 - Delay-initialize members of partitoned iterator
- PR #2202 - Added segmented fill for hpx::vector
- Issue #2201 - Null Thread id encountered on partitioned_vector
- PR #2200 - Fix hangs
- PR #2199 - Deprecating hpx/traits.hpp
- PR #2198 - Making explicit inclusion of external libraries into build
- PR #2197 - Fix typo in QT CMakeLists
- PR #2196 - Fixing a gcc warning about attributes being ignored
- PR #2194 - Fixing partitioned_vector_spmd_foreach example
- Issue #2193 - partitioned_vector_spmd_foreach seg faults
- PR #2192 - Support Boost.Thread v4
- PR #2191 - HPX.Compute prototype
- PR #2190 - Spawning operation on new thread if remaining stack space becomes too small
- PR #2189 - Adding callback taking index and future to when_each
- PR #2188 - Adding new example demonstrating receive_buffer
- PR #2187 - Mask 128-bit ints if CUDA is being used
- PR #2186 - Make startup & shutdown functions unique_function
- PR #2185 - Fixing logging output not to cause hang on shutdown
- PR #2184 - Allowing component clients as action return types
- Issue #2183 - Enabling logging output causes hang on shutdown
- Issue #2182 - 1d_stencil seg fault
- Issue #2181 - Setting small stack size does not change default
- PR #2180 - Changing default bind mode to balanced
- PR #2179 - adding prefetching_iterator and related tests used for prefetching containers within lambda functions
- PR #2177 - Fixing 2176
- Issue #2176 - Launch process test fails on OSX
- PR #2175 - Fix unbalanced config/warnings includes, add some new ones
- PR #2174 - Fix test categorization : regression not unit
- Issue #2172 - Different performance results
- Issue #2171 - “negative entry in reference count table” running octotiger on 32 nodes on queenbee
- Issue #2170 - Error while compiling on Mac + boost 1.60
- PR #2168 - Fixing problems with is_bitwise_serializable
- Issue #2167 - startup & shutdown function should accept unique_function
- Issue #2166 - Simple receive_buffer example
- PR #2165 - Fix wait all
- PR #2164 - Fix wait all
- PR #2163 - Fix some typos in config tests
- PR #2162 - Improve #includes
- PR #2160 - Add inspect check for missing #include <list>
- PR #2159 - Add missing finalize call to stop test hanging
- PR #2158 - Algo fixes
- PR #2157 - Stack check
- Issue #2156 - OSX reports stack space incorrectly (generic context coroutines)
- Issue #2155 - Race condition suspected in runtime
- PR #2154 - Replace boost::detail::atomic_count with the new util::atomic_count
- PR #2153 - Fix stack overflow on OSX
- PR #2152 - Define is_bitwise_serializable as is_trivially_copyable when available
- PR #2151 - Adding missing <cstring> for std::mem* functions
- Issue #2150 - Unable to use component clients as action return types
- PR #2149 - std::memmove copies bytes, use bytes*sizeof(type) when copying larger types
- PR #2146 - Adding customization point for parallel copy/move
- PR #2145 - Applying changes to address warnings issued by latest version of PVS Studio
- Issue #2148 - hpx::parallel::copy is broken after trivially copyable changes
- PR #2144 - Some minor tweaks to compute prototype
- PR #2143 - Added Boost version support information over OSX platform
- PR #2142 - Fixing memory leak in example
- PR #2141 - Add missing specializations in execution policies
- PR #2139 - This PR fixes a few problems reported by Clang’s Undefined Behavior sanitizer
- PR #2138 - Revert “Adding fedora docs”
- PR #2136 - Removed double semicolon
- PR #2135 - Add deprecated #include check for hpx_fwd.hpp
- PR #2134 - Resolved memory leak in stencil_8
- PR #2133 - Replace uses of boost pointer containers
- PR #2132 - Removing unused typedef
- PR #2131 - Add several include checks for std facilities
- PR #2130 - Fixing parcel compression, adding test
- PR #2129 - Fix invalid attribute warnings
- Issue #2128 - hpx::init seems to segfault
- PR #2127 - Making executor_traits N-nary
- PR #2126 - GCC 4.6 fails to deduce the correct type in lambda
- PR #2125 - Making parcel coalescing test actually test something
- Issue #2124 - Make a testcase for parcel compression
- Issue #2123 - hpx/hpx/runtime/applier_fwd.hpp - Multiple defined types
- Issue #2122 - Exception in primary_namespace::resolve_free_list
- Issue #2121 - Possible memory leak in 1d_stencil_8
- PR #2120 - Fixing 2119
- Issue #2119 - reduce_by_key compilation problems
- Issue #2118 - Premature unwrapping of boost::ref’ed arguments
- PR #2117 - Added missing initializer on last constructor for thread_description
- PR #2116 - Use a lightweight bind implementation when no placeholders are given
- PR #2115 - Replace boost::shared_ptr with std::shared_ptr
- PR #2114 - Adding hook functions for executor_parameter_traits supporting timers
- Issue #2113 - Compilation error with gcc version 4.9.3 (MacPorts gcc49 4.9.3_0)
- PR #2112 - Replace uses of safe_bool with explicit operator bool
- Issue #2111 - Compilation error on QT example
- Issue #2110 - Compilation error when passing non-future argument to unwrapped continuation in dataflow
- Issue #2109 - Warning while compiling hpx
- Issue #2109 - Stack trace of last bug causing issues with octotiger
- Issue #2108 - Stack trace of last bug causing issues with octotiger
- PR #2107 - Making sure that a missing parcel_coalescing module does not cause startup exceptions
- PR #2106 - Stop using hpx_fwd.hpp
- Issue #2105 - coalescing plugin handler is not optional any more
- Issue #2104 - Make executor_traits N-nary
- Issue #2103 - Build error with octotiger and hpx commit e657426d
- PR #2102 - Combining thread data storage
- PR #2101 - Added repartition version of 1d stencil that uses any performance counter
- PR #2100 - Drop obsolete TR1 result_of protocol
- PR #2099 - Replace uses of boost::bind with util::bind
- PR #2098 - Deprecated inspect checks
- PR #2097 - Reduce by key, extends #1141
- PR #2096 - Moving local cache from external to hpx/util
- PR #2095 - Bump minimum required Boost to 1.50.0
- PR #2094 - Add include checks for several Boost utilities
- Issue #2093 - /…/local_cache.hpp(89): error #303: explicit type is missing (“int” assumed)
- PR #2091 - Fix for Raspberry pi build
- PR #2090 - Fix storage size for util::function<>
- PR #2089 - Fix #2088
- Issue #2088 - More verbose output from cmake configuration
- PR #2087 - Making sure init_globally always executes hpx_main
- Issue #2086 - Race condition with recent HPX
- PR #2085 - Adding #include checker
- PR #2084 - Replace boost lock types with standard library ones
- PR #2083 - Simplify packaged task
- PR #2082 - Updating APEX version for testing
- PR #2081 - Cleanup exception headers
- PR #2080 - Make call_once variadic
- Issue #2079 - With GNU C++, line 85 of hpx/config/version.hpp causes link failure when linking application
- Issue #2078 - Simple test fails with _GLIBCXX_DEBUG defined
- PR #2077 - Instantiate board in nqueen client
- PR #2076 - Moving coalescing registration to TUs
- PR #2075 - Fixed some documentation typos
- PR #2074 - Adding flush-mode to message handler flush
- PR #2073 - Fixing performance regression introduced lately
- PR #2072 - Refactor local::condition_variable
- PR #2071 - Timer based on boost::asio::deadline_timer
- PR #2070 - Refactor tuple based functionality
- PR #2069 - Fixed typos
- Issue #2068 - Seg fault with octotiger
- PR #2067 - Algorithm cleanup
- PR #2066 - Split credit fixes
- PR #2065 - Rename HPX_MOVABLE_BUT_NOT_COPYABLE to HPX_MOVABLE_ONLY
- PR #2064 - Fixed some typos in docs
- PR #2063 - Adding example demonstrating template components
- Issue #2062 - Support component templates
- PR #2061 - Replace some uses of lexical_cast<string> with C++11 std::to_string
- PR #2060 - Replace uses of boost::noncopyable with HPX_NON_COPYABLE
- PR #2059 - Adding missing for_loop algorithms
- PR #2058 - Move several definitions to more appropriate headers
- PR #2057 - Simplify assert_owns_lock and ignore_while_checking
- PR #2056 - Replacing std::result_of with util::result_of
- PR #2055 - Fix process launching/connecting back
- PR #2054 - Add a forwarding coroutine header
- PR #2053 - Replace uses of boost::unordered_map with std::unordered_map
- PR #2052 - Rewrite tuple unwrap
- PR #2050 - Replace uses of BOOST_SCOPED_ENUM with C++11 scoped enums
- PR #2049 - Attempt to narrow down split_credit problem
- PR #2048 - Fixing gcc startup hangs
- PR #2047 - Fixing when_xxx and wait_xxx for MSVC12
- PR #2046 - adding persistent_auto_chunk_size and related tests for for_each
- PR #2045 - Fixing HPX_HAVE_THREAD_BACKTRACE_DEPTH build time configuration
- PR #2044 - Adding missing service executor types
- PR #2043 - Removing ambiguous definitions for is_future_range and future_range_traits
- PR #2042 - Clarify that HPX builds can use (much) more than 2GB per process
- PR #2041 - Changing future_iterator_traits to support pointers
- Issue #2040 - Improve documentation memory usage warning?
- PR #2039 - Coroutine cleanup
- PR #2038 - Fix cmake policy CMP0042 warning MACOSX_RPATH
- PR #2037 - Avoid redundant specialization of [unique_]function_nonser
- PR #2036 - nvcc dies with an internal error upon pushing/popping warnings inside templates
- Issue #2035 - Use a less restrictive iterator definition in hpx::lcos::detail::future_iterator_traits
- PR #2034 - Fixing compilation error with thread queue wait time performance counter
- Issue #2033 - Compilation error when compiling with thread queue waittime performance counter
- Issue #2032 - Ambiguous template instantiation for is_future_range and future_range_traits.
- PR #2031 - Don’t restart timer on every incoming parcel
- PR #2030 - Unify handling of execution policies in parallel algorithms
- PR #2029 - Make pkg-config .pc files use .dylib on OSX
- PR #2028 - Adding process component
- PR #2027 - Making check for compiler compatibility independent on compiler path
- PR #2025 - Fixing inspect tool
- PR #2024 - Intel13 removal
- PR #2023 - Fix errors related to older boost versions and parameter pack expansions in lambdas
- Issue #2022 - gmake fail: “No rule to make target /usr/lib46/libboost_context-mt.so”
- PR #2021 - Added Sudoku example
- Issue #2020 - Make errors related to init_globally.cpp example while building HPX out of the box
- PR #2019 - Fixed some compilation and cmake errors encountered in nqueen example
- PR #2018 - For loop algorithms
- PR #2017 - Non-recursive at_index implementation
- Issue #2016 - Add index-based for-loops
- Issue #2015 - Change default bind-mode to balanced
- PR #2014 - Fixed dataflow if invoked action returns a future
- PR #2013 - Fixing compilation issues with external example
- PR #2012 - Added Sierpinski Triangle example
- Issue #2011 - Compilation error while running sample hello_world_component code
- PR #2010 - Segmented move implemented for hpx::vector
- Issue #2009 - pkg-config order incorrect on 14.04 / GCC 4.8
- Issue #2008 - Compilation error in dataflow of action returning a future
- PR #2007 - Adding new performance counter exposing overall scheduler time
- PR #2006 - Function includes
- PR #2005 - Adding an example demonstrating how to initialize HPX from a global object
- PR #2004 - Fixing 2000
- PR #2003 - Adding generation parameter to gather to enable using it more than once
- PR #2002 - Turn on position independent code to solve link problem with hpx_init
- Issue #2001 - Gathering more than once segfaults
- Issue #2000 - Undefined reference to hpx::assertion_failed
- Issue #1999 - Seg fault in hpx::lcos::base_lco_with_value<*>::set_value_nonvirt() when running octo-tiger
- PR #1998 - Detect unknown command line options
- PR #1997 - Extending thread description
- PR #1996 - Adding natvis files to solution (MSVC only)
- Issue #1995 - Command line handling does not produce error
- PR #1994 - Possible missing include in test_utils.hpp
- PR #1993 - Add missing LANGUAGES tag to a hpx_add_compile_flag_if_available() call in CMakeLists.txt
- PR #1992 - Fixing shared_executor_test
- PR #1991 - Making sure the winsock library is properly initialized
- PR #1990 - Fixing bind_test placeholder ambiguity coming from boost-1.60
- PR #1989 - Performance tuning
- PR #1987 - Make configurable size of internal storage in util::function
- PR #1986 - AGAS Refactoring+1753 Cache mods
- PR #1985 - Adding missing task_block::run() overload taking an executor
- PR #1984 - Adding an optimized LRU Cache implementation (for AGAS)
- PR #1983 - Avoid invoking migration table look up for all objects
- PR #1981 - Replacing uintptr_t (which is not defined everywhere) with std::size_t
- PR #1980 - Optimizing LCO continuations
- PR #1979 - Fixing Cori
- PR #1978 - Fix test check that got broken in hasty fix to memory overflow
- PR #1977 - Refactor action traits
- PR #1976 - Fixes typo in README.rst
- PR #1975 - Reduce size of benchmark timing arrays to fix test failures
- PR #1974 - Add action to update data owned by the partitioned_vector component
- PR #1972 - Adding partitioned_vector SPMD example
- PR #1971 - Fixing 1965
- PR #1970 - Papi fixes
- PR #1969 - Fixing continuation recursions to not depend on fixed amount of recursions
- PR #1968 - More segmented algorithms
- Issue #1967 - Simplify component implementations
- PR #1966 - Migrate components
- Issue #1964 - fatal error: ‘boost/lockfree/detail/branch_hints.hpp’ file not found
- Issue #1962 - parallel:copy_if has race condition when used on in place arrays
- PR #1963 - Fixing Static Parcelport initialization
- PR #1961 - Fix function target
- Issue #1960 - Papi counters don’t reset
- PR #1959 - Fixing 1958
- Issue #1958 - inclusive_scan gives incorrect results with non-commutative operator
- PR #1957 - Fixing #1950
- PR #1956 - Sort by key example
- PR #1955 - Adding regression test for #1946: Hang in wait_all() in distributed run
- Issue #1954 - HPX releases should not use -Werror
- PR #1953 - Adding performance analysis for AGAS cache
- PR #1952 - Adapting test for explicit variadics to fail for gcc 4.6
- PR #1951 - Fixing memory leak
- Issue #1950 - Simplify external builds
- PR #1949 - Fixing yet another lock that is being held during suspension
- PR #1948 - Fixed container algorithms for Intel
- PR #1947 - Adding workaround for tagged_tuple
- Issue #1946 - Hang in wait_all() in distributed run
- PR #1945 - Fixed container algorithm tests
- Issue #1944 - assertion ‘p.destination_locality() == hpx::get_locality()’ failed
- PR #1943 - Fix a couple of compile errors with clang
- PR #1942 - Making parcel coalescing functional
- Issue #1941 - Re-enable parcel coalescing
- PR #1940 - Touching up make_future
- PR #1939 - Fixing problems in over-subscription management in the resource manager
- PR #1938 - Removing use of unified Boost.Thread header
- PR #1937 - Cleaning up the use of Boost.Accumulator headers
- PR #1936 - Making sure interval timer is started for aggregating performance counters
- PR #1935 - Tagged results
- PR #1934 - Fix remote async with deferred launch policy
- Issue #1933 - Floating point exception in
statistics_counter<boost::accumulators::tag::mean>::get_counter_value
- PR #1932 - Removing superfluous includes of boost/lockfree/detail/branch_hints.hpp
- PR #1931 - fix compilation with clang 3.8.0
- Issue #1930 - Missing online documentation for HPX 0.9.11
- PR #1929 - LWG2485: get() should be overloaded for const tuple&&
- PR #1928 - Revert “Using ninja for circle-ci builds”
- PR #1927 - Using ninja for circle-ci builds
- PR #1926 - Fixing serialization of std::array
- Issue #1925 - Issues with static HPX libraries
- Issue #1924 - Peformance degrading over time
- Issue #1923 - serialization of std::array appears broken in latest commit
- PR #1922 - Container algorithms
- PR #1921 - Tons of smaller quality improvements
- Issue #1920 - Seg fault in hpx::serialization::output_archive::add_gid when running octotiger
- Issue #1919 - Intel 15 compiler bug preventing HPX build
- PR #1918 - Address sanitizer fixes
- PR #1917 - Fixing compilation problems of parallel::sort with Intel compilers
- PR #1916 - Making sure code compiles if HPX_WITH_HWLOC=Off
- Issue #1915 - max_cores undefined if HPX_WITH_HWLOC=Off
- PR #1913 - Add utility member functions for partitioned_vector
- PR #1912 - Adding support for invoking actions to dataflow
- PR #1911 - Adding first batch of container algorithms
- PR #1910 - Keep cmake_module_path
- PR #1909 - Fix mpirun with pbs
- PR #1908 - Changing parallel::sort to return the last iterator as proposed by N4560
- PR #1907 - Adding a minimum version for Open MPI
- PR #1906 - Updates to the Release Procedure
- PR #1905 - Fixing #1903
- PR #1904 - Making sure std containers are cleared before serialization loads data
- Issue #1903 - When running octotiger, I get: assertion
'(*new_gids_)[gid].size() == 1' failed: HPX(assertion_failure)
- Issue #1902 - Immediate crash when running hpx/octotiger with _GLIBCXX_DEBUG defined.
- PR #1901 - Making non-serializable classes non-serializable
- Issue #1900 - Two possible issues with std::list serialization
- PR #1899 - Fixing a problem with credit splitting as revealed by #1898
- Issue #1898 - Accessing component from locality where it was not created segfaults
- PR #1897 - Changing parallel::sort to return the last iterator as proposed by N4560
- Issue #1896 - version 1.0?
- Issue #1895 - Warning comment on numa_allocator is not very clear
- PR #1894 - Add support for compilers that have thread_local
- PR #1893 - Fixing 1890
- PR #1892 - Adds typed future_type for executor_traits
- PR #1891 - Fix wording in certain parallel algorithm docs
- Issue #1890 - Invoking papi counters give segfault
- PR #1889 - Fixing problems as reported by clang-check
- PR #1888 - WIP parallel is_heap
- PR #1887 - Fixed resetting performance counters related to idle-rate, etc
- Issue #1886 - Run hpx with qsub does not work
- PR #1885 - Warning cleaning pass
- PR #1884 - Add missing parallel algorithm header
- PR #1883 - Add feature test for thread_local on Clang for TLS
- PR #1882 - Fix some redundant qualifiers
- Issue #1881 - Unable to compile Octotiger using HPX and Intel MPI on SuperMIC
- Issue #1880 - clang with libc++ on Linux needs TLS case
- PR #1879 - Doc fixes for #1868
- PR #1878 - Simplify functions
- PR #1877 - Removing most usage of Boost.Config
- PR #1876 - Add missing parallel algorithms to algorithm.hpp
- PR #1875 - Simplify callables
- PR #1874 - Address long standing FIXME on using
std::unique_ptr
with incomplete types - PR #1873 - Fixing 1871
- PR #1872 - Making sure PBS environment uses specified node list even if no PBS_NODEFILE env is available
- Issue #1871 - Fortran checks should be optional
- PR #1870 - Touch local::mutex
- PR #1869 - Documentation refactoring based off #1868
- PR #1867 - Embrace static_assert
- PR #1866 - Fix #1803 with documentation refactoring
- PR #1865 - Setting OUTPUT_NAME as target properties
- PR #1863 - Use SYSTEM for boost includes
- PR #1862 - Minor cleanups
- PR #1861 - Minor Corrections for Release
- PR #1860 - Fixing hpx gdb script
- Issue #1859 - reset_active_counters resets times and thread counts before some of the counters are evaluated
- PR #1858 - Release V0.9.11
- PR #1857 - removing diskperf example from 9.11 release
- PR #1856 - fix return in packaged_task_base::reset()
- Issue #1842 - Install error: file INSTALL cannot find libhpx_parcel_coalescing.so.0.9.11
- PR #1839 - Adding fedora docs
- PR #1824 - Changing version on master to V0.9.12
- PR #1818 - Fixing #1748
- Issue #1815 - seg fault in AGAS
- Issue #1803 - wait_all documentation
- Issue #1796 - Outdated documentation to be revised
- Issue #1759 - glibc munmap_chunk or free(): invalid pointer on SuperMIC
- Issue #1753 - HPX performance degrades with time since execution begins
- Issue #1748 - All public HPX headers need to be self contained
- PR #1719 - How to build HPX with Visual Studio
- Issue #1684 - Race condition when using –hpx:connect?
- PR #1658 - Add serialization for std::set (as there is for std::vector and std::map)
- PR #1641 - Generic client
- Issue #1632 - heartbeat example fails on separate nodes
- PR #1603 - Adds preferred namespace check to inspect tool
- Issue #1559 - Extend inspect tool
- Issue #1523 - Remote async with deferred launch policy never executes
- Issue #1472 - Serialization issues
- Issue #1457 - Implement N4392: C++ Latches and Barriers
- PR #1444 - Enabling usage of moveonly types for component construction
- Issue #1407 - The Intel 13 compiler has failing unit tests
- Issue #1405 - Allow component constructors to take movable only types
- Issue #1265 - Enable dataflow() to be usable with actions
- Issue #1236 - NUMA aware allocators
- Issue #802 - Fix Broken Examples
- Issue #559 - Add hpx::migrate facility
- Issue #449 - Make actions with template arguments usable and add documentation
- Issue #279 - Refactor addressing_service into a base class and two derived classes
- Issue #224 - Changing thread state metadata is not thread safe
- Issue #55 - Uniform syntax for enums should be implemented
HPX V0.9.11 (Nov 11, 2015)¶
Our main focus for this release was the design and development of a coherent set
of higher-level APIs exposing various types of parallelism to the application
programmer. We introduced the concepts of an executor
, which can be used to
customize the where
and when
of execution of tasks in the context of
parallelizing codes. We extended all APIs related to managing parallel tasks to
support executors which gives the user the choce of either using one of the
predefined executor types or to provide its own, possibly application specific,
executor. We paid very close attention to align all of these changes with the
existing C++ Standards documents or with the ongoing proposals for
standardization.
This release is the first after our change to a new development policy. We
switched all development to be strictly performed on branches only, all direct
commits to our main branch (master
) are prohibited. Any change has to go
through a peer review before it will be merged to master
. As a result the
overall stability of our code base has significantly increased, the development
process itself has been simplified. This change manifests itself in a large
number of pull-requests which have been merged (please see below for a full list
of closed issues and pull-requests). All in all for this release, we closed
almost 100 issues and merged over 290 pull-requests. There have been over 1600
commits to the master branch since the last release.
General changes¶
- We are moving into the direction of unifying managed and simple components. As
such, the classes
hpx::components::component
andhpx::components::component_base
have been added which currently just forward to the currently existing simple component facilities. The examples have been converted to only use those two classes. - Added integration with the CircleCI hosted continuous integration service. This gives us constant and immediate feedback on the health of our master branch.
- The compiler configuration subsystem in the build system has been reimplemented. Instead of using Boost.Config we now use our own lightweight set of cmake scripts to determine the available language and library features supported by the used compiler.
- The API for creating instances of components has been consolidated. All
component instances should be created using the
hpx::new_
only. It allows to instantiate both, single component instances and multiple component instances. The placement of the created components can be controlled by special distribution policies. Please see the corresponding documentation outlining the use ofhpx::new_
. - Introduced four new distribution policies which can be used with many API
functions which traditionally expected to be used with a locality id. The new
distribution policies are:
hpx::components::default_distribution_policy
which tries to place multiple component instances as evenly as possible.hpx::components::colocating_distribution_policy
which will refer to the locality where a given component instance is currently placed.hpx::components::binpacking_distribution_policy
which will place multiple component instances as evenly as possible based on any performance counter.hpx::components::target_distribution_policy
which allows to represent a given locality in the context of a distrwibution policy.
- The new distribution policies can now be also used with
hpx::async
. This change also deprecateshpx::async_colocated(id, ...)
which now is replaced by a distribution policy:hpx::async(hpx::colocated(id), ...)
. - The
hpx::vector
andhpx::unordered_map
data structures can now be used with the new distribution policies as well. - The parallel facility
hpx::parallel::task_region
has been renamed tohpx::parallel::task_block
based on the changes in the corresponding standardization proposal N4411. - Added extensions to the parallel facility
hpx::parallel::task_block
allowing to combine a task_block with an execution policy. This implies a minor breaking change as thehpx::parallel::task_block
is now a template. - Added new LCOs:
hpx::lcos::latch
andhpx::lcos::local::latch
which semantically conform to the proposedstd::latch
(see N4399). - Added performance counters exposing data related to data transferred by input/output (filesystem) operations (thanks to Maciej Brodowicz).
- Added performance counters allowing to track the number of action invocations (local and remote invocations).
- Added new command line options –hpx:print-counter-at and –hpx:reset-counters.
- The
hpx::vector
component has been renamed tohpx::partitioned_vector
to make it explicit that the underlying memory is not contiguous. - Introduced a completely new and uniform higher-level parallelism API which is based on executors. All existing parallelism APIs have been adapted to this. We have added a large number of different executor types, such as a numa-aware executor, a this-thread executor, etc.
- Added support for the MingW toolchain on Windows (thanks to Eric Lemanissier).
- HPX now includes support for APEX, (Autonomic Performance Environment for
eXascale). APEX is an instrumentation and software adaptation library that
provides an interface to TAU profiling / tracing as well as runtime adaptation
of HPX applications through policy definitions. For more information and
documentation, please see https://github.com/khuck/xpress-apex. To enable APEX
at configuration time, specify
-DHPX_WITH_APEX=On
. To also include support for TAU profiling, specify-DHPX_WITH_TAU=On
and specify the-DTAU_ROOT
,-DTAU_ARCH
and-DTAU_OPTIONS
cmake parameters. - We have implemented many more of the Using parallel algorithms. Please see Issue #1141 for the list of all available parallel algorithms (thanks to Daniel Bourgeois and John Biddiscombe for contributing their work).
Breaking changes¶
- We are moving into the direction of unifying managed and simple components. In
order to stop exposing the old facilities, all examples have been converted to
use the new classes. The breaking change in this release is that performance
counters are now a
hpx::components::component_base
instead ofhpx::components::managed_component_base
. - We removed the support for stackless threads. It turned out that there was no performance benefit when using stackless threads. As such, we decided to clean up our codebase. This feature was not documented.
- The CMake project name has changed from ‘hpx’ to ‘HPX’ for consistency and compatibilty with naming conventions and other CMake projects. Generated config files go into <prefix>/lib/cmake/HPX and not <prefix>/lib/cmake/hpx.
- The macro
HPX_REGISTER_MINIMAL_COMPONENT_FACTORY
has been deprecated. Please useHPX_REGISTER_COMPONENT
. instead. The old macro will be removed in the next release. - The obsolete distributing_factory and binpacking_factory components have been
removed. The corresponding functionality is now provided by the
hpx::new_
API function in conjunction with thehpx::default_layout
andhpx::binpacking
distribution policies (hpx::components::default_distribution_policy
andhpx::components::binpacking_distribution_policy
) - The API function
hpx::new_colocated
has been deprecated. Please use the consolidated APIhpx::new_
in conjunction with the newhpx::colocated
distribution policy (hpx::components::colocating_distribution_policy
) instead. The old API function will still be available for at least one release of HPX if the configuration variableHPX_WITH_COLOCATED_BACKWARDS_COMPATIBILITY
is enabled. - The API function
hpx::async_colocated
has been deprecated. Please use the consolidated APIhpx::async
in conjunction with the newhpx::colocated
distribution policy (hpx::components::colocating_distribution_policy
) instead. The old API function will still be available for at least one release of HPX if the configuration variableHPX_WITH_COLOCATED_BACKWARDS_COMPATIBILITY
is enabled. - The obsolete remote_object component has been removed.
- Replaced the use of Boost.Serialization with our own solution. While the new version is mostly compatible with Boost.Serialization, this change requires some minor code modifications in user code. For more information, please see the corresponding announcement on the hpx-users@stellar.cct.lsu.edu mailing list.
- The names used by cmake to influence various configuration options have been
unified. The new naming scheme relies on all configuration constants to start
with
HPX_WITH_...
, while the preprocessor constant which is used at build time starts withHPX_HAVE_...
. For instance, the former cmake command line-DHPX_MALLOC=...
now has to be specified a-DHPX_WITH_MALLOC=...
and will cause the preprocessor constantHPX_HAVE_MALLOC
to be defined. The actual name of the constant (i.e.MALLOC
) has not changed. Please see the corresponding documentation for more details (CMake variables used to configure HPX). - The
get_gid()
functions exposed by the component base classeshpx::components::server::simple_component_base
,hpx::components::server::managed_component_base
, andhpx::components::server::fixed_component_base
have been replaced by two new functions:get_unmanaged_id()
andget_id()
. To enable the old function name for backwards compatibility, use the cmake configuration optionHPX_WITH_COMPONENT_GET_GID_COMPATIBILITY=On
. - All functions which were named
get_gid()
but were returninghpx::id_type
have been renamed toget_id()
. To enable the old function names for backwards compatibility, use the cmake configuration optionHPX_WITH_COMPONENT_GET_GID_COMPATIBILITY=On
.
Bug fixes (closed tickets)¶
Here is a list of the important tickets we closed for this release.
- PR #1855 - Completely removing external/endian
- PR #1854 - Don’t pollute CMAKE_CXX_FLAGS through find_package()
- PR #1853 - Updating CMake configuration to get correct version of TAU library
- PR #1852 - Fixing Performance Problems with MPI Parcelport
- PR #1851 - Fixing hpx_add_link_flag() and hpx_remove_link_flag()
- PR #1850 - Fixing 1836, adding parallel::sort
- PR #1849 - Fixing configuration for use of more than 64 cores
- PR #1848 - Change default APEX version for release
- PR #1847 - Fix client_base::then on release
- PR #1846 - Removing broken lcos::local::channel from release
- PR #1845 - Adding example demonstrating a possible safe-object implementation to release
- PR #1844 - Removing stubs from accumulator examples
- PR #1843 - Don’t pollute CMAKE_CXX_FLAGS through find_package()
- PR #1841 - Fixing client_base<>::then
- PR #1840 - Adding example demonstrating a possible safe-object implementation
- PR #1838 - Update version rc1
- PR #1837 - Removing broken lcos::local::channel
- PR #1835 - Adding exlicit move constructor and assignment operator to hpx::lcos::promise
- PR #1834 - Making hpx::lcos::promise move-only
- PR #1833 - Adding fedora docs
- Issue #1832 - hpx::lcos::promise<> must be move-only
- PR #1831 - Fixing resource manager gcc5.2
- PR #1830 - Fix intel13
- PR #1829 - Unbreaking thread test
- PR #1828 - Fixing #1620
- PR #1827 - Fixing a memory management issue for the Parquet application
- Issue #1826 - Memory management issue in hpx::lcos::promise
- PR #1825 - Adding hpx::components::component and hpx::components::component_base
- PR #1823 - Adding git commit id to circleci build
- PR #1822 - applying fixes suggested by clang 3.7
- PR #1821 - Hyperlink fixes
- PR #1820 - added parallel multi-locality sanity test
- PR #1819 - Fixing #1667
- Issue #1817 - Hyperlinks generated by inspect tool are wrong
- PR #1816 - Support hpxrx
- PR #1814 - Fix async to dispatch to the correct locality in all cases
- Issue #1813 - async(launch::…, action(), …) always invokes locally
- PR #1812 - fixed syntax error in CMakeLists.txt
- PR #1811 - Agas optimizations
- PR #1810 - drop superfluous typedefs
- PR #1809 - Allow HPX to be used as an optional package in 3rd party code
- PR #1808 - Fixing #1723
- PR #1807 - Making sure resolve_localities does not hang during normal operation
- Issue #1806 - Spinlock no longer movable and deletes operator ‘=’, breaks MiniGhost
- Issue #1804 - register_with_basename causes hangs
- PR #1801 - Enhanced the inspect tool to take user directly to the problem with hyperlinks
- Issue #1800 - Problems compiling application on smic
- PR #1799 - Fixing cv exceptions
- PR #1798 - Documentation refactoring & updating
- PR #1797 - Updating the activeharmony CMake module
- PR #1795 - Fixing cv
- PR #1794 - Fix connect with hpx::runtime_mode_connect
- PR #1793 - fix a wrong use of HPX_MAX_CPU_COUNT instead of HPX_HAVE_MAX_CPU_COUNT
- PR #1792 - Allow for default constructed parcel instances to be moved
- PR #1791 - Fix connect with hpx::runtime_mode_connect
- Issue #1790 - assertion
action_.get()
failed: HPX(assertion_failure) when running Octotiger with pull request 1786 - PR #1789 - Fixing discover_counter_types API function
- Issue #1788 - connect with hpx::runtime_mode_connect
- Issue #1787 - discover_counter_types not working
- PR #1786 - Changing addressing_service to use std::unordered_map instead of std::map
- PR #1785 - Fix is_iterator for container algorithms
- PR #1784 - Adding new command line options:
- PR #1783 - Minor changes for APEX support
- PR #1782 - Drop legacy forwarding action traits
- PR #1781 - Attempt to resolve the race between cv::wait_xxx and cv::notify_all
- PR #1780 - Removing serialize_sequence
- PR #1779 - Fixed #1501: hwloc configuration options are wrong for MIC
- PR #1778 - Removing ability to enable/disable parcel handling
- PR #1777 - Completely removing stackless threads
- PR #1776 - Cleaning up util/plugin
- PR #1775 - Agas fixes
- PR #1774 - Action invocation count
- PR #1773 - replaced MSVC variable with WIN32
- PR #1772 - Fixing Problems in MPI parcelport and future serialization.
- PR #1771 - Fixing intel 13 compiler errors related to variadic template
template parameters for
lcos::when_
tests - PR #1770 - Forwarding decay to
std::
- PR #1769 - Add more characters with special regex meaning to the existing patch
- PR #1768 - Adding test for receive_buffer
- PR #1767 - Making sure that uptime counter throws exception on any attempt to be reset
- PR #1766 - Cleaning up code related to throttling scheduler
- PR #1765 - Restricting thread_data to creating only with intrusive_pointers
- PR #1764 - Fixing 1763
- Issue #1763 - UB in thread_data::operator delete
- PR #1762 - Making sure all serialization registries/factories are unique
- PR #1761 - Fixed #1751: hpx::future::wait_for fails a simple test
- PR #1758 - Fixing #1757
- Issue #1757 - pinning not correct using –hpx:bind
- Issue #1756 - compilation error with MinGW
- PR #1755 - Making output serialization const-correct
- Issue #1753 - HPX performance degrades with time since execution begins
- Issue #1752 - Error in AGAS
- Issue #1751 - hpx::future::wait_for fails a simple test
- PR #1750 - Removing hpx_fwd.hpp includes
- PR #1749 - Simplify result_of and friends
- PR #1747 - Removed superfluous code from message_buffer.hpp
- PR #1746 - Tuple dependencies
- Issue #1745 - Broken when_some which takes iterators
- PR #1744 - Refining archive interface
- PR #1743 - Fixing when_all when only a single future is passed
- PR #1742 - Config includes
- PR #1741 - Os executors
- Issue #1740 - hpx::promise has some problems
- PR #1739 - Parallel composition with generic containers
- Issue #1738 - After building program and successfully linking to a version of hpx DHPX_DIR seems to be ignored
- Issue #1737 - Uptime problems
- PR #1736 - added convenience c-tor and begin()/end() to serialize_buffer
- PR #1735 - Config includes
- PR #1734 - Fixed #1688: Add timer counters for tfunc_total and exec_total
- Issue #1733 - Add unit test for hpx/lcos/local/receive_buffer.hpp
- PR #1732 - Renaming get_os_thread_count
- PR #1731 - Basename registration
- Issue #1730 - Use after move of thread_init_data
- PR #1729 - Rewriting channel based on new gate component
- PR #1728 - Fixing #1722
- PR #1727 - Fixing compile problems with apply_colocated
- PR #1726 - Apex integration
- PR #1725 - fixed test timeouts
- PR #1724 - Renaming vector
- Issue #1723 - Drop support for intel compilers and gcc 4.4. based standard libs
- Issue #1722 - Add support for detecting non-ready futures before serialization
- PR #1721 - Unifying parallel executors, initializing from launch policy
- PR #1720 - dropped superfluous typedef
- Issue #1718 - Windows 10 x64, VS 2015 - Unknown CMake command “add_hpx_pseudo_target”.
- PR #1717 - Timed executor traits for thread-executors
- PR #1716 - serialization of arrays didn’t work with non-pod types. fixed
- PR #1715 - List serialization
- PR #1714 - changing misspellings
- PR #1713 - Fixed distribution policy executors
- PR #1712 - Moving library detection to be executed after feature tests
- PR #1711 - Simplify parcel
- PR #1710 - Compile only tests
- PR #1709 - Implemented timed executors
- PR #1708 - Implement parallel::executor_traits for thread-executors
- PR #1707 - Various fixes to threads::executors to make custom schedulers work
- PR #1706 - Command line option –hpx:cores does not work as expected
- Issue #1705 - command line option –hpx:cores does not work as expected
- PR #1704 - vector deserialization is speeded up a little
- PR #1703 - Fixing shared_mutes
- Issue #1702 - Shared_mutex does not compile with no_mutex cond_var
- PR #1701 - Add distribution_policy_executor
- PR #1700 - Executor parameters
- PR #1699 - Readers writer lock
- PR #1698 - Remove leftovers
- PR #1697 - Fixing held locks
- PR #1696 - Modified Scan Partitioner for Algorithms
- PR #1695 - This thread executors
- PR #1694 - Fixed #1688: Add timer counters for tfunc_total and exec_total
- PR #1693 - Fix #1691: is_executor template specification fails for inherited executors
- PR #1692 - Fixed #1662: Possible exception source in coalescing_message_handler
- Issue #1691 - is_executor template specification fails for inherited executors
- PR #1690 - added macro for non-intrusive serialization of classes without a default c-tor
- PR #1689 - Replace value_or_error with custom storage, unify future_data state
- Issue #1688 - Add timer counters for tfunc_total and exec_total
- PR #1687 - Fixed interval timer
- PR #1686 - Fixing cmake warnings about not existing pseudo target dependencies
- PR #1685 - Converting partitioners to use bulk async execute
- PR #1683 - Adds a tool for inspect that checks for character limits
- PR #1682 - Change project name to (uppercase) HPX
- PR #1681 - Counter shortnames
- PR #1680 - Extended Non-intrusive Serialization to Ease Usage for Library Developers
- PR #1679 - Working on 1544: More executor changes
- PR #1678 - Transpose fixes
- PR #1677 - Improve Boost compatibility check
- PR #1676 - 1d stencil fix
- Issue #1675 - hpx project name is not HPX
- PR #1674 - Fixing the MPI parcelport
- PR #1673 - added move semantics to map/vector deserialization
- PR #1672 - Vs2015 await
- PR #1671 - Adapt transform for #1668
- PR #1670 - Started to work on #1668
- PR #1669 - Add this_thread_executors
- Issue #1667 - Apple build instructions in docs are out of date
- PR #1666 - Apex integration
- PR #1665 - Fixes an error with the whitespace check that showed the incorrect location of the error
- Issue #1664 - Inspect tool found incorrect endline whitespace
- PR #1663 - Improve use of locks
- Issue #1662 - Possible exception source in coalescing_message_handler
- PR #1661 - Added support for 128bit number serialization
- PR #1660 - Serialization 128bits
- PR #1659 - Implemented inner_product and adjacent_diff algos
- PR #1658 - Add serialization for std::set (as there is for std::vector and std::map)
- PR #1657 - Use of shared_ptr in io_service_pool changed to unique_ptr
- Issue #1656 - 1d_stencil codes all have wrong factor
- PR #1654 - When using runtime_mode_connect, find the correct localhost public ip address
- PR #1653 - Fixing 1617
- PR #1652 - Remove traits::action_may_require_id_splitting
- PR #1651 - Fixed performance counters related to AGAS cache timings
- PR #1650 - Remove leftovers of traits::type_size
- PR #1649 - Shorten target names on Windows to shorten used path names
- PR #1648 - Fixing problems introduced by merging #1623 for older compilers
- PR #1647 - Simplify running automatic builds on Windows
- Issue #1646 - Cache insert and update performance counters are broken
- Issue #1644 - Remove leftovers of traits::type_size
- Issue #1643 - Remove traits::action_may_require_id_splitting
- PR #1642 - Adds spell checker to the inspect tool for qbk and doxygen comments
- PR #1640 - First step towards fixing 688
- PR #1639 - Re-apply remaining changes from limit_dataflow_recursion branch
- PR #1638 - This fixes possible deadlock in the test ignore_while_locked_1485
- PR #1637 - Fixing hpx::wait_all() invoked with two vector<future<T>>
- PR #1636 - Partially re-apply changes from limit_dataflow_recursion branch
- PR #1635 - Adding missing test for #1572
- PR #1634 - Revert “Limit recursion-depth in dataflow to a configurable constant”
- PR #1633 - Add command line option to ignore batch environment
- PR #1631 - hpx::lcos::queue exhibits strange behavior
- PR #1630 - Fixed endline_whitespace_check.cpp to detect lines with only whitespace
- Issue #1629 - Inspect trailing whitespace checker problem
- PR #1628 - Removed meaningless const qualifiers. Minor icpc fix.
- PR #1627 - Fixing the queue LCO and add example demonstrating its use
- PR #1626 - Deprecating get_gid(), add get_id() and get_unmanaged_id()
- PR #1625 - Allowing to specify whether to send credits along with message
- Issue #1624 - Lifetime issue
- Issue #1623 - hpx::wait_all() invoked with two vector<future<T>> fails
- PR #1622 - Executor partitioners
- PR #1621 - Clean up coroutines implementation
- Issue #1620 - Revert #1535
- PR #1619 - Fix result type calculation for hpx::make_continuation
- PR #1618 - Fixing RDTSC on Xeon/Phi
- Issue #1617 - hpx cmake not working when run as a subproject
- Issue #1616 - cmake problem resulting in RDTSC not working correctly for Xeon Phi creates very strange results for duration counters
- Issue #1615 - hpx::make_continuation requires input and output to be the same
- PR #1614 - Fixed remove copy test
- Issue #1613 - Dataflow causes stack overflow
- PR #1612 - Modified foreach partitioner to use bulk execute
- PR #1611 - Limit recursion-depth in dataflow to a configurable constant
- PR #1610 - Increase timeout for CircleCI
- PR #1609 - Refactoring thread manager, mainly extracting thread pool
- PR #1608 - Fixed running multiple localities without localities parameter
- PR #1607 - More algorithm fixes to adjacentfind
- Issue #1606 - Running without localities parameter binds to bogus port range
- Issue #1605 - Too many serializations
- PR #1604 - Changes the HPX image into a hyperlink
- PR #1601 - Fixing problems with remove_copy algorithm tests
- PR #1600 - Actions with ids cleanup
- PR #1599 - Duplicate binding of global ids should fail
- PR #1598 - Fixing array access
- PR #1597 - Improved the reliability of connecting/disconnecting localities
- Issue #1596 - Duplicate id binding should fail
- PR #1595 - Fixing more cmake config constants
- PR #1594 - Fixing preprocessor constant used to enable C++11 chrono
- PR #1593 - Adding operator|() for hpx::launch
- Issue #1592 - Error (typo) in the docs
- Issue #1590 - CMake fails when CMAKE_BINARY_DIR contains ‘+’.
- Issue #1589 - Disconnecting a locality results in segfault using heartbeat example
- PR #1588 - Fix doc string for config option HPX_WITH_EXAMPLES
- PR #1586 - Fixing 1493
- PR #1585 - Additional Check for Inspect Tool to detect Endline Whitespace
- Issue #1584 - Clean up coroutines implementation
- PR #1583 - Adding a check for end line whitespace
- PR #1582 - Attempt to fix assert firing after scheduling loop was exited
- PR #1581 - Fixed adjacentfind_binary test
- PR #1580 - Prevent some of the internal cmake lists from growing indefinitely
- PR #1579 - Removing type_size trait, replacing it with special archive type
- Issue #1578 - Remove demangle_helper
- PR #1577 - Get ptr problems
- Issue #1576 - Refactor async, dataflow, and future::then
- PR #1575 - Fixing tests for parallel rotate
- PR #1574 - Cleaning up schedulers
- PR #1573 - Fixing thread pool executor
- PR #1572 - Fixing number of configured localities
- PR #1571 - Reimplement decay
- PR #1570 - Refactoring async, apply, and dataflow APIs
- PR #1569 - Changed range for mach-o library lookup
- PR #1568 - Mark decltype support as required
- PR #1567 - Removed const from algorithms
- Issue #1566 - CMAKE Configuration Test Failures for clang 3.5 on debian
- PR #1565 - Dylib support
- PR #1564 - Converted partitioners and some algorithms to use executors
- PR #1563 - Fix several #includes for Boost.Preprocessor
- PR #1562 - Adding configuration option disabling/enabling all message handlers
- PR #1561 - Removed all occurrences of boost::move replacing it with std::move
- Issue #1560 - Leftover HPX_REGISTER_ACTION_DECLARATION_2
- PR #1558 - Revisit async/apply SFINAE conditions
- PR #1557 - Removing type_size trait, replacing it with special archive type
- PR #1556 - Executor algorithms
- PR #1555 - Remove the necessity to specify archive flags on the receiving end
- PR #1554 - Removing obsolete Boost.Serialization macros
- PR #1553 - Properly fix HPX_DEFINE_*_ACTION macros
- PR #1552 - Fixed algorithms relying on copy_if implementation
- PR #1551 - Pxfs - Modifying FindOrangeFS.cmake based on OrangeFS 2.9.X
- Issue #1550 - Passing plain identifier inside HPX_DEFINE_PLAIN_ACTION_1
- PR #1549 - Fixing intel14/libstdc++4.4
- PR #1548 - Moving raw_ptr to detail namespace
- PR #1547 - Adding support for executors to future.then
- PR #1546 - Executor traits result types
- PR #1545 - Integrate executors with dataflow
- PR #1543 - Fix potential zero-copy for primarynamespace::bulk_service_async et.al.
- PR #1542 - Merging HPX0.9.10 into pxfs branch
- PR #1541 - Removed stale cmake tests, unused since the great cmake refactoring
- PR #1540 - Fix idle-rate on platforms without TSC
- PR #1539 - Reporting situation if zero-copy-serialization was performed by a parcel generated from a plain apply/async
- PR #1538 - Changed return type of bulk executors and added test
- Issue #1537 - Incorrect cpuid config tests
- PR #1536 - Changed return type of bulk executors and added test
- PR #1535 - Make sure promise::get_gid() can be called more than once
- PR #1534 - Fixed async_callback with bound callback
- PR #1533 - Updated the link in the documentation to a publically- accessible URL
- PR #1532 - Make sure sync primitives are not copyable nor movable
- PR #1531 - Fix unwrapped issue with future ranges of void type
- PR #1530 - Serialization complex
- Issue #1528 - Unwrapped issue with future<void>
- Issue #1527 - HPX does not build with Boost 1.58.0
- PR #1526 - Added support for boost.multi_array serialization
- PR #1525 - Properly handle deferred futures, fixes #1506
- PR #1524 - Making sure invalid action argument types generate clear error message
- Issue #1522 - Need serialization support for boost multi array
- Issue #1521 - Remote async and zero-copy serialization optimizations don’t play well together
- PR #1520 - Fixing UB whil registering polymorphic classes for serialization
- PR #1519 - Making detail::condition_variable safe to use
- PR #1518 - Fix when_some bug missing indices in its result
- Issue #1517 - Typo may affect CMake build system tests
- PR #1516 - Fixing Posix context
- PR #1515 - Fixing Posix context
- PR #1514 - Correct problems with loading dynamic components
- PR #1513 - Fixing intel glibc4 4
- Issue #1508 - memory and papi counters do not work
- Issue #1507 - Unrecognized Command Line Option Error causing exit status 0
- Issue #1506 - Properly handle deferred futures
- PR #1505 - Adding #include - would not compile without this
- Issue #1502 -
boost::filesystem::exists
throws unexpected exception - Issue #1501 - hwloc configuration options are wrong for MIC
- PR #1504 - Making sure boost::filesystem::exists() does not throw
- PR #1500 - Exit application on
--hpx:version
/-v
and--hpx:info
- PR #1498 - Extended task block
- PR #1497 - Unique ptr serialization
- PR #1496 - Unique ptr serialization (closed)
- PR #1495 - Switching circleci build type to debug
- Issue #1494 -
--hpx:version
/-v
does not exit after printing version information - Issue #1493 - add an
hpx_
prefix to libraries and components to avoid name conflicts - Issue #1492 - Define and ensure limitations for arguments to async/apply
- PR #1489 - Enable idle rate counter on demand
- PR #1488 - Made sure
detail::condition_variable
can be safely destroyed - PR #1487 - Introduced default (main) template implementation for
ignore_while_checking
- PR #1486 - Add HPX inspect tool
- Issue #1485 -
ignore_while_locked
doesn’t support all Lockable types - PR #1484 - Docker image generation
- PR #1483 - Move external endian library into HPX
- PR #1482 - Actions with integer type ids
- Issue #1481 - Sync primitives safe destruction
- Issue #1480 - Move external/boost/endian into hpx/util
- Issue #1478 - Boost inspect violations
- PR #1479 - Adds serialization for arrays; some futher/minor fixes
- PR #1477 - Fixing problems with the Intel compiler using a GCC 4.4 std library
- PR #1476 - Adding
hpx::lcos::latch
andhpx::lcos::local::latch
- Issue #1475 - Boost inspect violations
- PR #1473 - Fixing action move tests
- Issue #1471 - Sync primitives should not be movable
- PR #1470 - Removing
hpx::util::polymorphic_factory
- PR #1468 - Fixed container creation
- Issue #1467 - HPX application fail during finalization
- Issue #1466 - HPX doesn’t pick up Torque’s nodefile on SuperMIC
- Issue #1464 - HPX option for pre and post bootstrap performance counters
- PR #1463 - Replacing
async_colocated(id, ...)
withasync(colocated(id), ...)
- PR #1462 - Consolidated task_region with N4411
- PR #1461 - Consolidate inconsistent CMake option names
- Issue #1460 - Which malloc is actually used? or at least which one is HPX built with
- Issue #1459 - Make cmake configure step fail explicitly if compiler version is not supported
- Issue #1458 - Update
parallel::task_region
with N4411 - PR #1456 - Consolidating
new_<>()
- Issue #1455 - Replace
async_colocated(id, ...)
withasync(colocated(id), ...)
- PR #1454 - Removed harmful std::moves from return statements
- PR #1453 - Use range-based for-loop instead of Boost.Foreach
- PR #1452 - C++ feature tests
- PR #1451 - When serializing, pass archive flags to traits::get_type_size
- Issue #1450 - traits:get_type_size needs archive flags to enable zero_copy optimizations
- Issue #1449 - “couldn’t create performance counter” - AGAS
- Issue #1448 - Replace distributing factories with
new_<T[]>(...)
- PR #1447 - Removing obsolete remote_object component
- PR #1446 - Hpx serialization
- PR #1445 - Replacing travis with circleci
- PR #1443 - Always stripping HPX command line arguments before executing start function
- PR #1442 - Adding –hpx:bind=none to disable thread affinities
- Issue #1439 - Libraries get linked in multiple times, RPATH is not properly set
- PR #1438 - Removed superfluous typedefs
- Issue #1437 -
hpx::init()
should strip HPX-related flags from argv - Issue #1436 - Add strong scaling option to htts
- PR #1435 - Adding
async_cb
,async_continue_cb
, andasync_colocated_cb
- PR #1434 - Added missing install rule, removed some dead CMake code
- PR #1433 - Add GitExternal and SubProject cmake scripts from eyescale/cmake repo
- Issue #1432 - Add command line flag to disable thread pinning
- PR #1431 - Fix #1423
- Issue #1430 - Inconsistent CMake option names
- Issue #1429 - Configure setting
HPX_HAVE_PARCELPORT_MPI
is ignored - PR #1428 - Fixes #1419 (closed)
- PR #1427 - Adding stencil_iterator and transform_iterator
- PR #1426 - Fixes #1419
- PR #1425 - During serialization memory allocation should honour allocator chunk size
- Issue #1424 - chunk allocation during serialization does not use memory pool/allocator chunk size
- Issue #1423 - Remove
HPX_STD_UNIQUE_PTR
- Issue #1422 - hpx:threads=all allocates too many os threads
- PR #1420 - added .travis.yml
- Issue #1419 - Unify enums:
hpx::runtime::state
andhpx::state
- PR #1416 - Adding travis builder
- Issue #1414 - Correct directory for dispatch_gcc46.hpp iteration
- Issue #1410 - Set operation algorithms
- Issue #1389 - Parallel algorithms relying on scan partitioner break for small number of elements
- Issue #1325 - Exceptions thrown during parcel handling are not handled correctly
- Issue #1315 - Errors while running performance tests
- Issue #1309 -
hpx::vector
partitions are not easily extendable by applications - PR #1300 - Added serialization/de-serialization to examples.tuplespace
- Issue #1251 - hpx::threads::get_thread_count doesn’t consider pending threads
- Issue #1008 - Decrease in application performance overtime; occasional spikes of major slowdown
- Issue #1001 - Zero copy serialization raises assert
- Issue #721 - Make HPX usable for Xeon Phi
- Issue #524 - Extend scheduler to support threads which can’t be stolen
HPX V0.9.10 (Mar 24, 2015)¶
General changes¶
This is the 12th official release of HPX. It coincides with the 7th anniversary of the first commit to our source code repository. Since then, we have seen over 12300 commits amounting to more than 220000 lines of C++ code.
The major focus of this release was to improve the reliability of large scale runs. We believe to have achieved this goal as we now can reliably run HPX applications on up to ~24k cores. We have also shown that HPX can be used with success for symmetric runs (applications using both, host cores and Intel Xeon/Phi coprocessors). This is a huge step forward in terms of the usability of HPX. The main focus of this work involved isolating the causes of the segmentation faults at start up and shut down. Many of these issues were discovered to be the result of the suspension of threads which hold locks.
A very important improvement introduced with this release is the refactoring of the code representing our parcel-port implementation. Parcel- ports can now be implemented by 3rd parties as independent plugins which are dynamically loaded at runtime (static linking of parcel-ports is also supported). This refactoring also includes a massive improvement of the performance of our existing parcel-ports. We were able to significantly reduce the networking latencies and to improve the available networking bandwidth. Please note that in this release we disabled the ibverbs and ipc parcel ports as those have not been ported to the new plugin system yet (see Issue #839).
Another corner stone of this release is our work towards a complete implementation of __cpp11_n4104__ (Working Draft, Technical Specification for C++ Extensions for Parallelism). This document defines a set of parallel algorithms to be added to the C++ standard library. We now have implemented about 75% of all specified parallel algorithms (see [link hpx.manual.parallel.parallel_algorithms Parallel Algorithms] for more details). We also implemented some extensions to __cpp11_n4104__ allowing to invoke all of the algorithms asynchronously.
This release adds a first implementation of hpx::vector
which is a
distributed data structure closely aligned to the functionality of
std::vector
. The difference is that hpx::vector
stores the data in
partitions where the partitions can be distributed over different localities. We
started to work on allowing to use the parallel algorithms with hpx::vector
.
At this point we have implemented only a few of the parallel algorithms to
support distributed data structures (like hpx::vector
) for testing purposes
(see Issue #1338 for a documentation of our progress).
Breaking changes¶
With this release we put a lot of effort into changing the code base to be more compatible to C++11. These changes have caused the following issues for backward compatibility:
- Move to Variadics- All of the API now uses variadic templates. However, this
change required to modify the argument sequence for some of the exiting API
functions (
hpx::async_continue
,hpx::apply_continue
,hpx::when_each
,hpx::wait_each
, synchronous invocation of actions). - Changes to Macros- We also removed the macros
HPX_STD_FUNCTION
andHPX_STD_TUPLE
. This shouldn’t affect any user code as we replacedHPX_STD_FUNCTION
withhpx::util::function_nonser
which was the default expansion used for this macro. All HPX API functions which expect ahpx::util::function_nonser
(or ahpx::util::unique_function_nonser
) can now be transparently called with a compatiblestd::function
instead. Similarly,HPX_STD_TUPLE
was replaced by its default expansion as well:hpx::util::tuple
. - Changes to
hpx::unique_future
-hpx::unique_future
, which was deprecated in the previous release forhpx::future
is now completely removed from HPX. This completes the transition to a completely standards conforming implementation ofhpx::future
. - Changes to Supported Compilers. Finally, in order to utilize more C++11 semantics, we have officially dropped support for GCC 4.4 and MSVC 2012. Please see our Prerequisites page for more details.
Bug fixes (closed tickets)¶
Here is a list of the important tickets we closed for this release.
- Issue #1402 - Internal shared_future serialization copies
- Issue #1399 - Build takes unusually long time…
- Issue #1398 - Tests using the scan partitioner are broken on at least gcc 4.7 and intel compiler
- Issue #1397 - Completely remove hpx::unique_future
- Issue #1396 - Parallel scan algorithms with different initial values
- Issue #1395 - Race Condition - 1d_stencil_8 - SuperMIC
- Issue #1394 - “suspending thread while at least one lock is being held” - 1d_stencil_8 - SuperMIC
- Issue #1393 - SEGFAULT in 1d_stencil_8 on SuperMIC
- Issue #1392 - Fixing #1168
- Issue #1391 - Parallel Algorithms for scan partitioner for small number of elements
- Issue #1387 - Failure with more than 4 localities
- Issue #1386 - Dispatching unhandled exceptions to outer user code
- Issue #1385 - Adding Copy algorithms, fixing
parallel::copy_if
- Issue #1384 - Fixing 1325
- Issue #1383 - Fixed #504: Refactor Dataflow LCO to work with futures, this removes the dataflow component as it is obsolete
- Issue #1382 -
is_sorted
,is_sorted_until
andis_partitioned
algorithms - Issue #1381 - fix for CMake versions prior to 3.1
- Issue #1380 - resolved warning in CMake 3.1 and newer
- Issue #1379 - Compilation error with papi
- Issue #1378 - Towards safer migration
- Issue #1377 - HPXConfig.cmake should include
TCMALLOC_LIBRARY
andTCMALLOC_INCLUDE_DIR
- Issue #1376 - Warning on uninitialized member
- Issue #1375 - Fixing 1163
- Issue #1374 - Fixing the MSVC 12 release builder
- Issue #1373 - Modifying parallel search algorithm for zero length searches
- Issue #1372 - Modifying parallel search algorithm for zero length searches
- Issue #1371 - Avoid holding a lock during agas::incref while doing a credit split
- Issue #1370 -
--hpx:bind
throws unexpected error - Issue #1369 - Getting rid of (void) in loops
- Issue #1368 - Variadic templates support for tuple
- Issue #1367 - One last batch of variadic templates support
- Issue #1366 - Fixing symbolic namespace hang
- Issue #1365 - More held locks
- Issue #1364 - Add counters 1363
- Issue #1363 - Add thread overhead counters
- Issue #1362 - Std config removal
- Issue #1361 - Parcelport plugins
- Issue #1360 - Detuplify transfer_action
- Issue #1359 - Removed obsolete checks
- Issue #1358 - Fixing 1352
- Issue #1357 - Variadic templates support for runtime_support and components
- Issue #1356 - fixed coordinate test for intel13
- Issue #1355 - fixed coordinate.hpp
- Issue #1354 - Lexicographical Compare completed
- Issue #1353 - HPX should set
Boost_ADDITIONAL_VERSIONS
flags - Issue #1352 - Error: Cannot find action ‘’ in type registry: HPX(bad_action_code)
- Issue #1351 - Variadic templates support for appliers
- Issue #1350 - Actions simplification
- Issue #1349 - Variadic when and wait functions
- Issue #1348 - Added hpx_init header to test files
- Issue #1347 - Another batch of variadic templates support
- Issue #1346 - Segmented copy
- Issue #1345 - Attempting to fix hangs during shutdown
- Issue #1344 - Std config removal
- Issue #1343 - Removing various distribution policies for hpx::vector
- Issue #1342 - Inclusive scan
- Issue #1341 - Exclusive scan
- Issue #1340 - Adding
parallel::count
for distributed data structures, adding tests - Issue #1339 - Update argument order for transform_reduce
- Issue #1337 - Fix dataflow to handle properly ranges of futures
- Issue #1336 - dataflow needs to hold onto futures passed to it
- Issue #1335 - Fails to compile with msvc14
- Issue #1334 - Examples build problem
- Issue #1333 - Distributed transform reduce
- Issue #1332 - Variadic templates support for actions
- Issue #1331 - Some ambiguous calls of map::erase have been prevented by adding additional check in locality constructor.
- Issue #1330 - Defining Plain Actions does not work as described in the documentation
- Issue #1329 - Distributed vector cleanup
- Issue #1328 - Sync docs and comments with code in hello_world example
- Issue #1327 - Typos in docs
- Issue #1326 - Documentation and code diverged in Fibonacci tutorial
- Issue #1325 - Exceptions thrown during parcel handling are not handled correctly
- Issue #1324 - fixed bandwidth calculation
- Issue #1323 - mmap() failed to allocate thread stack due to insufficient resources
- Issue #1322 - HPX fails to build aa182cf
- Issue #1321 - Limiting size of outgoing messages while coalescing parcels
- Issue #1320 - passing a future with launch::deferred in remote function call causes hang
- Issue #1319 - An exception when tries to specify number high priority threads with abp-priority
- Issue #1318 - Unable to run program with abp-priority and numa-sensitivity enabled
- Issue #1317 - N4071 Search/Search_n finished, minor changes
- Issue #1316 - Add config option to make -Ihpx.run_hpx_main!=1 the default
- Issue #1314 - Variadic support for async and apply
- Issue #1313 - Adjust when_any/some to the latest proposed interfaces
- Issue #1312 - Fixing #857: hpx::naming::locality leaks parcelport specific information into the public interface
- Issue #1311 - Distributed get’er/set’er_values for distributed vector
- Issue #1310 - Crashing in hpx::parcelset::policies::mpi::connection_handler::handle_messages() on SuperMIC
- Issue #1308 - Unable to execute an application with –hpx:threads
- Issue #1307 - merge_graph linking issue
- Issue #1306 - First batch of variadic templates support
- Issue #1305 - Create a compiler wrapper
- Issue #1304 - Provide a compiler wrapper for hpx
- Issue #1303 - Drop support for GCC44
- Issue #1302 - Fixing #1297
- Issue #1301 - Compilation error when tried to use boost range iterators with wait_all
- Issue #1298 - Distributed vector
- Issue #1297 - Unable to invoke component actions recursively
- Issue #1294 - HDF5 build error
- Issue #1275 - The parcelport implementation is non-optimal
- Issue #1267 - Added classes and unit tests for local_file, orangefs_file and pxfs_file
- Issue #1264 - Error “assertion ‘!m_fun’ failed” randomly occurs when using TCP
- Issue #1254 - thread binding seems to not work properly
- Issue #1220 - parallel::copy_if is broken
- Issue #1217 - Find a better way of fixing the issue patched by #1216
- Issue #1168 - Starting HPX on Cray machines using aprun isn’t working correctly
- Issue #1085 - Replace startup and shutdown barriers with broadcasts
- Issue #981 - With SLURM, –hpx:threads=8 should not be necessary
- Issue #857 - hpx::naming::locality leaks parcelport specific information into the public interface
- Issue #850 - “flush” not documented
- Issue #763 - Create buildbot instance that uses std::bind as HPX_STD_BIND
- Issue #680 - Convert parcel ports into a plugin system
- Issue #582 - Make exception thrown from HPX threads available from
hpx::init
- Issue #504 - Refactor Dataflow LCO to work with futures
- Issue #196 - Don’t store copies of the locality network metadata in the gva table
HPX V0.9.9 (Oct 31, 2014, codename Spooky)¶
General changes¶
We have had over 1500 commits since the last release and we have closed over 200 tickets (bugs, feature requests, pull requests, etc.). These are by far the largest numbers of commits and resolved issues for any of the HPX releases so far. We are especially happy about the large number of people who contributed for the first time to HPX.
We completed the transition from the older (non-conforming) implementation of
hpx::future
to the new and fully conforming version by removing the old code and by renaming the typehpx::unique_future
tohpx::future
. In order to maintain backwards compatibility with existing code which uses the typehpx::unique_future
we support the configuration variableHPX_UNIQUE_FUTURE_ALIAS
. If this variable is set toON
while running cmake it will additionally define a template alias for this type.We rewrote and significantly changed our build system. Please have a look at the new (now generated) documentation here: HPX build system. Please revisit your build scripts to adapt to the changes. The most notable changes are:
HPX_NO_INSTALL
is no longer necessary.- For external builds, you need to set
HPX_DIR
instead ofHPX_ROOT
as described here: Using HPX with CMake-based projects. - IDEs that support multiple configurations (Visual Studio and XCode) can now be used as intended. that means no build dir.
- Building HPX statically (without dynamic libraries) is now supported
(
-DHPX_STATIC_LINKING=On
). - Please note that many variables used to configure the build process have been renamed to unify the naming conventions (see the section CMake variables used to configure HPX for more information).
- This also fixes a long list of issues, for more information see Issue #1204.
We started to implement various proposals to the C++ Standardization committee related to parallelism and concurrency, most notably N4409 (Working Draft, Technical Specification for C++ Extensions for Parallelism), N4411 (Task Region Rev. 3), and N4313 (Working Draft, Technical Specification for C++ Extensions for Concurrency).
We completely remodeled our automatic build system to run builds and unit tests on various systems and compilers. This allows us to find most bugs right as they were introduced and helps to maintain a high level of quality and compatibility. The newest build logs can be found at HPX Buildbot Website.
Bug fixes (closed tickets)¶
Here is a list of the important tickets we closed for this release.
- Issue #1296 - Rename make_error_future to make_exceptional_future, adjust to N4123
- Issue #1295 - building issue
- Issue #1293 - Transpose example
- Issue #1292 - Wrong abs() function used in example
- Issue #1291 - non-synchronized shift operators have been removed
- Issue #1290 - RDTSCP is defined as true for Xeon Phi build
- Issue #1289 - Fixing 1288
- Issue #1288 - Add new performance counters
- Issue #1287 - Hierarchy scheduler broken performance counters
- Issue #1286 - Algorithm cleanup
- Issue #1285 - Broken Links in Documentation
- Issue #1284 - Uninitialized copy
- Issue #1283 - missing boost::scoped_ptr includes
- Issue #1282 - Update documentation of build options for schedulers
- Issue #1281 - reset idle rate counter
- Issue #1280 - Bug when executing on Intel MIC
- Issue #1279 - Add improved when_all/wait_all
- Issue #1278 - Implement improved when_all/wait_all
- Issue #1277 - feature request: get access to argc argv and variables_map
- Issue #1276 - Remove merging map
- Issue #1274 - Weird (wrong) string code in papi.cpp
- Issue #1273 - Sequential task execution policy
- Issue #1272 - Avoid CMake name clash for Boost.Thread library
- Issue #1271 - Updates on HPX Test Units
- Issue #1270 - hpx/util/safe_lexical_cast.hpp is added
- Issue #1269 - Added default value for “LIB” cmake variable
- Issue #1268 - Memory Counters not working
- Issue #1266 - FindHPX.cmake is not installed
- Issue #1263 - apply_remote test takes too long
- Issue #1262 - Chrono cleanup
- Issue #1261 - Need make install for papi counters and this builds all the examples
- Issue #1260 - Documentation of Stencil example claims
- Issue #1259 - Avoid double-linking Boost on Windows
- Issue #1257 - Adding additional parameter to create_thread
- Issue #1256 - added buildbot changes to release notes
- Issue #1255 - Cannot build MiniGhost
- Issue #1253 - hpx::thread defects
- Issue #1252 - HPX_PREFIX is too fragile
- Issue #1250 - switch_to_fiber_emulation does not work properly
- Issue #1249 - Documentation is generated under Release folder
- Issue #1248 - Fix usage of hpx_generic_coroutine_context and get tests passing on powerpc
- Issue #1247 - Dynamic linking error
- Issue #1246 - Make cpuid.cpp C++11 compliant
- Issue #1245 - HPX fails on startup (setting thread affinity mask)
- Issue #1244 - HPX_WITH_RDTSC configure test fails, but should succeed
- Issue #1243 - CTest dashboard info for CSCS CDash drop location
- Issue #1242 - Mac fixes
- Issue #1241 - Failure in Distributed with Boost 1.56
- Issue #1240 - fix a race condition in examples.diskperf
- Issue #1239 - fix wait_each in examples.diskperf
- Issue #1238 - Fixed #1237: hpx::util::portable_binary_iarchive failed
- Issue #1237 - hpx::util::portable_binary_iarchive faileds
- Issue #1235 - Fixing clang warnings and errors
- Issue #1234 - TCP runs fail: Transport endpoint is not connected
- Issue #1233 - Making sure the correct number of threads is registered with AGAS
- Issue #1232 - Fixing race in wait_xxx
- Issue #1231 - Parallel minmax
- Issue #1230 - Distributed run of 1d_stencil_8 uses less threads than spec. & sometimes gives errors
- Issue #1229 - Unstable number of threads
- Issue #1228 - HPX link error (cmake / MPI)
- Issue #1226 - Warning about struct/class thread_counters
- Issue #1225 - Adding parallel::replace etc
- Issue #1224 - Extending dataflow to pass through non-future arguments
- Issue #1223 - Remaining find algorithms implemented, N4071
- Issue #1222 - Merging all the changes
- Issue #1221 - No error output when using mpirun with hpx
- Issue #1219 - Adding new AGAS cache performance counters
- Issue #1216 - Fixing using futures (clients) as arguments to actions
- Issue #1215 - Error compiling simple component
- Issue #1214 - Stencil docs
- Issue #1213 - Using more than a few dozen MPI processes on SuperMike results in a seg fault before getting to hpx_main
- Issue #1212 - Parallel rotate
- Issue #1211 - Direct actions cause the future’s shared_state to be leaked
- Issue #1210 - Refactored local::promise to be standard conformant
- Issue #1209 - Improve command line handling
- Issue #1208 - Adding parallel::reverse and parallel::reverse_copy
- Issue #1207 - Add copy_backward and move_backward
- Issue #1206 - N4071 additional algorithms implemented
- Issue #1204 - Cmake simplification and various other minor changes
- Issue #1203 - Implementing new launch policy for (local) async:
hpx::launch::fork
. - Issue #1202 - Failed assertion in connection_cache.hpp
- Issue #1201 - pkg-config doesn’t add mpi link directories
- Issue #1200 - Error when querying time performance counters
- Issue #1199 - library path is now configurable (again)
- Issue #1198 - Error when querying performance counters
- Issue #1197 - tests fail with intel compiler
- Issue #1196 - Silence several warnings
- Issue #1195 - Rephrase initializers to work with VC++ 2012
- Issue #1194 - Simplify parallel algorithms
- Issue #1193 - Adding
parallel::equal
- Issue #1192 - HPX(out_of_memory) on including <hpx/hpx.hpp>
- Issue #1191 - Fixing #1189
- Issue #1190 - Chrono cleanup
- Issue #1189 - Deadlock .. somewhere? (probably serialization)
- Issue #1188 - Removed
future::get_status()
- Issue #1186 - Fixed FindOpenCL to find current AMD APP SDK
- Issue #1184 - Tweaking future unwrapping
- Issue #1183 - Extended
parallel::reduce
- Issue #1182 -
future::unwrap
hangs forlaunch::deferred
- Issue #1181 - Adding
all_of
,any_of
, andnone_of
and corresponding documentation - Issue #1180 -
hpx::cout
defect - Issue #1179 -
hpx::async
does not work for member function pointers when called on types with self-defined unaryoperator*
- Issue #1178 - Implemented variadic
hpx::util::zip_iterator
- Issue #1177 - MPI parcelport defect
- Issue #1176 -
HPX_DEFINE_COMPONENT_CONST_ACTION_TPL
does not have a 2-argument version - Issue #1175 - Create util::zip_iterator working with util::tuple<>
- Issue #1174 - Error Building HPX on linux, root_certificate_authority.cpp
- Issue #1173 - hpx::cout output lost
- Issue #1172 - HPX build error with Clang 3.4.2
- Issue #1171 -
CMAKE_INSTALL_PREFIX
ignored - Issue #1170 - Close hpx_benchmarks repository on Github
- Issue #1169 - Buildbot emails have syntax error in url
- Issue #1167 - Merge partial implementation of standards proposal N3960
- Issue #1166 - Fixed several compiler warnings
- Issue #1165 - cmake warns: “tests.regressions.actions” does not exist
- Issue #1164 - Want my own serialization of hpx::future
- Issue #1162 - Segfault in hello_world example
- Issue #1161 - Use
HPX_ASSERT
to aid the compiler - Issue #1160 - Do not put -DNDEBUG into hpx_application.pc
- Issue #1159 - Support Clang 3.4.2
- Issue #1158 - Fixed #1157: Rename when_n/wait_n, add when_xxx_n/wait_xxx_n
- Issue #1157 - Rename when_n/wait_n, add when_xxx_n/wait_xxx_n
- Issue #1156 - Force inlining fails
- Issue #1155 - changed header of printout to be compatible with python csv module
- Issue #1154 - Fixing iostreams
- Issue #1153 - Standard manipulators (like std::endl) do not work with hpx::ostream
- Issue #1152 - Functions revamp
- Issue #1151 - Supressing cmake 3.0 policy warning for CMP0026
- Issue #1150 - Client Serialization error
- Issue #1149 - Segfault on Stampede
- Issue #1148 - Refactoring mini-ghost
- Issue #1147 - N3960 copy_if and copy_n implemented and tested
- Issue #1146 - Stencil print
- Issue #1145 - N3960 hpx::parallel::copy implemented and tested
- Issue #1144 - OpenMP examples 1d_stencil do not build
- Issue #1143 - 1d_stencil OpenMP examples do not build
- Issue #1142 - Cannot build HPX with gcc 4.6 on OS X
- Issue #1140 - Fix OpenMP lookup, enable usage of config tests in external CMake projects.
- Issue #1139 - hpx/hpx/config/compiler_specific.hpp
- Issue #1138 - clean up pkg-config files
- Issue #1137 - Improvements to create binary packages
- Issue #1136 - HPX_GCC_VERSION not defined on all compilers
- Issue #1135 - Avoiding collision between winsock2.h and windows.h
- Issue #1134 - Making sure, that hpx::finalize can be called from any locality
- Issue #1133 - 1d stencil examples
- Issue #1131 - Refactor unique_function implementation
- Issue #1130 - Unique function
- Issue #1129 - Some fixes to the Build system on OS X
- Issue #1128 - Action future args
- Issue #1127 - Executor causes segmentation fault
- Issue #1124 - Adding new API functions:
register_id_with_basename
,unregister_id_with_basename
,find_ids_from_basename
; adding test - Issue #1123 - Reduce nesting of try-catch construct in
encode_parcels
? - Issue #1122 - Client base fixes
- Issue #1121 - Update
hpxrun.py.in
- Issue #1120 - HTTS2 tests compile errors on v110 (VS2012)
- Issue #1119 - Remove references to boost::atomic in accumulator example
- Issue #1118 - Only build test thread_pool_executor_1114_test if
HPX_LOCAL_SCHEDULER
is set - Issue #1117 - local_queue_executor linker error on vc110
- Issue #1116 - Disabled performance counter should give runtime errors, not invalid data
- Issue #1115 - Compile error with Intel C++ 13.1
- Issue #1114 - Default constructed executor is not usable
- Issue #1113 - Fast compilation of logging causes ABI incompatibilities
between different
NDEBUG
values - Issue #1112 - Using thread_pool_executors causes segfault
- Issue #1111 -
hpx::threads::get_thread_data
always returns zero - Issue #1110 - Remove unnecessary null pointer checks
- Issue #1109 - More tests adjustments
- Issue #1108 - Clarify build rules for “libboost_atomic-mt.so”?
- Issue #1107 - Remove unnecessary null pointer checks
- Issue #1106 - network_storage benchmark imporvements, adding legends to plots and tidying layout
- Issue #1105 - Add more plot outputs and improve instructions doc
- Issue #1104 - Complete quoting for parameters of some CMake commands
- Issue #1103 - Work on test/scripts
- Issue #1102 - Changed minimum requirement of window install to 2012
- Issue #1101 - Changed minimum requirement of window install to 2012
- Issue #1100 - Changed readme to no longer specify using MSVC 2010 compiler
- Issue #1099 - Error returning futures from component actions
- Issue #1098 - Improve storage test
- Issue #1097 - data_actions quickstart example calls missing function decorate_action of data_get_action
- Issue #1096 - MPI parcelport broken with new zero copy optimization
- Issue #1095 - Warning C4005: _WIN32_WINNT: Macro redefinition
- Issue #1094 - Syntax error for -DHPX_UNIQUE_FUTURE_ALIAS in master
- Issue #1093 - Syntax error for -DHPX_UNIQUE_FUTURE_ALIAS
- Issue #1092 - Rename unique_future<> back to future<>
- Issue #1091 - Inconsistent error message
- Issue #1090 - On windows 8.1 the examples crashed if using more than one os thread
- Issue #1089 - Components should be allowed to have their own executor
- Issue #1088 - Add possibility to select a network interface for the ibverbs parcelport
- Issue #1087 - ibverbs and ipc parcelport uses zero copy optimization
- Issue #1083 - Make shell examples copyable in docs
- Issue #1082 - Implement proper termination detection during shutdown
- Issue #1081 - Implement thread_specific_ptr for hpx::threads
- Issue #1072 - make install not working properly
- Issue #1070 - Complete quoting for parameters of some CMake commands
- Issue #1059 - Fix more unused variable warnings
- Issue #1051 - Implement when_each
- Issue #973 - Would like option to report hwloc bindings
- Issue #970 - Bad flags for Fortran compiler
- Issue #941 - Create a proper user level context switching class for BG/Q
- Issue #935 - Build error with gcc 4.6 and Boost 1.54.0 on hpx trunk and 0.9.6
- Issue #934 - Want to build HPX without dynamic libraries
- Issue #927 - Make hpx/lcos/reduce.hpp accept futures of id_type
- Issue #926 - All unit tests that are run with more than one thread with CTest/hpx_run_test should configure hpx.os_threads
- Issue #925 - regression_dataflow_791 needs to be brought in line with HPX standards
- Issue #899 - Fix race conditions in regression tests
- Issue #879 - Hung test leads to cascading test failure; make tests should support the MPI parcelport
- Issue #865 - future<T> and friends shall work for movable only Ts
- Issue #847 - Dynamic libraries are not installed on OS X
- Issue #816 - First Program tutorial pull request
- Issue #799 - Wrap lexical_cast to avoid exceptions
- Issue #720 - broken configuration when using ccmake on Ubuntu
- Issue #622 -
--hpx:hpx
and--hpx:debug-hpx-log
is nonsensical - Issue #525 - Extend barrier LCO test to run in distributed
- Issue #515 - Multi-destination version of hpx::apply is broken
- Issue #509 - Push Boost.Atomic changes upstream
- Issue #503 - Running HPX applications on Windows should not require setting %PATH%
- Issue #461 - Add a compilation sanity test
- Issue #456 - hpx_run_tests.py should log output from tests that timeout
- Issue #454 - Investigate threadmanager performance
- Issue #345 - Add more versatile environmental/cmake variable support to hpx_find_* CMake macros
- Issue #209 - Support multiple configurations in generated build files
- Issue #190 - hpx::cout should be a std::ostream
- Issue #189 - iostreams component should use startup/shutdown functions
- Issue #183 - Use Boost.ICL for correctness in AGAS
- Issue #44 - Implement real futures
HPX V0.9.8 (Mar 24, 2014)¶
We have had over 800 commits since the last release and we have closed over 65 tickets (bugs, feature requests, etc.).
With the changes below, HPX is once again leading the charge of a whole new era of computation. By intrinsically breaking down and synchronizing the work to be done, HPX insures that application developers will no longer have to fret about where a segment of code executes. That allows coders to focus their time and energy to understanding the data dependencies of their algorithms and thereby the core obstacles to an efficient code. Here are some of the advantages of using HPX:
- HPX is solidly rooted in a sophisticated theoretical execution model – ParalleX
- HPX exposes an API fully conforming to the C++11 and the draft C++14 standards, extended and applied to distributed computing. Everything programmers know about the concurrency primitives of the standard C++ library is still valid in the context of HPX.
- It provides a competitive, high performance implementation of modern, future-proof ideas which gives an smooth migration path from todays mainstream techniques
- There is no need for the programmer to worry about lower level parallelization paradigms like threads or message passing; no need to understand pthreads, MPI, OpenMP, or Windows threads, etc.
- There is no need to think about different types of parallelism such as tasks, pipelines, or fork-join, task or data parallelism.
- The same source of your program compiles and runs on Linux, BlueGene/Q, Mac OS X, Windows, and Android.
- The same code runs on shared memory multi-core systems and supercomputers, on handheld devices and Intel® Xeon Phi™ accelerators, or a heterogeneous mix of those.
General changes¶
- A major API breaking change for this release was introduced by implementing
hpx::future
andhpx::shared_future
fully in conformance with the C++11 Standard. Whilehpx::shared_future
is new and will not create any compatibility problems, we revised the interface and implementation of the existinghpx::future
. For more details please see the mailing list archive. To avoid any incompatibilities for existing code we named the type which implements thestd::future
interface ashpx::unique_future
. For the next release this will be renamed tohpx::future
, making it full conforming to C++11 Standard. - A large part of the code base of HPX has been refactored and partially
re-implemented. The main changes were related to
- The threading subsystem: these changes significantly reduce the amount of overheads caused by the schedulers, improve the modularity of the code base, and extend the variety of available scheduling algorithms.
- The parcel subsystem: these changes improve the performance of the HPX networking layer, modularize the structure of the parcelports, and simplify the creation of new parcelports for other underlying networking libraries.
- The API subsystem: these changes improved the conformance of the API to C++11 Standard, extend and unify the available API functionality, and decrease the overheads created by various elements of the API.
- The robustness of the component loading subsystem has been improved significantly, allowing to more portably and more reliably register the components needed by an application as startup. This additionally speeds up general application initialization.
- We added new API functionality like
hpx::migrate
andhpx::copy_component
which are the basic building blocks necessary for implementing higher level abstractions for system-wide load balancing, runtime-adaptive resource management, and object-oriented checkpointing and state-management. - We removed the use of C++11 move emulation (using Boost.Move), replacing it with C++11 rvalue references. This is the first step towards using more and more native C++11 facilities which we plan to introduce in the future.
- We improved the reference counting scheme used by HPX which helps managing distributed objects and memory. This improves the overall stability of HPX and further simplifies writing real world applications.
- The minimal Boost version required to use HPX is now V1.49.0.
- This release coincides with the first release of HPXPI (V0.1.0), the first implementation of the XPI specification.
Bug fixes (closed tickets)¶
Here is a list of the important tickets we closed for this release.
- Issue #1086 - Expose internal boost::shared_array to allow user management of array lifetime
- Issue #1083 - Make shell examples copyable in docs
- Issue #1080 - /threads{locality#*/total}/count/cumulative broken
- Issue #1079 - Build problems on OS X
- Issue #1078 - Improve robustness of component loading
- Issue #1077 - Fix a missing enum definition for ‘take’ mode
- Issue #1076 - Merge Jb master
- Issue #1075 - Unknown CMake command “add_hpx_pseudo_target”
- Issue #1074 - Implement
apply_continue_callback
andapply_colocated_callback
- Issue #1073 - The new
apply_colocated
andasync_colocated
functions lead to automatic registered functions - Issue #1071 - Remove deferred_packaged_task
- Issue #1069 - serialize_buffer with allocator fails at destruction
- Issue #1068 - Coroutine include and forward declarations missing
- Issue #1067 - Add allocator support to util::serialize_buffer
- Issue #1066 - Allow for MPI_Init being called before HPX launches
- Issue #1065 - AGAS cache isn’t used/populated on worker localities
- Issue #1064 - Reorder includes to ensure ws2 includes early
- Issue #1063 - Add
hpx::runtime::suspend
andhpx::runtime::resume
- Issue #1062 - Fix
async_continue
to propery handle return types - Issue #1061 - Implement
async_colocated
andapply_colocated
- Issue #1060 - Implement minimal component migration
- Issue #1058 - Remove
HPX_UTIL_TUPLE
from code base - Issue #1057 - Add performance counters for threading subsystem
- Issue #1055 - Thread allocation uses two memory pools
- Issue #1053 - Work stealing flawed
- Issue #1052 - Fix a number of warnings
- Issue #1049 - Fixes for TLS on OSX and more reliable test running
- Issue #1048 - Fixing after 588 hang
- Issue #1047 - Use port ‘0’ for networking when using one locality
- Issue #1046 -
composable_guard
test is broken when having more than one thread - Issue #1045 - Security missing headers
- Issue #1044 - Native TLS on FreeBSD via __thread
- Issue #1043 -
async
et.al. compute the wrong result type - Issue #1042 -
async
et.al. implicitly unwrap reference_wrappers - Issue #1041 - Remove redundant costly Kleene stars from regex searches
- Issue #1040 - CMake script regex match patterns has unnecessary kleenes
- Issue #1039 - Remove use of Boost.Move and replace with std::move and real rvalue refs
- Issue #1038 - Bump minimal required Boost to 1.49.0
- Issue #1037 - Implicit unwrapping of futures in async broken
- Issue #1036 - Scheduler hangs when user code attempts to “block” OS-threads
- Issue #1035 - Idle-rate counter always reports 100% idle rate
- Issue #1034 - Symbolic name registration causes application hangs
- Issue #1033 - Application options read in from an options file generate an error message
- Issue #1032 -
hpx::id_type
local reference counting is wrong - Issue #1031 - Negative entry in reference count table
- Issue #1030 - Implement condition_variable
- Issue #1029 - Deadlock in thread scheduling subsystem
- Issue #1028 - HPX-thread cumulative count performance counters report incorrect value
- Issue #1027 - Expose
hpx::thread_interrupted
error code as a separate exception type - Issue #1026 - Exceptions thrown in asynchronous calls can be lost if the value of the future is never queried
- Issue #1025 -
future::wait_for
/wait_until
do not remove callback - Issue #1024 - Remove dependence to boost assert and create hpx assert
- Issue #1023 - Segfaults with tcmalloc
- Issue #1022 - prerequisites link in readme is broken
- Issue #1020 - HPX Deadlock on external synchronization
- Issue #1019 - Convert using
BOOST_ASSERT
toHPX_ASSERT
- Issue #1018 - compiling bug with gcc 4.8.1
- Issue #1017 - Possible crash in io_pool executor
- Issue #1016 - Crash at startup
- Issue #1014 - Implement Increment/Decrement Merging
- Issue #1013 - Add more logging channels to enable greater control over logging granularity
- Issue #1012 -
--hpx:debug-hpx-log
and--hpx:debug-agas-log
lead to non-thread safe writes - Issue #1011 - After installation, running applications from the build/staging directory no longer works
- Issue #1010 - Mergable decrement requests are not being merged
- Issue #1009 -
--hpx:list-symbolic-names
crashes - Issue #1007 - Components are not properly destroyed
- Issue #1006 - Segfault/hang in set_data
- Issue #1003 - Performance counter naming issue
- Issue #982 - Race condition during startup
- Issue #912 - OS X: component type not found in map
- Issue #663 - Create a buildbot slave based on Clang 3.2/OSX
- Issue #636 - Expose
this_locality::apply<act>(p1, p2);
for local execution - Issue #197 - Add
--console=address
option for PBS runs - Issue #175 - Asynchronous AGAS API
HPX V0.9.7 (Nov 13, 2013)¶
We have had over 1000 commits since the last release and we have closed over 180 tickets (bugs, feature requests, etc.).
General changes¶
- Ported HPX to BlueGene/Q
- Improved HPX support for Xeon/Phi accelerators
- Reimplemented
hpx::bind
,hpx::tuple
, andhpx::function
for better performance and better compliance with the C++11 Standard. Addedhpx::mem_fn
. - Reworked
hpx::when_all
andhpx::when_any
for better compliance with the ongoing C++ standardization effort, added heterogeneous version for those functions. Addedhpx::when_any_swapped
. - Added
hpx::copy
as a precursor for a migrate functionality - Added
hpx::get_ptr
allowing to directly access the memory underlying a given component - Added the
hpx::lcos::broadcast
,hpx::lcos::reduce
, andhpx::lcos::fold
collective operations - Added
hpx::get_locality_name
allowing to retrieve the name of any of the localities for the application. - Added support for more flexible thread affinity control from the HPX command
line, such as new modes for
--hpx:bind
(balanced
,scattered
,compact
), improved default settings when running multiple localities on the same node. - Added experimental executors for simpler thread pooling and scheduling. This API may change in the future as it will stay aligned with the ongoing C++ standardization efforts.
- Massively improved the performance of the HPX serialization code. Added partial support for zero copy serialization of array and bitwise-copyable types.
- General performance improvements of the code related to threads and futures.
Bug fixes (closed tickets)¶
Here is a list of the important tickets we closed for this release.
- Issue #1005 - Allow to disable array optimizations and zero copy optimizations for each parcelport
- Issue #1004 - Generate new HPX logo image for the docs
- Issue #1002 - If MPI parcelport is not available, running HPX under mpirun should fail
- Issue #1001 - Zero copy serialization raises assert
- Issue #1000 - Can’t connect to a HPX application running with the MPI parcelport from a non MPI parcelport locality
- Issue #999 - Optimize
hpx::when_n
- Issue #998 - Fixed const-correctness
- Issue #997 - Making serialize_buffer::data() type save
- Issue #996 - Memory leak in hpx::lcos::promise
- Issue #995 - Race while registering pre-shutdown functions
- Issue #994 - thread_rescheduling regression test does not compile
- Issue #992 - Correct comments and messages
- Issue #991 - setcap cap_sys_rawio=ep for power profiling causes an HPX application to abort
- Issue #989 - Jacobi hangs during execution
- Issue #988 - multiple_init test is failing
- Issue #986 - Can’t call a function called “init” from “main” when using
<hpx/hpx_main.hpp>
- Issue #984 - Reference counting tests are failing
- Issue #983 - thread_suspension_executor test fails
- Issue #980 - Terminating HPX threads don’t leave stack in virgin state
- Issue #979 - Static scheduler not in documents
- Issue #978 - Preprocessing limits are broken
- Issue #977 - Make tests.regressions.lcos.future_hang_on_get shorter
- Issue #976 - Wrong library order in pkgconfig
- Issue #975 - Please reopen #963
- Issue #974 - Option pu-offset ignored in fixing_588 branch
- Issue #972 - Cannot use MKL with HPX
- Issue #969 - Non-existent INI files requested on the command line via
--hpx:config
do not cause warnings or errors. - Issue #968 - Cannot build examples in fixing_588 branch
- Issue #967 - Command line description of
--hpx:queuing
seems wrong - Issue #966 -
--hpx:print-bind
physical core numbers are wrong - Issue #965 - Deadlock when building in Release mode
- Issue #963 - Not all worker threads are working
- Issue #962 - Problem with SLURM integration
- Issue #961 -
--hpx:print-bind
outputs incorrect information - Issue #960 - Fix cut and paste error in documentation of get_thread_priority
- Issue #959 - Change link to boost.atomic in documentation to point to boost.org
- Issue #958 - Undefined reference to intrusive_ptr_release
- Issue #957 - Make tuple standard compliant
- Issue #956 - Segfault with a3382fb
- Issue #955 -
--hpx:nodes
and--hpx:nodefiles
do not work with foreign nodes - Issue #954 - Make order of arguments for hpx::async and hpx::broadcast consistent
- Issue #953 - Cannot use MKL with HPX
- Issue #952 -
register_[pre_]shutdown_function
never throw - Issue #951 - Assert when number of threads is greater than hardware concurrency
- Issue #948 -
HPX_HAVE_GENERIC_CONTEXT_COROUTINES
conflicts withHPX_HAVE_FIBER_BASED_COROUTINES
- Issue #947 - Need MPI_THREAD_MULTIPLE for backward compatibility
- Issue #946 - HPX does not call
MPI_Finalize
- Issue #945 - Segfault with
hpx::lcos::broadcast
- Issue #944 - OS X: assertion
pu_offset_ < hardware_concurrency
failed - Issue #943 - #include <hpx/hpx_main.hpp> does not work
- Issue #942 - Make the BG/Q work with -O3
- Issue #940 - Use separator when concatenating locality name
- Issue #939 - Refactor MPI parcelport to use
MPI_Wait
instead of multipleMPI_Test
calls - Issue #938 - Want to officially access
client_base::gid_
- Issue #937 -
client_base::gid_
should be private`` - Issue #936 - Want doxygen-like source code index
- Issue #935 - Build error with gcc 4.6 and Boost 1.54.0 on hpx trunk and 0.9.6
- Issue #933 - Cannot build HPX with Boost 1.54.0
- Issue #932 - Components are destructed too early
- Issue #931 - Make HPX work on BG/Q
- Issue #930 - make git-docs is broken
- Issue #929 - Generating index in docs broken
- Issue #928 - Optimize
hpx::util::static_
for C++11 compilers supporting magic statics - Issue #924 - Make kill_process_tree (in process.py) more robust on Mac OSX
- Issue #923 - Correct BLAS and RNPL cmake tests
- Issue #922 - Cannot link against BLAS
- Issue #921 - Implement
hpx::mem_fn
- Issue #920 - Output locality with
--hpx:print-bind
- Issue #919 - Correct grammar; simplify boolean expressions
- Issue #918 - Link to hello_world.cpp is broken
- Issue #917 - adapt cmake file to new boostbook version
- Issue #916 - fix problem building documentation with xsltproc >= 1.1.27
- Issue #915 - Add another TBBMalloc library search path
- Issue #914 - Build problem with Intel compiler on Stampede (TACC)
- Issue #913 - fix error messages in fibonacci examples
- Issue #911 - Update OS X build instructions
- Issue #910 - Want like to specify MPI_ROOT instead of compiler wrapper script
- Issue #909 - Warning about void* arithmetic
- Issue #908 - Buildbot for MIC is broken
- Issue #906 - Can’t use
--hpx:bind=balanced
with multiple MPI processes - Issue #905 -
--hpx:bind
documentation should describe full grammar - Issue #904 - Add hpx::lcos::fold and hpx::lcos::inverse_fold collective operation
- Issue #903 - Add
hpx::when_any_swapped()
- Issue #902 - Add
hpx::lcos::reduce
collective operation - Issue #901 - Web documentation is not searchable
- Issue #900 - Web documentation for trunk has no index
- Issue #898 - Some tests fail with GCC 4.8.1 and MPI parcel port
- Issue #897 - HWLOC causes failures on Mac
- Issue #896 - pu-offset leads to startup error
- Issue #895 -
hpx::get_locality_name
not defined - Issue #894 - Race condition at shutdown
- Issue #893 -
--hpx:print-bind
switches std::cout to hexadecimal mode - Issue #892 -
hwloc_topology_load
can be expensive – don’t call multiple times - Issue #891 - The documentation for
get_locality_name
is wrong - Issue #890 -
--hpx:print-bind
should not exit - Issue #889 -
--hpx:debug-hpx-log=FILE
does not work - Issue #888 - MPI parcelport does not exit cleanly for –hpx:print-bind
- Issue #887 - Choose thread affinities more cleverly
- Issue #886 - Logging documentation is confusing
- Issue #885 - Two threads are slower than one
- Issue #884 - is_callable failing with member pointers in C++11
- Issue #883 - Need help with is_callable_test
- Issue #882 - tests.regressions.lcos.future_hang_on_get does not terminate
- Issue #881 - tests/regressions/block_matrix/matrix.hh won’t compile with GCC 4.8.1
- Issue #880 - HPX does not work on OS X
- Issue #878 -
future::unwrap
triggers assertion - Issue #877 - “make tests” has build errors on Ubuntu 12.10
- Issue #876 - tcmalloc is used by default, even if it is not present
- Issue #875 - global_fixture is defined in a header file
- Issue #874 - Some tests take very long
- Issue #873 - Add block-matrix code as regression test
- Issue #872 - HPX documentation does not say how to run tests with detailed output
- Issue #871 - All tests fail with “make test”
- Issue #870 - Please explicitly disable serialization in classes that don’t support it
- Issue #868 - boost_any test failing
- Issue #867 - Reduce the number of copies of
hpx::function
arguments - Issue #863 - Futures should not require a default constructor
- Issue #862 - value_or_error shall not default construct its result
- Issue #861 -
HPX_UNUSED
macro - Issue #860 - Add functionality to copy construct a component
- Issue #859 -
hpx::endl
should flush - Issue #858 - Create
hpx::get_ptr<>
allowing to access component implementation - Issue #855 - Implement
hpx::INVOKE
- Issue #854 -
hpx/hpx.hpp
does not includehpx/include/iostreams.hpp
- Issue #853 - Feature request: null future
- Issue #852 - Feature request: Locality names
- Issue #851 -
hpx::cout
output does not appear on screen - Issue #849 - All tests fail on OS X after installing
- Issue #848 - Update OS X build instructions
- Issue #846 - Update hpx_external_example
- Issue #845 - Issues with having both debug and release modules in the same directory
- Issue #844 - Create configuration header
- Issue #843 - Tests should use CTest
- Issue #842 - Remove buffer_pool from MPI parcelport
- Issue #841 - Add possibility to broadcast an index with hpx::lcos::broadcast
- Issue #838 - Simplify
util::tuple
- Issue #837 - Adopt boost::tuple tests for
util::tuple
- Issue #836 - Adopt boost::function tests for
util::function
- Issue #835 - Tuple interface missing pieces
- Issue #833 - Partially preprocessing files not working
- Issue #832 - Native papi counters do not work with wild cards
- Issue #831 - Arithmetics counter fails if only one parameter is given
- Issue #830 - Convert hpx::util::function to use new scheme for serializing its base pointer
- Issue #829 - Consistently use
decay<T>
instead ofremove_const< remove_reference<T>>
- Issue #828 - Update future implementation to N3721 and N3722
- Issue #827 - Enable MPI parcelport for bootstrapping whenever application was started using mpirun
- Issue #826 - Support command line option
--hpx:print-bind
even if--hpx::bind
was not used - Issue #825 - Memory counters give segfault when attempting to use thread wild cards or numbers only total works
- Issue #824 - Enable lambda functions to be used with hpx::async/hpx::apply
- Issue #823 - Using a hashing filter
- Issue #822 - Silence unused variable warning
- Issue #821 - Detect if a function object is callable with given arguments
- Issue #820 - Allow wildcards to be used for performance counter names
- Issue #819 - Make the AGAS symbolic name registry distributed
- Issue #818 - Add future::then() overload taking an executor
- Issue #817 - Fixed typo
- Issue #815 - Create an lco that is performing an efficient broadcast of actions
- Issue #814 - Papi counters cannot specify thread#* to get the counts for all threads
- Issue #813 - Scoped unlock
- Issue #811 - simple_central_tuplespace_client run error
- Issue #810 - ostream error when << any objects
- Issue #809 - Optimize parcel serialization
- Issue #808 - HPX applications throw exception when executed from the build directory
- Issue #807 - Create performance counters exposing overall AGAS statistics
- Issue #795 - Create timed make_ready_future
- Issue #794 - Create heterogeneous
when_all
/when_any
/etc. - Issue #721 - Make HPX usable for Xeon Phi
- Issue #694 - CMake should complain if you attempt to build an example without its dependencies
- Issue #692 - SLURM support broken
- Issue #683 - python/hpx/process.py imports epoll on all platforms
- Issue #619 - Automate the doc building process
- Issue #600 - GTC performance broken
- Issue #577 - Allow for zero copy serialization/networking
- Issue #551 - Change executable names to have debug postfix in Debug builds
- Issue #544 - Write a custom .lib file on Windows pulling in hpx_init and hpx.dll, phase out hpx_init
- Issue #534 -
hpx::init
should take functions bystd::function
and should accept all forms of hpx_main - Issue #508 - FindPackage fails to set FOO_LIBRARY_DIR
- Issue #506 - Add cmake support to generate ini files for external applications
- Issue #470 - Changing build-type after configure does not update boost library names
- Issue #453 - Document
hpx_run_tests.py
- Issue #445 - Significant performance mismatch between MPI and HPX in SMP for allgather example
- Issue #443 - Make docs viewable from build directory
- Issue #421 - Support multiple HPX instances per node in a batch environment like PBS or SLURM
- Issue #316 - Add message size limitation
- Issue #249 - Clean up locking code in big boot barrier
- Issue #136 - Persistent CMake variables need to be marked as cache variables
HPX V0.9.6 (Jul 30, 2013)¶
We have had over 1200 commits since the last release and we have closed roughly 140 tickets (bugs, feature requests, etc.).
General changes¶
The major new fetures in this release are:
- We further consolidated the API exposed by HPX. We aligned our APIs as much as possible with the existing C++11 Standard and related proposals to the C++ standardization committee (such as N3632 and N3857).
- We implemented a first version of a distributed AGAS service which essentially eliminates all explicit AGAS network traffic.
- We created a native ibverbs parcelport allowing to take advantage of the superior latency and bandwidth characteristics of Infiniband networks.
- We successfully ported HPX to the Xeon Phi platform.
- Support for the SLURM scheduling system was implemented.
- Major efforts have been dedicated to improving the performance counter framework, numerous new counters were implemented and new APIs were added.
- We added a modular parcel compression system allowing to improve bandwidth utilization (by reducing the overall size of the tranferred data).
- We added a modular parcel coalescing system allowing to combine several parcels into larger messages. This reduces latencies introduced by the communication layer.
- Added an experimental executors API allowing to use different scheduling policies for different parts of the code. This API has been modelled after the Standards proposal N3562. This API is bound to change in the future, though.
- Added minimal security support for localities which is enforced on the parcelport level. This support is preliminary and experimental and might change in the future.
- We created a parcelport using low level MPI functions. This is in support of legacy applications which are to be gradually ported and to support platforms where MPI is the only available portable networking layer.
- We added a preliminary and experimental implementation of a tuple-space object which exposes an interface similar to such systems described in the literature (see for instance The Linda Coordination Language).
Bug fixes (closed tickets)¶
Here is a list of the important tickets we closed for this release. This is again a very long list of newly implemented features and fixed issues.
- Issue #806 - make (all) in examples folder does nothing
- Issue #805 - Adding the introduction and fixing DOCBOOK dependencies for Windows use
- Issue #804 - Add stackless (non-suspendable) thread type
- Issue #803 - Create proper serialization support functions for util::tuple
- Issue #800 - Add possibility to disable array optimizations during serialization
- Issue #798 - HPX_LIMIT does not work for local dataflow
- Issue #797 - Create a parcelport which uses MPI
- Issue #796 - Problem with Large Numbers of Threads
- Issue #793 - Changing dataflow test case to hang consistently
- Issue #792 - CMake Error
- Issue #791 - Problems with local::dataflow
- Issue #790 - wait_for() doesn’t compile
- Issue #789 - HPX with Intel compiler segfaults
- Issue #788 - Intel compiler support
- Issue #787 - Fixed SFINAEd specializations
- Issue #786 - Memory issues during benchmarking.
- Issue #785 - Create an API allowing to register external threads with HPX
- Issue #784 - util::plugin is throwing an error when a symbol is not found
- Issue #783 - How does hpx:bind work?
- Issue #782 - Added quotes around STRING REPLACE potentially empty arguments
- Issue #781 - Make sure no exceptions propagate into the thread manager
- Issue #780 - Allow arithmetics performance counters to expand its parameters
- Issue #779 - Test case for 778
- Issue #778 - Swapping futures segfaults
- Issue #777 - hpx::lcos::details::when_xxx don’t restore completion handlers
- Issue #776 - Compiler chokes on dataflow overload with launch policy
- Issue #775 - Runtime error with local dataflow (copying futures?)
- Issue #774 - Using local dataflow without explicit namespace
- Issue #773 - Local dataflow with unwrap: functor operators need to be const
- Issue #772 - Allow (remote) actions to return a future
- Issue #771 - Setting HPX_LIMIT gives huge boost MPL errors
- Issue #770 - Add launch policy to (local) dataflow
- Issue #769 - Make compile time configuration information available
- Issue #768 - Const correctness problem in local dataflow
- Issue #767 - Add launch policies to async
- Issue #766 - Mark data structures for optimized (array based) serialization
- Issue #765 - Align hpx::any with N3508: Any Library Proposal (Revision 2)
- Issue #764 - Align hpx::future with newest N3558: A Standardized Representation of Asynchronous Operations
- Issue #762 - added a human readable output for the ping pong example
- Issue #761 - Ambiguous typename when constructing derived component
- Issue #760 - Simple components can not be derived
- Issue #759 - make install doesn’t give a complete install
- Issue #758 - Stack overflow when using locking_hook<>
- Issue #757 - copy paste error; unsupported function overloading
- Issue #756 - GTCX runtime issue in Gordon
- Issue #755 - Papi counters don’t work with reset and evaluate API’s
- Issue #753 - cmake bugfix and improved component action docs
- Issue #752 - hpx simple component docs
- Issue #750 - Add hpx::util::any
- Issue #749 - Thread phase counter is not reset
- Issue #748 - Memory performance counter are not registered
- Issue #747 - Create performance counters exposing arithmetic operations
- Issue #745 - apply_callback needs to invoke callback when applied locally
- Issue #744 - CMake fixes
- Issue #743 - Problem Building github version of HPX
- Issue #742 - Remove HPX_STD_BIND
- Issue #741 - assertion ‘px != 0’ failed: HPX(assertion_failure) for low numbers of OS threads
- Issue #739 - Performance counters do not count to the end of the program or evalution
- Issue #738 - Dedicated AGAS server runs don’t work; console ignores -a option.
- Issue #737 - Missing bind overloads
- Issue #736 - Performance counter wildcards do not always work
- Issue #735 - Create native ibverbs parcelport based on rdma operations
- Issue #734 - Threads stolen performance counter total is incorrect
- Issue #733 - Test benchmarks need to be checked and fixed
- Issue #732 - Build fails with Mac, using mac ports clang-3.3 on latest git branch
- Issue #731 - Add global start/stop API for performance counters
- Issue #730 - Performance counter values are apparently incorrect
- Issue #729 - Unhandled switch
- Issue #728 - Serialization of hpx::util::function between two localities causes seg faults
- Issue #727 - Memory counters on Mac OS X
- Issue #725 - Restore original thread priority on resume
- Issue #724 - Performance benchmarks do not depend on main HPX libraries
- Issue #723 - [teletype]–hpx:nodes=``cat $PBS_NODEFILE`` works; –hpx:nodefile=$PBS_NODEFILE does not.[c++]
- Issue #722 - Fix binding const member functions as actions
- Issue #719 - Create performance counter exposing compression ratio
- Issue #718 - Add possibility to compress parcel data
- Issue #717 - strip_credit_from_gid has misleading semantics
- Issue #716 - Non-option arguments to programs run using
pbsdsh
must be before--hpx:nodes
, contrary to directions - Issue #715 - Re-thrown exceptions should retain the original call site
- Issue #714 - failed assertion in debug mode
- Issue #713 - Add performance counters monitoring connection caches
- Issue #712 - Adjust parcel related performance counters to be connection type specific
- Issue #711 - configuration failure
- Issue #710 - Error “timed out while trying to find room in the connection cache” when trying to start multiple localities on a single computer
- Issue #709 - Add new thread state ‘staged’ referring to task descriptions
- Issue #708 - Detect/mitigate bad non-system installs of GCC on Redhat systems
- Issue #707 - Many examples do not link with Git HEAD version
- Issue #706 -
hpx::init
removes portions of non-option command line arguments before last=
sign - Issue #705 - Create rolling average and median aggregating performance counters
- Issue #704 - Create performance counter to expose thread queue waiting time
- Issue #703 - Add support to HPX build system to find librcrtool.a and related headers
- Issue #699 - Generalize instrumentation support
- Issue #698 - compilation failure with hwloc absent
- Issue #697 - Performance counter counts should be zero indexed
- Issue #696 - Distributed problem
- Issue #695 - Bad perf counter time printed
- Issue #693 -
--help
doesn’t print component specific command line options - Issue #692 - SLURM support broken
- Issue #691 - exception while executing any application linked with hwloc
- Issue #690 - thread_id_test and thread_launcher_test failing
- Issue #689 - Make the buildbots use hwloc
- Issue #687 - compilation error fix (hwloc_topology)
- Issue #686 - Linker Error for Applications
- Issue #684 - Pinning of service thread fails when number of worker threads equals the number of cores
- Issue #682 - Add performance counters exposing number of stolen threads
- Issue #681 - Add apply_continue for asynchronous chaining of actions
- Issue #679 - Remove obsolete async_callback API functions
- Issue #678 - Add new API for setting/triggering LCOs
- Issue #677 - Add async_continue for true continuation style actions
- Issue #676 - Buildbot for gcc 4.4 broken
- Issue #675 - Partial preprocessing broken
- Issue #674 - HPX segfaults when built with gcc 4.7
- Issue #673 -
use_guard_pages
has inconsistent preprocessor guards - Issue #672 - External build breaks if library path has spaces
- Issue #671 - release tarballs are tarbombs
- Issue #670 - CMake won’t find Boost headers in layout=versioned install
- Issue #669 - Links in docs to source files broken if not installed
- Issue #667 - Not reading ini file properly
- Issue #664 - Adapt new meanings of ‘const’ and ‘mutable’
- Issue #661 - Implement BTL Parcel port
- Issue #655 - Make HPX work with the “decltype” result_of
- Issue #647 - documentation for specifying the number of high priority
threads
--hpx:high-priority-threads
- Issue #643 - Error parsing host file
- Issue #642 - HWLoc issue with TAU
- Issue #639 - Logging potentially suspends a running thread
- Issue #634 - Improve error reporting from parcel layer
- Issue #627 - Add tests for async and apply overloads that accept regular C++ functions
- Issue #626 - hpx/future.hpp header
- Issue #601 - Intel support
- Issue #557 - Remove action codes
- Issue #531 - AGAS request and response classes should use switch statements
- Issue #529 - Investigate the state of hwloc support
- Issue #526 - Make HPX aware of hyper-threading
- Issue #518 - Create facilities allowing to use plain arrays as action arguments
- Issue #473 - hwloc thread binding is broken on CPUs with hyperthreading
- Issue #383 - Change result type detection for hpx::util::bind to use result_of protocol
- Issue #341 - Consolidate route code
- Issue #219 - Only copy arguments into actions once
- Issue #177 - Implement distributed AGAS
- Issue #43 - Support for Darwin (Xcode + Clang)
HPX V0.9.5 (Jan 16, 2013)¶
We have had over 1000 commits since the last release and we have closed roughly 150 tickets (bugs, feature requests, etc.).
General changes¶
This release is continuing along the lines of code and API consolidation, and overall usability inprovements. We dedicated much attention to performance and we were able to significantly improve the threading and networking subsystems.
We successfully ported HPX to the Android platform. HPX applications now not only can run on mobile devices, but we support heterogeneous applications running across architecture boundaries. At the Supercomputing Conference 2012 we demonstrated connecting Android tablets to simulations running on a Linux cluster. The Android tablet was used to query performance counters from the Linux simulation and to steer its parameters.
We successfully ported HPX to Mac OSX (using the Clang compiler). Thanks to Pyry Jahkola for contributing the corresponding patches. Please see the section How to install HPX on OS X (Mac) for more details.
We made a special effort to make HPX usable in highly concurrent use cases. Many
of the HPX API functions which possibly take longer than 100 microseconds to
execute now can be invoked asynchronously. We added uniform support for
composing futures which simplifies to write asynchronous code. HPX actions
(function objects encapsulating possibly concurrent remote function invocations)
are now well integrated with all other API facilities such like hpx::bind
.
All of the API has been aligned as much as possible with established paradigms.
HPX now mirrors many of the facilities as defined in the C++11 Standard, such as
hpx::thread
, hpx::function
, hpx::future
, etc.
A lot of work has been put into improving the documentation. Many of the API functions are documented now, concepts are explained in detail, and examples are better described than before. The new documentation index enables finding information with lesser effort.
This is the first release of HPX we perform after the move to Github This step has enabled a wider participation from the community and further encourages us in our decision to release HPX as a true open source library (HPX is licensed under the very liberal Boost Software License).
Bug fixes (closed tickets)¶
Here is a list of the important tickets we closed for this release. This is by far the longest list of newly implemented features and fixed issues for any of HPX’ releases so far.
- Issue #666 - Segfault on calling hpx::finalize twice
- Issue #665 - Adding declaration num_of_cores
- Issue #662 - pkgconfig is building wrong
- Issue #660 - Need uninterrupt function
- Issue #659 - Move our logging library into a different namespace
- Issue #658 - Dynamic performance counter types are broken
- Issue #657 - HPX v0.9.5 (RC1) hello_world example segfaulting
- Issue #656 - Define the affinity of parcel-pool, io-pool, and timer-pool threads
- Issue #654 - Integrate the Boost auto_index tool with documentation
- Issue #653 - Make HPX build on OS X + Clang + libc++
- Issue #651 - Add fine-grained control for thread pinning
- Issue #650 - Command line no error message when using -hpx:(anything)
- Issue #645 - Command line aliases don’t work in [teletype]``@file``[c++]
- Issue #644 - Terminated threads are not always properly cleaned up
- Issue #640 -
future_data<T>::set_on_completed_
used without locks - Issue #638 - hpx build with intel compilers fails on linux
- Issue #637 - –copy-dt-needed-entries breaks with gold
- Issue #635 - Boost V1.53 will add Boost.Lockfree and Boost.Atomic
- Issue #633 - Re-add examples to final 0.9.5 release
- Issue #632 - Example
thread_aware_timer
is broken - Issue #631 - FFT application throws error in parcellayer
- Issue #630 - Event synchronization example is broken
- Issue #629 - Waiting on futures hangs
- Issue #628 - Add an
HPX_ALWAYS_ASSERT
macro - Issue #625 - Port coroutines context switch benchmark
- Issue #621 - New INI section for stack sizes
- Issue #618 - pkg_config support does not work with a HPX debug build
- Issue #617 - hpx/external/logging/boost/logging/detail/cache_before_init.hpp:139:67: error: ‘get_thread_id’ was not declared in this scope
- Issue #616 - Change wait_xxx not to use locking
- Issue #615 - Revert visibility ‘fix’ (fb0b6b8245dad1127b0c25ebafd9386b3945cca9)
- Issue #614 - Fix Dataflow linker error
- Issue #613 - find_here should throw an exception on failure
- Issue #612 - Thread phase doesn’t show up in debug mode
- Issue #611 - Make stack guard pages configurable at runtime (initialization time)
- Issue #610 - Co-Locate Components
- Issue #609 - future_overhead
- Issue #608 -
--hpx:list-counter-infos
problem - Issue #607 - Update Boost.Context based backend for coroutines
- Issue #606 - 1d_wave_equation is not working
- Issue #605 - Any C++ function that has serializable arguments and a serializable return type should be remotable
- Issue #604 - Connecting localities isn’t working anymore
- Issue #603 - Do not verify any ini entries read from a file
- Issue #602 - Rename argument_size to type_size/ added implementation to get parcel size
- Issue #599 - Enable locality specific command line options
- Issue #598 - Need an API that accesses the performance counter reporting the system uptime
- Issue #597 - compiling on ranger
- Issue #595 - I need a place to store data in a thread self pointer
- Issue #594 - 32/64 interoperability
- Issue #593 - Warn if logging is disabled at compile time but requested at runtime
- Issue #592 - Add optional argument value to
--hpx:list-counters
and--hpx:list-counter-infos
- Issue #591 - Allow for wildcards in performance counter names specified
with
--hpx:print-counter
- Issue #590 - Local promise semantic differences
- Issue #589 - Create API to query performance counter names
- Issue #587 - Add get_num_localities and get_num_threads to AGAS API
- Issue #586 - Adjust local AGAS cache size based on number of localities
- Issue #585 - Error while using counters in HPX
- Issue #584 - counting argument size of actions, initial pass.
- Issue #581 - Remove
RemoteResult
template parameter forfuture<>
- Issue #580 - Add possibility to hook into actions
- Issue #578 - Use angle brackets in HPX error dumps
- Issue #576 - Exception incorrectly thrown when
--help
is used - Issue #575 - HPX(bad_component_type) with gcc 4.7.2 and boost 1.51
- Issue #574 -
--hpx:connect
command line parameter not working correctly - Issue #571 -
hpx::wait()
(callback version) should pass the future to the callback function - Issue #570 -
hpx::wait
should operate onboost::arrays
andstd::lists
- Issue #569 - Add a logging sink for Android
- Issue #568 - 2-argument version of
HPX_DEFINE_COMPONENT_ACTION
- Issue #567 - Connecting to a running HPX application works only once
- Issue #565 - HPX doesn’t shutdown properly
- Issue #564 - Partial preprocessing of new component creation interface
- Issue #563 - Add
hpx::start
/hpx::stop
to avoid blocking main thread - Issue #562 - All command line arguments swallowed by hpx
- Issue #561 - Boost.Tuple is not move aware
- Issue #558 -
boost::shared_ptr<>
style semantics/syntax for client classes - Issue #556 - Creation of partially preprocessed headers should be enabled for Boost newer than V1.50
- Issue #555 -
BOOST_FORCEINLINE
does not name a type - Issue #554 - Possible race condition in thread
get_id()
- Issue #552 - Move enable client_base
- Issue #550 - Add stack size category ‘huge’
- Issue #549 - ShenEOS run seg-faults on single or distributed runs
- Issue #545 -
AUTOGLOB
broken for add_hpx_component - Issue #542 - FindHPX_HDF5 still searches multiple times
- Issue #541 - Quotes around application name in hpx::init
- Issue #539 - Race conditition occuring with new lightweight threads
- Issue #535 - hpx_run_tests.py exits with no error code when tests are missing
- Issue #530 - Thread description(<unknown>) in logs
- Issue #523 - Make thread objects more lightweight
- Issue #521 -
hpx::error_code
is not usable for lightweight error handling - Issue #520 - Add full user environment to HPX logs
- Issue #519 - Build succeeds, running fails
- Issue #517 - Add a guard page to linux coroutine stacks
- Issue #516 - hpx::thread::detach suspends while holding locks, leads to hang in debug
- Issue #514 - Preprocessed headers for <hpx/apply.hpp> don’t compile
- Issue #513 - Buildbot configuration problem
- Issue #512 - Implement action based stack size customization
- Issue #511 - Move action priority into a separate type trait
- Issue #510 - trunk broken
- Issue #507 - no matching function for call to
boost::scoped_ptr<hpx::threads::topology>::scoped_ptr(hpx::threads::linux_topology*)
- Issue #505 - undefined_symbol regression test currently failing
- Issue #502 - Adding OpenCL and OCLM support to HPX for Windows and Linux
- Issue #501 - find_package(HPX) sets cmake output variables
- Issue #500 - wait_any/wait_all are badly named
- Issue #499 - Add support for disabling pbs support in pbs runs
- Issue #498 - Error during no-cache runs
- Issue #496 - Add partial preprocessing support to cmake
- Issue #495 - Support HPX modules exporting startup/shutdown functions only
- Issue #494 - Allow modules to specify when to run startup/shutdown functions
- Issue #493 - Avoid constructing a string in make_success_code
- Issue #492 - Performance counter creation is no longer synchronized at startup
- Issue #491 - Performance counter creation is no longer synchronized at startup
- Issue #490 - Sheneos on_completed_bulk seg fault in distributed
- Issue #489 - compiling issue with g++44
- Issue #488 - Adding OpenCL and OCLM support to HPX for the MSVC platform
- Issue #487 - FindHPX.cmake problems
- Issue #485 - Change distributing_factory and binpacking_factory to use bulk creation
- Issue #484 - Change
HPX_DONT_USE_PREPROCESSED_FILES
toHPX_USE_PREPROCESSED_FILES
- Issue #483 - Memory counter for Windows
- Issue #479 - strange errors appear when requesting performance counters on multiple nodes
- Issue #477 - Create (global) timer for multi-threaded measurements
- Issue #472 - Add partial preprocessing using Wave
- Issue #471 - Segfault stack traces don’t show up in release
- Issue #468 - External projects need to link with internal components
- Issue #462 - Startup/shutdown functions are called more than once
- Issue #458 - Consolidate hpx::util::high_resolution_timer and
hpx::util::high_resolution_clock
- Issue #457 - index out of bounds in
allgather_and_gate
on 4 cores or more - Issue #448 - Make HPX compile with clang
- Issue #447 - ‘make tests’ should execute tests on local installation
- Issue #446 - Remove SVN-related code from the codebase
- Issue #444 - race condition in smp
- Issue #441 - Patched Boost.Serialization headers should only be installed if needed
- Issue #439 - Components using
HPX_REGISTER_STARTUP_MODULE
fail to compile with MSVC - Issue #436 - Verify that no locks are being held while threads are suspended
- Issue #435 - Installing HPX should not clobber existing Boost installation
- Issue #434 - Logging external component failed (Boost 1.50)
- Issue #433 - Runtime crash when building all examples
- Issue #432 - Dataflow hangs on 512 cores/64 nodes
- Issue #430 - Problem with distributing factory
- Issue #424 - File paths referring to XSL-files need to be properly escaped
- Issue #417 - Make dataflow LCOs work out of the box by using partial preprocessing
- Issue #413 - hpx_svnversion.py fails on Windows
- Issue #412 - Make hpx::error_code equivalent to hpx::exception
- Issue #398 - HPX clobbers out-of-tree application specific CMake
variables (specifically
CMAKE_BUILD_TYPE
) - Issue #394 - Remove code generating random port numbers for network
- Issue #378 - ShenEOS scaling issues
- Issue #354 - Create a coroutines wrapper for Boost.Context
- Issue #349 - Commandline option
--localities=N/-lN
should be necessary only on AGAS locality - Issue #334 - Add auto_index support to cmake based documentation toolchain
- Issue #318 - Network benchmarks
- Issue #317 - Implement network performance counters
- Issue #310 - Duplicate logging entries
- Issue #230 - Add compile time option to disable thread debugging info
- Issue #171 - Add an INI option to turn off deadlock detection independently of logging
- Issue #170 - OSHL internal counters are incorrect
- Issue #103 - Better diagnostics for multiple component/action registerations under the same name
- Issue #48 - Support for Darwin (Xcode + Clang)
- Issue #21 - Build fails with GCC 4.6
HPX V0.9.0 (Jul 5, 2012)¶
We have had roughly 800 commits since the last release and we have closed approximately 80 tickets (bugs, feature requests, etc.).
General changes¶
Significant improvements made to the usability of HPX in large-scale, distributed environments.
Renamed
hpx::lcos::packaged_task
tohpx::lcos::packaged_action
to reflect the semantic differences to a packaged_task as defined by the C++11 Standard.HPX now exposes
hpx::thread
which is compliant to the C++11 std::thread type except that it (purely locally) represents an HPX thread. This new type does not expose any of the remote capabilities of the underlying HPX-thread implementation.The type
hpx::lcos::future
is now compliant to the C++11 std::future<> type. This type can be used to synchronize both, local and remote operations. In both cases the control flow will ‘return’ to the future in order to trigger any continuation.The types
hpx::lcos::local::promise
andhpx::lcos::local::packaged_task
are now compliant to the C++11std::promise<>
andstd::packaged_task<>
types. These can be used to create a future representing local work only. Use the typeshpx::lcos::promise
andhpx::lcos::packaged_action
to wrap any (possibly remote) action into a future.hpx::thread
andhpx::lcos::future
are now cancelable.Added support for sequential and logic composition of
hpx::lcos::future
s. The member functionhpx::lcos::future::when
permits futures to be sequentially composed. The helper functionshpx::wait_all
,hpx::wait_any
, andhpx::wait_n
can be used to wait for more than one future at a time.HPX now exposes
hpx::apply
andhpx::async
as the preferred way of creating (or invoking) any deferred work. These functions are usable with various types of functions, function objects, and actions and provide a uniform way to spawn deferred tasks.HPX now utilizes
hpx::util::bind
to (partially) bind local functions and function objects, and also actions. Remote bound actions can have placeholders as well.HPX continuations are now fully polymorphic. The class
hpx::actions::forwarding_continuation
is an example of how the user can write is own types of continuations. It can be used to execute any function as an continuation of a particular action.Reworked the action invocation API to be fully conformant to normal functions. Actions can now be invoked using
hpx::apply
,hpx::async
, or using theoperator()
implemented on actions. Actions themselves can now be cheaply instantiated as they do not have any members anymore.Reworked the lazy action invocation API. Actions can now be directly bound using
hpx::util::bind
by passing an action instance as the first argument.A minimal HPX program now looks like this:
#include <hpx/hpx_init.hpp> int hpx_main() { return hpx::finalize(); } int main() { return hpx::init(); }
This removes the immediate dependency on the Boost.Program Options library.
Note
This minimal version of an HPX program does not support any of the default command line arguments (such as –help, or command line options related to PBS). It is suggested to always pass
argc
andargv
to HPX as shown in the example below.In order to support those, but still not to depend on Boost.Program Options, the minimal program can be written as:
#include <hpx/hpx_init.hpp> // The arguments for hpx_main can be left off, which very similar to the // behavior of ``main()`` as defined by C++. int hpx_main(int argc, char* argv[]) { return hpx::finalize(); } int main(int argc, char* argv[]) { return hpx::init(argc, argv); }
Added performance counters exposing the number of component instances which are alive on a given locality.
Added performance counters exposing then number of messages sent and received, the number of parcels sent and received, the number of bytes sent and received, the overall time required to send and receive data, and the overall time required to serialize and deserialize the data.
Added a new component:
hpx::components::binpacking_factory
which is equivalent to the existinghpx::components::distributing_factory
component, except that it equalizes the overall population of the components to create. It exposes two factory methods, one based on the number of existing instances of the component type to create, and one based on an arbitrary performance counter which will be queried for all relevant localities.Added API functions allowing to access elements of the diagnostic information embedded in the given exception:
hpx::get_locality_id
,hpx::get_host_name
,hpx::get_process_id
,hpx::get_function_name
,hpx::get_file_name
,hpx::get_line_number
,hpx::get_os_thread
,hpx::get_thread_id
, andhpx::get_thread_description
.
Bug fixes (closed tickets)¶
Here is a list of the important tickets we closed for this release:
- Issue #71 - GIDs that are not serialized via
handle_gid<>
should raise an exception - Issue #105 - Allow for
hpx::util::function
s to be registered in the AGAS symbolic namespace - Issue #107 - Nasty threadmanger race condition (reproducible in sheneos_test)
- Issue #108 - Add millisecond resolution to HPX logs on Linux
- Issue #110 - Shutdown hang in distributed with release build
- Issue #116 - Don’t use TSS for the applier and runtime pointers
- Issue #162 - Move local synchronous execution shortcut from hpx::function to the applier
- Issue #172 - Cache sources in CMake and check if they change manually
- Issue #178 - Add an INI option to turn off ranged-based AGAS caching
- Issue #187 - Support for disabling performance counter deployment
- Issue #202 - Support for sending performance counter data to a specific file
- Issue #218 - boost.coroutines allows different stack sizes, but stack pool is unaware of this
- Issue #231 - Implement movable
boost::bind
- Issue #232 - Implement movable
boost::function
- Issue #236 - Allow binding
hpx::util::function
to actions - Issue #239 - Replace
hpx::function
withhpx::util::function
- Issue #240 - Can’t specify RemoteResult with lcos::async
- Issue #242 - REGISTER_TEMPLATE support for plain actions
- Issue #243 -
handle_gid<>
support forhpx::util::function
- Issue #245 -
*_c_cache code
throws an exception if the queried GID is not in the local cache - Issue #246 - Undefined references in dataflow/adaptive1d example
- Issue #252 - Problems configuring sheneos with CMake
- Issue #254 - Lifetime of components doesn’t end when client goes out of scope
- Issue #259 - CMake does not detect that MSVC10 has lambdas
- Issue #260 - io_service_pool segfault
- Issue #261 - Late parcel executed outside of pxthread
- Issue #263 - Cannot select allocator with CMake
- Issue #264 - Fix allocator select
- Issue #267 - Runtime error for hello_world
- Issue #269 - pthread_affinity_np test fails to compile
- Issue #270 - Compiler noise due to -Wcast-qual
- Issue #275 - Problem with configuration tests/include paths on Gentoo
- Issue #325 - Sheneos is 200-400 times slower than the fortran equivalent
- Issue #331 -
hpx::init
andhpx_main()
should not depend on program_options - Issue #333 - Add doxygen support to CMake for doc toolchain
- Issue #340 - Performance counters for parcels
- Issue #346 - Component loading error when running hello_world in distributed on MSVC2010
- Issue #362 - Missing initializer error
- Issue #363 - Parcel port serialization error
- Issue #366 - Parcel buffering leads to types incompatible exception
- Issue #368 - Scalable alternative to rand() needed for HPX
- Issue #369 - IB over IP is substantially slower than just using standard TCP/IP
- Issue #374 -
hpx::lcos::wait
should work with dataflows and arbitrary classes meeting the future interface - Issue #375 - Conflicting/ambiguous overloads of
hpx::lcos::wait
- Issue #376 - Find_HPX.cmake should set CMake variable HPX_FOUND for out of tree builds
- Issue #377 - ShenEOS interpolate bulk and interpolate_one_bulk are broken
- Issue #379 - Add support for distributed runs under SLURM
- Issue #382 - _Unwind_Word not declared in boost.backtrace
- Issue #387 - Doxygen should look only at list of specified files
- Issue #388 - Running
make install
on an out-of-tree application is broken - Issue #391 - Out-of-tree application segfaults when running in qsub
- Issue #392 - Remove HPX_NO_INSTALL option from cmake build system
- Issue #396 - Pragma related warnings when compiling with older gcc versions
- Issue #399 - Out of tree component build problems
- Issue #400 - Out of source builds on Windows: linker should not receive compiler flags
- Issue #401 - Out of source builds on Windows: components need to be linked with hpx_serialization
- Issue #404 - gfortran fails to link automatically when fortran files are present
- Issue #405 - Inability to specify linking order for external libraries
- Issue #406 - Adapt action limits such that dataflow applications work without additional defines
- Issue #415 -
locality_results
is not a member ofhpx::components::server
- Issue #425 - Breaking changes to
traits::*result
wrtstd::vector<id_type>
- Issue #426 - AUTOGLOB needs to be updated to support fortran
HPX V0.8.1 (Apr 21, 2012)¶
This is a point release including important bug fixes for HPX V0.8.0 (Mar 23, 2012).
General changes¶
- HPX does not need to be installed anymore to be functional.
Bug fixes (closed tickets)¶
Here is a list of the important tickets we closed for this point release:
- Issue #295 - Don’t require install path to be known at compile time.
- Issue #371 - Add hpx iostreams to standard build.
- Issue #384 - Fix compilation with GCC 4.7.
- Issue #390 - Remove keep_factory_alive startup call from ShenEOS; add shutdown call to H5close.
- Issue #393 - Thread affinity control is broken.
Bug fixes (commits)¶
Here is a list of the important commits included in this point release:
- r7642 - External: Fix backtrace memory violation.
- r7775 - Components: Fix symbol visibility bug with component startup
- providers. This prevents one components providers from overriding another components.
- r7778 - Components: Fix startup/shutdown provider shadowing issues.
HPX V0.8.0 (Mar 23, 2012)¶
We have had roughly 1000 commits since the last release and we have closed approximately 70 tickets (bugs, feature requests, etc.).
General changes¶
- Improved PBS support, allowing for arbitrary naming schemes of node-hostnames.
- Finished verification of the reference counting framework.
- Implemented decrement merging logic to optimize the distributed reference counting system.
- Restructured the LCO framework. Renamed
hpx::lcos::eager_future<>
andhpx::lcos::lazy_future<>
intohpx::lcos::packaged_task
andhpx::lcos::deferred_packaged_task
. Splithpx::lcos::promise
intohpx::lcos::packaged_task
andhpx::lcos::future
. Added ‘local’ futures (in namespacehpx::lcos::local
). - Improved the general performance of local and remote action invocations. This (under certain circumstances) drastically reduces the number of copies created for each of the parameters and return values.
- Reworked the performance counter framework. Performance counters are now created only when needed, which reduces the overall resource requirements. The new framework allows for much more flexible creation and management of performance counters. The new sine example application demonstrates some of the capabilities of the new infrastructure.
- Added a buildbot-based continuous build system which gives instant, automated feedback on each commit to SVN.
- Added more automated tests to verify proper functioning of HPX.
- Started to create documentation for HPX and its API.
- Added documentation toolchain to the build system.
- Added dataflow LCO.
- Changed default HPX command line options to have
hpx:
prefix. For instance, the former option--threads
is now--hpx:threads
. This has been done to make ambiguities with possible application specific command line options as unlikely as possible. See the section HPX Command Line Options for a full list of available options. - Added the possibility to define command line aliases. The former short (one-letter) command line options have been predefined as aliases for backwards compatibility. See the section HPX Command Line Options for a detailed description of command line option aliasing.
- Network connections are now cached based on the connected host. The number of simultaneous connections to a particular host is now limited. Parcels are buffered and bundled if all connections are in use.
- Added more refined thread affinity control. This is based on the external library Portable Hardware Locality (HWLOC).
- Improved support for Windows builds with CMake.
- Added support for components to register their own command line options.
- Added the possibility to register custom startup/shutdown functions for any component. These functions are guaranteed to be executed by an HPX thread.
- Added two new experimental thread schedulers: hierarchy_scheduler and
periodic_priority_scheduler. These can be activated by using the command line
options
--hpx:queuing
=hierarchy
or--hpx:queuing
=periodic
.
Example applications¶
- Graph500 performance benchmark (thanks to Matthew Anderson for contributing this application).
- GTC (Gyrokinetic Toroidal Code): a skeleton for particle in cell type codes.
- Random Memory Access: an example demonstrating random memory accesses in a large array
- ShenEOS example, demonstrating partitioning of large read-only data structures and exposing an interpolation API.
- Sine performance counter demo.
- Accumulator examples demonstrating how to write and use HPX components.
- Quickstart examples (like hello_world, fibonacci, quicksort, factorial, etc.) demonstrating simple HPX concepts which introduce some of the concepts in HPX.
- Load balancing and work stealing demos.
API changes¶
Moved all local LCOs into a separate namespace
hpx::lcos::local
(for instance,hpx::lcos::local_mutex
is nowhpx::lcos::local::mutex
).Replaced
hpx::actions::function
withhpx::util::function
. Cleaned up related code.Removed
hpx::traits::handle_gid
and moved handling of global reference counts into the corresponding serialization code.Changed terminology:
prefix
is now calledlocality_id
, renamed the corresponding API functions (such ashpx::get_prefix
, which is now calledhpx::get_locality_id
).Adding
hpx::find_remote_localities
, andhpx::get_num_localities
.Changed performance counter naming scheme to make it more bash friendly. The new performance counter naming scheme is now
/object{parentname#parentindex/instance#index}/counter#parameters
Added
hpx::get_worker_thread_num
replacinghpx::threadmanager_base::get_thread_num
.Renamed
hpx::get_num_os_threads
tohpx::get_os_threads_count
.Added
hpx::threads::get_thread_count
.Restructured the Futures sub-system, renaming types in accordance with the terminology used by the C++11 ISO standard.
Bug fixes (closed tickets)¶
Here is a list of the important tickets we closed for this release:
- Issue #31 - Specialize handle_gid<> for examples and tests
- Issue #72 - Fix AGAS reference counting
- Issue #104 - heartbeat throws an exception when decrefing the performance counter it’s watching
- Issue #111 - throttle causes an exception on the target application
- Issue #142 - One failed component loading causes an unrelated component to fail
- Issue #165 - Remote exception propagation bug in AGAS reference counting test
- Issue #186 - Test credit exhaustion/splitting (e.g. prepare_gid and symbol NS)
- Issue #188 - Implement remaining AGAS reference counting test cases
- Issue #258 - No type checking of GIDs in stubs classes
- Issue #271 - Seg fault/shared pointer assertion in distributed code
- Issue #281 - CMake options need descriptive text
- Issue #283 - AGAS caching broken (gva_cache needs to be rewritten with ICL)
- Issue #285 - HPX_INSTALL root directory not the same as CMAKE_INSTALL_PREFIX
- Issue #286 - New segfault in dataflow applications
- Issue #289 - Exceptions should only be logged if not handled
- Issue #290 - c++11 tests failure
- Issue #293 - Build target for component libraries
- Issue #296 - Compilation error with Boost V1.49rc1
- Issue #298 - Illegal instructions on termination
- Issue #299 - gravity aborts with multiple threads
- Issue #301 - Build error with Boost trunk
- Issue #303 - Logging assertion failure in distributed runs
- Issue #304 - Exception ‘what’ strings are lost when exceptions from decode_parcel are reported
- Issue #306 - Performance counter user interface issues
- Issue #307 - Logging exception in distributed runs
- Issue #308 - Logging deadlocks in distributed
- Issue #309 - Reference counting test failures and exceptions
- Issue #311 - Merge AGAS remote_interface with the runtime_support object
- Issue #314 - Object tracking for id_types
- Issue #315 - Remove handle_gid and handle credit splitting in id_type serialization
- Issue #320 - applier::get_locality_id() should return an error value (or throw an exception)
- Issue #321 - Optimization for id_types which are never split should be restored
- Issue #322 - Command line processing ignored with Boost 1.47.0
- Issue #323 - Credit exhaustion causes object to stay alive
- Issue #324 - Duplicate exception messages
- Issue #326 - Integrate Quickbook with CMake
- Issue #329 - –help and –version should still work
- Issue #330 - Create pkg-config files
- Issue #337 - Improve usability of performance counter timestamps
- Issue #338 - Non-std exceptions deriving from std::exceptions in tfunc may be sliced
- Issue #339 - Decrease the number of send_pending_parcels threads
- Issue #343 - Dynamically setting the stack size doesn’t work
- Issue #351 - ‘make install’ does not update documents
- Issue #353 - Disable FIXMEs in the docs by default; add a doc developer CMake option to enable FIXMEs
- Issue #355 - ‘make’ doesn’t do anything after correct configuration
- Issue #356 - Don’t use
hpx::util::static_
in topology code - Issue #359 - Infinite recursion in hpx::tuple serialization
- Issue #361 - Add compile time option to disable logging completely
- Issue #364 - Installation seriously broken in r7443
HPX V0.7.0 (Dec 12, 2011)¶
We have had roughly 1000 commits since the last release and we have closed approximately 120 tickets (bugs, feature requests, etc.).
General changes¶
- Completely removed code related to deprecated AGAS V1, started to work on AGAS V2.1.
- Started to clean up and streamline the exposed APIs (see ‘API changes’ below for more details).
- Revamped and unified performance counter framework, added a lot of new performance counter instances for monitoring of a diverse set of internal HPX parameters (queue lengths, access statistics, etc.).
- Improved general error handling and logging support.
- Fixed several race conditions, improved overall stability, decreased memory footprint, improved overall performance (major optimizations include native TLS support and ranged-based AGAS caching).
- Added support for running HPX applications with PBS.
- Many updates to the build system, added support for gcc 4.5.x and 4.6.x, added C++11 support.
- Many updates to default command line options.
- Added many tests, set up buildbot for continuous integration testing.
- Better shutdown handling of distributed applications.
Example applications¶
- quickstart/factorial and quickstart/fibonacci, future-recursive parallel algorithms.
- quickstart/hello_world, distributed hello world example.
- quickstart/rma, simple remote memory access example
- quickstart/quicksort, parallel quicksort implementation.
- gtc, gyrokinetic torodial code.
- bfs, breadth-first-search, example code for a graph application.
- sheneos, partitioning of large data sets.
- accumulator, simple component example.
- balancing/os_thread_num, balancing/px_thread_phase, examples demonstrating load balancing and work stealing.
API changes¶
- Added
hpx::find_all_localities
. - Added
hpx::terminate
for non-graceful termination of applications. - Added
hpx::lcos::async
functions for simpler asynchronous programming. - Added new AGAS interface for handling of symbolic namespace
(
hpx::agas::*
). - Renamed
hpx::components::wait
tohpx::lcos::wait
. - Renamed
hpx::lcos::future_value
tohpx::lcos::promise
. - Renamed
hpx::lcos::recursive_mutex
tohpx::lcos::local_recursive_mutex
,hpx::lcos::mutex
tohpx::lcos::local_mutex
- Removed support for Boost versions older than V1.38, recommended Boost version is now V1.47 and newer.
- Removed
hpx::process
(this will be replaced by a real process implementation in the future). - Removed non-functional LCO code (
hpx::lcos::dataflow
,hpx::lcos::thunk
,hpx::lcos::dataflow_variable
). - Removed deprecated
hpx::naming::full_address
.
Bug fixes (closed tickets)¶
Here is a list of the important tickets we closed for this release:
- Issue #28 - Integrate Windows/Linux CMake code for HPX core
- Issue #32 - hpx::cout() should be hpx::cout
- Issue #33 - AGAS V2 legacy client does not properly handle error_code
- Issue #60 - AGAS: allow for registerid to optionally take ownership of the gid
- Issue #62 - adaptive1d compilation failure in Fusion
- Issue #64 - Parcel subsystem doesn’t resolve domain names
- Issue #83 - No error handling if no console is available
- Issue #84 - No error handling if a hosted locality is treated as the bootstrap server
- Issue #90 - Add general commandline option -N
- Issue #91 - Add possibility to read command line arguments from file
- Issue #92 - Always log exceptions/errors to the log file
- Issue #93 - Log the command line/program name
- Issue #95 - Support for distributed launches
- Issue #97 - Attempt to create a bad component type in AMR examples
- Issue #100 - factorial and factorial_get examples trigger AGAS component type assertions
- Issue #101 - Segfault when hpx::process::here() is called in fibonacci2
- Issue #102 - unknown_component_address in int_object_semaphore_client
- Issue #114 - marduk raises assertion with default parameters
- Issue #115 - Logging messages for SMP runs (on the console) shouldn’t be buffered
- Issue #119 - marduk linking strategy breaks other applications
- Issue #121 - pbsdsh problem
- Issue #123 - marduk, dataflow and adaptive1d fail to build
- Issue #124 - Lower default preprocessing arity
- Issue #125 - Move hpx::detail::diagnostic_information out of the detail namespace
- Issue #126 - Test definitions for AGAS reference counting
- Issue #128 - Add averaging performance counter
- Issue #129 - Error with endian.hpp while building adaptive1d
- Issue #130 - Bad initialization of performance counters
- Issue #131 - Add global startup/shutdown functions to component modules
- Issue #132 - Avoid using auto_ptr
- Issue #133 - On Windows hpx.dll doesn’t get installed
- Issue #134 - HPX_LIBRARY does not reflect real library name (on Windows)
- Issue #135 - Add detection of unique_ptr to build system
- Issue #137 - Add command line option allowing to repeatedly evaluate performance counters
- Issue #139 - Logging is broken
- Issue #140 - CMake problem on windows
- Issue #141 - Move all non-component libraries into $PREFIX/lib/hpx
- Issue #143 - adaptive1d throws an exception with the default command line options
- Issue #146 - Early exception handling is broken
- Issue #147 - Sheneos doesn’t link on Linux
- Issue #149 - sheneos_test hangs
- Issue #154 - Compilation fails for r5661
- Issue #155 - Sine performance counters example chokes on chrono headers
- Issue #156 - Add build type to –version
- Issue #157 - Extend AGAS caching to store gid ranges
- Issue #158 - r5691 doesn’t compile
- Issue #160 - Re-add AGAS function for resolving a locality to its prefix
- Issue #168 - Managed components should be able to access their own GID
- Issue #169 - Rewrite AGAS future pool
- Issue #179 - Complete switch to request class for AGAS server interface
- Issue #182 - Sine performance counter is loaded by other examples
- Issue #185 - Write tests for symbol namespace reference counting
- Issue #191 - Assignment of read-only variable in point_geometry
- Issue #200 - Seg faults when querying performance counters
- Issue #204 - –ifnames and suffix stripping needs to be more generic
- Issue #205 - –list-* and –print-counter-* options do not work together and produce no warning
- Issue #207 - Implement decrement entry merging
- Issue #208 - Replace the spinlocks in AGAS with hpx::lcos::local_mutexes
- Issue #210 - Add an –ifprefix option
- Issue #214 - Performance test for PX-thread creation
- Issue #216 - VS2010 compilation
- Issue #222 - r6045 context_linux_x86.hpp
- Issue #223 - fibonacci hangs when changing the state of an active thread
- Issue #225 - Active threads end up in the FEB wait queue
- Issue #226 - VS Build Error for Accumulator Client
- Issue #228 - Move all traits into namespace hpx::traits
- Issue #229 - Invalid initialization of reference in thread_init_data
- Issue #235 - Invalid GID in iostreams
- Issue #238 - Demangle type names for the default implementation of get_action_name
- Issue #241 - C++11 support breaks GCC 4.5
- Issue #247 - Reference to temporary with GCC 4.4
- Issue #248 - Seg fault at shutdown with GCC 4.4
- Issue #253 - Default component action registration kills compiler
- Issue #272 - G++ unrecognized command line option
- Issue #273 - quicksort example doesn’t compile
- Issue #277 - Invalid CMake logic for Windows
About HPX¶
History¶
The development of High Performance ParalleX (HPX) began in 2007. At that time, Hartmut Kaiser became interested in the work done by the ParalleX group at the Center for Computation and Technology (CCT), a multi-disciplinary research institute at Louisiana State University (LSU). The ParalleX group was working to develop a new and experimental execution model for future high performance computing architectures. This model was christened ParalleX. The first implementations of ParalleX were crude, and many of those designs had to be discarded entirely. However, over time the team learned quite a bit about how to design a parallel, distributed runtime system which implements the concepts of ParalleX.
From the very beginning, this endeavour has been a group effort. In addition to a handful of interested researchers, there have always been graduate and undergraduate students participating in the discussions, design, and implementation of HPX. In 2011 we decided to formalize our collective research efforts by creating the STE||AR group (Systems Technology, Emergent Parallelism, and Algorithm Research). Over time, the team grew to include researchers around the country and the world. In 2014, the STE||AR Group was reorganized to become the international community it is today. This consortium of researchers aims to develop stable, sustainable, and scalable tools which will enable application developers to exploit the parallelism latent in the machines of today and tomorrow. Our goal of the HPX project is to create a high quality, freely available, open source implementation of ParalleX concepts for conventional and future systems by building a modular and standards conforming runtime system for SMP and distributed application environments. The API exposed by HPX is conformant to the interfaces defined by the C++11/14 ISO standard and adheres to the programming guidelines used by the Boost collection of C++ libraries. We steer the development of HPX with real world applications and aim to provide a smooth migration path for domain scientists.
To learn more about STE||AR and ParalleX, see People and Why HPX?.
People¶
The STE||AR Group (pronounced as stellar) stands for “Systems Technology, Emergent Parallelism, and Algorithm Research”. We are an international group of faculty, researchers, and students working at various institutions around the world. The goal of the STE||AR Group is to promote the development of scalable parallel applications by providing a community for ideas, a framework for collaboration, and a platform for communicating these concepts to the broader community.
Our work is focused on building technologies for scalable parallel applications. HPX, our general purpose C++ runtime system for parallel and distributed applications, is no exception. We use HPX for a broad range of scientific applications, helping scientists and developers to write code which scales better and shows better performance compared to more conventional programming models such as MPI.
HPX is based on ParalleX which is a new (and still experimental) parallel execution model aiming to overcome the limitations imposed by the current hardware and the techniques we use to write applications today. Our group focuses on two types of applications - those requiring excellent strong scaling, allowing for a dramatic reduction of execution time for fixed workloads and those needing highest level of sustained performance through massive parallelism. These applications are presently unable (through conventional practices) to effectively exploit a relatively small number of cores in a multi-core system. By extension, these application will not be able to exploit high-end exascale computing systems which are likely to employ hundreds of millions of such cores by the end of this decade.
Critical bottlenecks to the effective use of new generation high performance computing (HPC) systems include:
- Starvation: due to lack of usable application parallelism and means of managing it,
- Overhead: reduction to permit strong scalability, improve efficiency, and enable dynamic resource management,
- Latency: from remote access across system or to local memories,
- Contention: due to multicore chip I/O pins, memory banks, and system interconnects.
The ParalleX model has been devised to address these challenges by enabling a new computing dynamic through the application of message-driven computation in a global address space context with lightweight synchronization. The work on HPX is centered around implementing the concepts as defined by the ParalleX model. HPX is currently targeted at conventional machines, such as classical Linux based Beowulf clusters and SMP nodes.
We fully understand that the success of HPX (and ParalleX) is very much the result of the work of many people. To see a list of who is contributing see our tables below.
HPX contributors¶
Contributors to this document¶
Name | Institution | |
---|---|---|
Hartmut Kaiser | Center for Computation and Technology (CCT), Louisiana State University (LSU) | ![]() |
Thomas Heller | Department of Computer Science 3 - Computer Architecture, Friedrich-Alexander University Erlangen-Nuremberg (FAU) | ![]() |
Bryce Adelstein Lelbach | NVIDIA | ![]() |
Vinay C Amatya | Center for Computation and Technology (CCT), Louisiana State University (LSU) | ![]() |
Steven Brandt | Center for Computation and Technology (CCT), Louisiana State University (LSU) | ![]() |
Maciej Brodowicz | Center for Research in Extreme Scale Technologies (CREST), Indiana University (IU) | ![]() |
Adrian Serio | Center for Computation and Technology (CCT), Louisiana State University (LSU) | ![]() |
Acknowledgements¶
Thanks also to the following people who contributed directly or indirectly to the project through discussions, pull requests, documentation patches, etc.
- Jan Mełech, for adding automatic serialization of simple structs.
- Austin McCartney, for adding concept emulation of the Ranges TS bidirectional and random access iterator concepts.
- Marco Diers, reporting and fixing issues related PMIx.
- Maximilian Bremer, for reporting multiple issues and extending the component migration tests.
- Piotr Mikołajczyk, for his improvements and fixes to the set and count algorithms.
- Grant Rostig, for reporting several deficiencies on our web pages.
- Jakub Golinowski, for implementing an HPX backend for OpenCV and in the process improving documentation and reporting issues.
- Mikael Simberg (Swiss National Supercomputing Centre), for his tireless help cleaning up and maintaining HPX.
- Tianyi Zhang, for his work on HPXMP.
- Shahrzad Shirzad, for her contributions related to Phylanx.
- Christopher Ogle, for his contributions to the parallel algorithms.
- Surya Priy, for his work with statistic performance counters.
- Anushi Maheshwari, for her work on random number generation.
- Bruno Pitrus, for his work with parallel algorithms.
- Nikunj Gupta, for rewriting the implementation of
hpx_main.hpp
and for his fixes for tests. - Christopher Taylor, for his interest in HPX and the fixes he provided.
- Shoshana Jakobovits, for her work on the resource partitioner.
- Denis Blank, who re-wrote our unwrapped function to accept plain values arbitrary containers, and properly deal with nested futures.
- Ajai V. George, who implemented several of the parallel algorithms.
- Taeguk Kwon, who worked on implementing parallel algorithms as well as adapting the parallel algorithms to the Ranges TS.
- Zach Byerly (Louisiana State University (LSU)), who in his work developing applications on top of HPX opened tickets and contributed to the HPX examples.
- Daniel Estermann, for his work porting HPX to the Raspberry Pi.
- Alireza Kheirkhahan (Louisiana State University (LSU)), who built and administered our local cluster as well as his work in distributed IO.
- Abhimanyu Rawat, who worked on stack overflow detection.
- David Pfander, who improved signal handling in HPX, provided his optimization expertise, and worked on incorporating the Vc vectorization into HPX.
- Denis Demidov, who contributed his insights with VexCL.
- Khalid Hasanov, who contributed changes which allowed to run HPX on 64Bit power-pc architectures.
- Zahra Khatami (Louisiana State University (LSU)), who contributed the prefetching iterators and the persistent auto chunking executor parameters implementation.
- Marcin Copik, who worked on implementing GPU support for C++AMP and HCC. He also worked on implementing a HCC backend for HPX.Compute.
- Minh-Khanh Do, who contributed the implementation of several segmented algorithms.
- Bibek Wagle (Louisiana State University (LSU)), who worked on fixing and analyzing the performance of the parcel coalescing plugin in HPX.
- Lukas Troska, who reported several problems and contributed various test cases allowing to reproduce the corresponding issues.
- Andreas Schaefer, who worked on integrating his library (LibGeoDecomp) with HPX. He reported various problems and submitted several patches to fix issues allowing for a better integration with LibGeoDecomp.
- Satyaki Upadhyay, who contributed several examples to HPX.
- Brandon Cordes, who contributed several improvements to the inspect tool.
- Harris Brakmic, who contributed an extensive build system description for building HPX with Visual Studio.
- Parsa Amini (Louisiana State University (LSU)), who refactored and simplified the implementation of AGAS in HPX and who works on its implementation and optimization.
- Luis Martinez de Bartolome who implemented a build system extension for HPX integrating it with the Conan C/C++ package manager.
- Vinay C Amatya (Louisiana State University (LSU)), who contributed to the documentation and provided some of the HPX examples.
- Kevin Huck and Nick Chaimov (University of Oregon), who contributed the integration of APEX (Autonomic Performance Environment for eXascale) with HPX.
- Francisco Jose Tapia, who helped with implementing the parallel sort algorithm for HPX.
- Patrick Diehl, who worked on implementing CUDA support for our companion library targeting GPGPUs (HPXCL).
- Eric Lemanissier contributed fixes to allow compilation using the MingW toolchain.
- Nidhi Makhijani who helped cleaning up some enum consistencies in HPX and contributed to the resource manager used in the thread scheduling subsystem. She also worked on HPX in the context of the Google Summer of Code 2015.
- Larry Xiao, Devang Bacharwar, Marcin Copik, and Konstantin Kronfeldner who worked on HPX in the context of the Google Summer of Code program 2015.
- Daniel Bourgeois (Center for Computation and Technology (CCT)) who contributed to HPX the implementation of several parallel algorithms (as proposed by N4313).
- Anuj Sharma and Christopher Bross (Department of Computer Science 3 - Computer Architecture), who worked on HPX in the context of the Google Summer of Code program 2014.
- Martin Stumpf (Department of Computer Science 3 - Computer Architecture), who rebuilt our contiguous testing infrastructure (see the HPX Buildbot Website). Martin is also working on HPXCL (mainly all work related to OpenCL) and implementing an HPX backend for POCL, a portable computing language solution based on OpenCL.
- Grant Mercer (University of Nevada, Las Vegas), who helped creating many of the parallel algorithms (as proposed by N4313).
- Damond Howard (Louisiana State University (LSU)), who works on HPXCL (mainly all work related to CUDA).
- Christoph Junghans (Los Alamos National Lab), who helped making our buildsystem more portable.
- Antoine Tran Tan (Laboratoire de Recherche en Informatique, Paris), who worked on integrating HPX as a backend for NT2. He also contributed an implementation of an API similar to Fortran co-arrays on top of HPX.
- John Biddiscombe (Swiss National Supercomputing Centre), who helped with the BlueGene/Q port of HPX, implemented the parallel sort algorithm, and made several other contributions.
- Erik Schnetter (Perimeter Institute for Theoretical Physics), who greatly helped to make HPX more robust by submitting a large amount of problem reports, feature requests, and made several direct contributions.
- Mathias Gaunard (Metascale), who contributed several patches to reduce compile time warnings generated while compiling HPX.
- Andreas Buhr, who helped with improving our documentation, especially by suggesting some fixes for inconsistencies.
- Patricia Grubel (New Mexico State University), who contributed the description of the different HPX thread scheduler policies and is working on the performance analysis of our thread scheduling subsystem.
- Lars Viklund, whose wit, passion for testing, and love of odd architectures has been an amazing contribution to our team. He has also contributed platform specific patches for FreeBSD and MSVC12.
- Agustin Berge, who contributed patches fixing some very nasty hidden template meta-programming issues. He rewrote large parts of the API elements ensuring strict conformance with C++11/14.
- Anton Bikineev for contributing changes to make using
boost::lexical_cast
safer, he also contributed a thread safety fix to the iostreams module. He also contributed a complete rewrite of the serialization infrastructure replacing Boost.Serialization inside HPX. - Pyry Jahkola, who contributed the Mac OS build system and build documentation on how to build HPX using Clang and libc++.
- Mario Mulansky, who created an HPX backend for his Boost.Odeint library, and who submitted several test cases allowing us to reproduce and fix problems in HPX.
- Rekha Raj, who contributed changes to the description of the Windows build instructions.
- Jeremy Kemp how worked on an HPX OpenMP backend and added regression tests.
- Alex Nagelberg for his work on implementing a C wrapper API for HPX.
- Chen Guo, helvihartmann, Nicholas Pezolano, and John West who added and improved examples in HPX.
- Joseph Kleinhenz, Markus Elfring, Kirill Kropivyansky, Alexander Neundorf, Bryant Lam, and Alex Hirsch who improved our CMake.
- Praveen Velliengiri, Jean-Loup Tastet, Michael Levine, Aalekh Nigam, HadrienG2, Prayag Verma, and Avyav Kumar who improved the documentation.
- Jayesh Badwaik, J. F. Bastien, Christoph Garth, Christopher Hinz, Brandon Kohn, Mario Lang, Maikel Nadolski, pierrele, hendrx, Dekken, woodmeister123, xaguilar, Andrew Kemp, Dylan Stark, Matthew Anderson, Jeremy Wilke, Jiazheng Yuan, CyberDrudge, david8dixon, Maxwell Reeser, Raffaele Solcà, Marco Ippolito, Jules Pénuchot, and yurivict who contributed to the general improvement of HPX.
In addition to the people who worked directly with HPX development we would like to acknowledge the NSF, DoE, DARPA, Center for Computation and Technology (CCT), Department of Computer Science 3 - Computer Architecture, and Swiss National Supercomputing Centre who fund and support our work. We would also like to thank the following organizations for granting us allocations of their compute resources: LSU HPC, LONI, XSEDE, NERSC, and the Gauss Center for Supercomputing.
HPX is currently funded by the following grants:
- The National Science Foundation through awards 1240655 (STAR), 1339782 (STORM), and 1737785 (Phylanx). Any opinions, findings, and conclusions or recommendations expressed in this material are those of the author(s) and do not necessarily reflect the views of the National Science Foundation.
- The Department of Energy (DoE) through the awards DE-AC52-06NA25396 (FLeCSI) and DE-NA0003525 (Resilience). Neither the United States Government nor any agency thereof, nor any of their employees, makes any warranty, express or implied, or assumes any legal liability or responsibility for the accuracy, completeness, or usefulness of any information, apparatus, product, or process disclosed, or represents that its use would not infringe privately owned rights. Reference herein to any specific commercial product, process, or service by trade name, trademark, manufacturer, or otherwise does not necessarily constitute or imply its endorsement, recommendation, or favoring by the United States Government or any agency thereof. The views and opinions of authors expressed herein do not necessarily state or reflect those of the United States Government or any agency thereof.
- The Defense Technical Information Center (DTIC) under contract FA8075-14-D-0002/0007. Neither the United States Government nor any agency thereof, nor any of their employees makes any warranty, express or implied, or assumes any legal liability or responsibility for the accuracy, completeness, or usefulness of any information, apparatus, product, or process disclosed, or represents that its use would not infringe privately owned rights.
- The Bavarian Research Foundation (Bayerische Forschungsstfitung) through the grant AZ-987-11.
- The European Commission’s Horizon 2020 programme through the grant H2020-EU.1.2.2. 671603 (AllScale).